Understanding the Quantum of Energy in Modern Physics
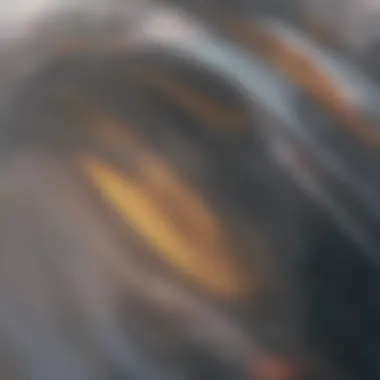
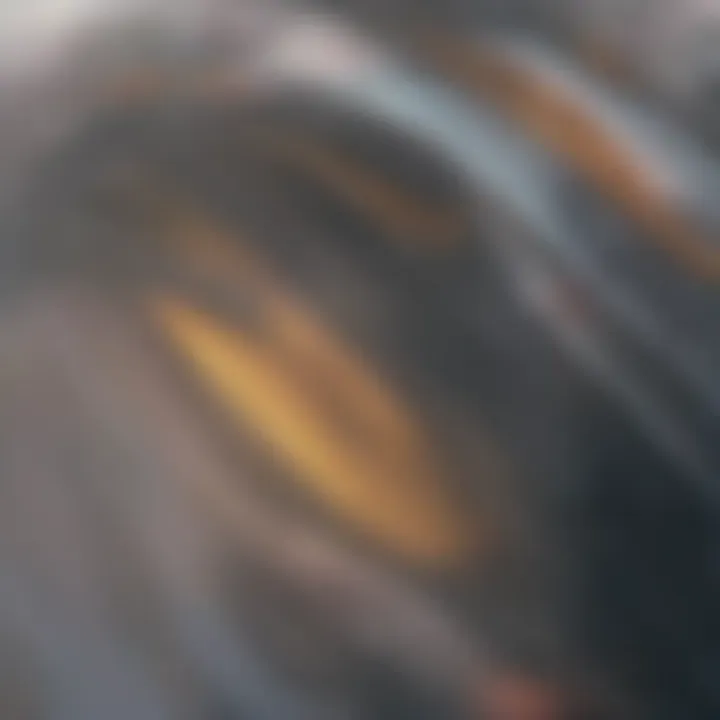
Intro
The quantum of energy is a concept that may appear abstract but is fundamentally crucial to our understanding of the universe. This article strives to unpack the layers of this concept. Understanding energy quantization can help clarify the behavior of matter at microscopic levels, providing insight into phenomena that govern not only physics but chemistry and even technology.
The developments in quantum theory have reshaped the scientific landscape. This guide will journey through essential concepts, key research findings, and implications of energy quantization. It aims to make these advanced ideas accessible to students, researchers, educators, and professionals alike.
Key Research Findings
Overview of Recent Discoveries
Recent discoveries related to the quantum of energy have further illuminated aspects that were once enigmatic. Researchers have explored energy quantization in numerous contexts, ranging from the behavior of electrons in atoms to the characteristics of light. One significant finding is the experimental validation of the quantized nature of energy levels in hydrogen, a fundamental element. Scientists used advanced techniques such as laser cooling and atomic spectroscopy to observe these transitions more clearly.
Another noteworthy advancement is the development of quantum computing. Here, the principles of energy quantization lead to faster processing and storage capabilities. Superposition and entanglement are phenomena that arise from these principles, raising questions about traditional paradigms in computation and information science.
Significance of Findings in the Field
These discoveries have profound implications. The acknowledgment of discrete energy levels aids in the understanding of atomic structures and chemical bonds. Moreover, the insights gained from quantum computing promise to revolutionize industries by optimizing complex problem solving.
"The implications of quantized energy levels extend beyond theoretical physics; they lead us to innovations that impact everyday technologies.โ
Breakdown of Complex Concepts
Simplification of Advanced Theories
To understand the quantum of energy, grasping associated theories is imperative. The concept of quantization challenges classical views of continuous energy levels. Instead, energy exists in discrete amounts known as quanta. This principle is observable in phenomena such as photoelectric effect, where light interacts with grains of matter, leading to emission of electrons.
Visual Aids and Infographics
Utilizing visual aids can significantly enhance comprehension. Diagrams illustrating the electron energy levels in an atom or the graphical representation of the photoelectric effect serve as practical tools. These visuals can make the complex nature of quantum mechanics more relatable.
In summary, the quantum of energy is not just an academic curiosity. Understanding it sheds light on fundamental scientific principles. Furthermore, it presents a wealth of opportunities for future research and technological advancement.
Defining Energy in Physics
Energy stands as a foundational concept in physics. It serves as a measuring stick for all physical interactions and phenomena we observe in the universe. Understanding energy is crucial because it influences every aspect of the material world. This section will elaborate on the nature of energy and how it is quantified, establishing a solid groundwork for discussing more intricate topics like the quantum of energy.
The significance of defining energy in physics extends beyond theoretical applications. It encompasses real-world implications across various fields such as chemistry, engineering, and environmental science. When one understands energy, they gain insights into natural processes, technological innovations, and resource management. Energy accounts for the ability to perform work or produce change within a system, thereby becoming a vital lens through which we can view interactions between matter.
The Fundamental Nature of Energy
The fundamental nature of energy hinges on its ability to exist in multiple forms while adhering to the law of conservation. Energy is neither created nor destroyed; it merely transforms from one form to another. This principle lays the groundwork for various phenomena we study in physics, from the motion of planets to chemical reactions.
Energy can manifest as potential energy, kinetic energy, thermal energy, and many other forms. Each type of energy has specific characteristics and equations that govern its behavior. For example, kinetic energy relates directly to the motion of an object, while potential energy depends on an object's position within a force field. By grasping the fundamental nature of energy, we can begin to appreciate its role in the universe more profoundly.
Types of Energy: A Brief Overview
Energy can be categorized into various types, each with unique properties and functions. Here are some of the main types of energy:
- Kinetic Energy: The energy an object possesses due to its motion. It is dependent on the mass of the object and its velocity, expressed through the equation:[ KE = \frac12 mv^2 ]
- Potential Energy: The energy stored within an object due to its position or configuration. Gravitational potential energy, for instance, is determined by an object's height and mass.
- Thermal Energy: The total energy of particles in a system, often related to temperature. It reflects how fast particles are moving and their interactions.
- Chemical Energy: Energy stored within the bonds of chemical compounds. This type is released or absorbed during chemical reactions.
- Nuclear Energy: Released during nuclear reactions, this energy comes from the nucleus of atoms and can be harnessed for power.
Understanding these energy types is essential. Each plays a crucial role in scientific exploration and technological advancements. By analyzing how energy behaves and changes, we can uncover the principles that govern both classical physics and the more complex realm of quantum mechanics.
The Concept of Quantum Mechanics
Historical Background
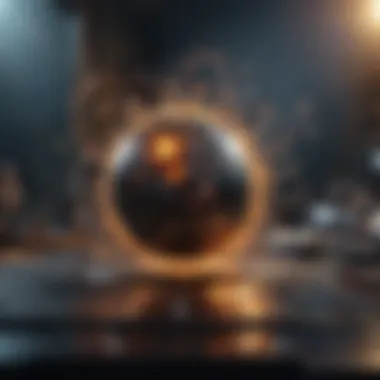
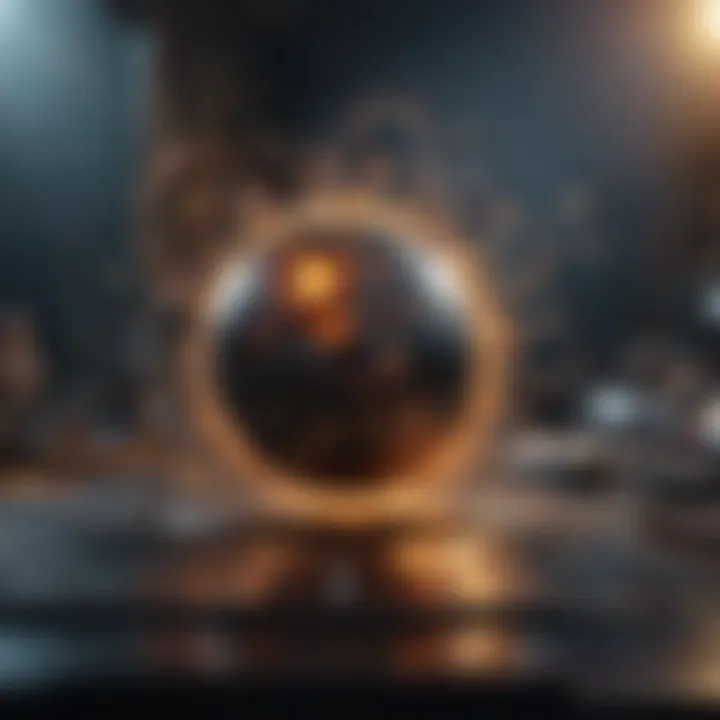
Quantum mechanics emerged in the early 20th century, driven by the inability of classical physics to explain certain experimental results. Notably, Max Planck introduced the concept of quantization in 1900, proposing that energy is emitted or absorbed in discrete units called quanta. This idea laid the groundwork for quantum theory.
In 1905, Albert Einstein expanded these ideas with his explanation of the photoelectric effect, demonstrating that light also exhibits particle-like behavior. By 1925, Werner Heisenberg developed matrix mechanics, and a few years later, Erwin Schrรถdinger presented wave mechanics, culminating in the formulation of the Schrรถdinger equation. These milestones solidified quantum mechanics as a robust framework for understanding atomic behavior.
Fundamental Principles of Quantum Mechanics
The principles of quantum mechanics are distinct and often counterintuitive. Key concepts include:
- Wave-Particle Duality: Particles exhibit both wave-like and particle-like properties depending on how they are observed.
- Uncertainty Principle: Heisenberg's principle states that certain pairs of properties, like position and momentum, cannot be simultaneously measured with absolute precision.
- Superposition: A particle can exist in multiple states at once until measured, leading to various possible outcomes.
- Entanglement: Particles can become correlated in such a way that the state of one instantly influences the state of another, regardless of distance.
The implications of these principles are far-reaching. They challenge classical notions of reality and foster a deeper understanding of the universe. They also form the theoretical basis for advanced technologies such as quantum computing and quantum cryptography, which leverage these unique properties for practical applications.
Quantum of Energy Defined
The concept of quantum of energy is essential in understanding how energy operates at microscopic scales. In classical physics, energy seems continuous, but quantum physics reveals that it is actually quantized. This means that energy is absorbed or emitted in discrete quantities called quanta. Understanding energy quantization aids in explaining phenomena like atomic spectra and the behavior of particles in quantum mechanics.
A few fundamental elements establish the foundation of quantum energy:
- The discrete nature of energy levels in atoms.
- The implications of energy quantization for particle behavior.
- The central role of Planck's constant in measuring energy.
These elements shape not only theoretical discussions in physics but also practical applications in technology and other sciences. Recognizing the specifics of how energy can only exist in certain values reshapes our understanding of the universe.
"In quantum mechanics, energy levels are quantized, radically changing the laws by which matter behaves on atomic and subatomic scales."
Planck's Constant and Its Role
Planck's constant is a fundamental quantity that provides the scale at which quantum effects become significant. Denoted by the symbol ( h ), this constant has a value of approximately ( 6.62607015 imes 10^-34 ) mยฒ kg / s. It connects the energy of a photon to the frequency of its associated electromagnetic waves, establishing:
[ E = h \cdot f ]
where ( E ) is energy and ( f ) is frequency. This relationship indicates that the energy of a photon is directly proportional to its frequency, implying that higher-frequency waves carry more energy than lower-frequency ones.
Understanding Planckโs constant is crucial for explaining various quantum phenomena, such as:
- The photoelectric effect, which supports the quantization of light.
- Blackbody radiation, leading to the development of quantum theory.
The implications of Planck's constant extend beyond physics into fields like chemistry and technology, making it indispensable in exploring the quantum realm.
Formula for Energy Quantization
The formula for energy quantization further elucidates how energy is restricted to discrete values, particularly within atomic systems. The energy levels of an electron in an atom can be expressed using the equation:
[ E_n = -\fracZ^2 \cdot k \cdot e^42 \cdot n^2 ]
where:\n- ( E_n ) is the energy level of the electron,
- ( Z ) is the atomic number,
- ( k ) is Coulomb's constant,
- ( e ) is the electron charge,
- ( n ) is the principal quantum number.
This equation indicates that energy levels are inversely related to the square of the principal quantum number ( n ). As ( n ) increases, the energy levels become less negative, which corresponds to higher potential energy states for the electron. Notably, transitions between these energy levels result in the emission or absorption of quanta of energy, such as photons. Thus, this formula is central to understanding atomic structure and electron transitions.
In summary, the exploration of the quantum of energy reveals intricate patterns underlying atomic and subatomic behavior. As we delve into the broader implications, we see how this concept shapes modern scientific inquiry.
Implications of Energy Quantization
The implications of energy quantization are profound, influencing both theoretical physics and practical applications. In a world where classical physics once dominated, the quantized nature of energy introduces a level of complexity that challenges our understanding. Energy quantization helps explain phenomena that classical theories cannot, such as the behavior of electrons in an atom or the properties of semiconductors. It is essential for explaining how matter interacts at the atomic and subatomic levels.
Quantum States and Energy Levels
In quantum mechanics, systems are described by their quantum states. These states represent specific energy levels that particles can occupy. Unlike classical mechanics, where energy can vary continuously, energy levels in quantum systems are discrete. An electron in an atom might inhabit one of several distinct energy levels. When energy is absorbed or emitted, it corresponds to a transition between these states. This quantization leads to several key effects, such as emission and absorption spectra unique to each element.
- Energy Level Transition:
- Stability of Atoms:
- Chemical Properties:
- When an electron moves between energy levels, it absorbs or releases energy. This process creates unique spectral lines.
- The wider the energy gap, the higher the energy of the photon emitted or absorbed.

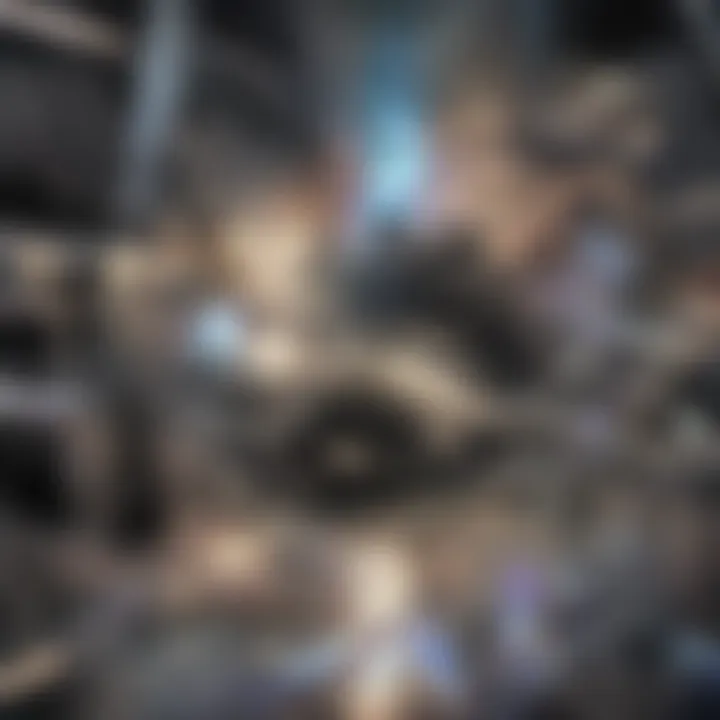
- Electrons in lower energy states are more stable than those in higher ones. Hence, atoms are less likely to participate in reactions unless energy is provided.
- The arrangement of electrons in energy levels contributes significantly to the properties of an element, influencing its reactivity and bonding behavior.
Discrete vs Continuous Energy Spectra
The concepts of discrete and continuous energy spectra illustrate a critical distinction in quantum mechanics. Discrete energy spectra are evident when examining quantum systems like atoms, where particles have specific energy states. Continuous spectra, on the other hand, occur in systems where particles are not restricted to fixed energy levels, allowing for a range of possible energies.
- Discrete Energy Spectra:
- Continuous Energy Spectra:
- Observed in atoms and molecules.
- Characterized by distinct separation between energy levels, leading to specific emission or absorption lines.
- Relevant for understanding atomic and molecular transitions.
- Found in classical systems or certain types of gases.
- Energy can vary without discrete jumps, leading to a continuum of energy values.
- Important in fields like thermodynamics, where systems can assume a range of energies in equilibrium.
"The beauty of quantum mechanics lies in its ability to describe the fundamentally distinct behaviors of particles, where quantization plays a key role."
Understanding the implications of energy quantization deepens our insight into the natural world, impacting various domains from solid-state physics to chemistry and even cosmology. Embracing both discrete and continuous spectra enables scientists to model complex systems, paving the way for innovative applications in technology and theoretical exploration.
Applications of Quantum Energy Concepts
The applications of quantum energy concepts extend across various fields, showcasing a transformative impact that drives innovation and deeper understanding of nature. These concepts not only influence theoretical aspects within physics but also manifest in practical technologies and scientific methodologies. By exploring such applications, we can appreciate how quantum mechanics shapes our modern world and opens new avenues for research and development.
In Technologies: Quantum Computing and Cryptography
Quantum computing represents a paradigm shift in information processing. In classical computing, data is processed in bits that are either 0 or 1. Quantum computing, however, uses quantum bits, or qubits. A qubit can exist in multiple states simultaneously, thanks to the principle of superposition. This enables quantum computers to perform complex calculations at unprecedented speeds. Important algorithms, such as Shor's algorithm, can factor large numbers efficiently, posing a significant challenge to current cryptographic systems that rely on the difficulty of this task.
In cryptography, the principles of quantum mechanics are exploited for secure communications through quantum key distribution (QKD). QKD relies on the behavior of quantum systems. For example, any attempt to eavesdrop on a quantum key transmission alters the states of the quantum particles, making the intrusion detectable. Protocols like BB84 are notable examples of QKD that ensure a high level of security, paving the way for future secure communications.
"Quantum computing and cryptography will revolutionize industries by enhancing security and computational power, reshaping the landscape of technology as we know it."
In Chemistry: Molecular and Atomic Reactions
The role of quantum mechanics is equally vital in chemistry, particularly in understanding molecular and atomic reactions. Quantum energy concepts help to explain how atoms interact, the formation of chemical bonds, and the transitions between different states of matter. For instance, the concepts of wave functions and energy levels are essential in determining molecular structures and reaction pathways. Atomic orbitals, as defined by quantum mechanics, dictate the electron arrangements that govern chemical properties.
Additionally, phenomena like electron tunneling, where particles pass through potential energy barriers, are explained through quantum principles. This understanding allows chemists to predict reaction rates and mechanisms more accurately, which is fundamental for drug design and materials science. Further, spectroscopic techniques, such as nuclear magnetic resonance (NMR) and infrared spectroscopy, rely on quantum behaviors to elucidate molecular characteristics, opening doors for significant advancements in both academic and industrial settings.
The exploration and applications of quantum energy concepts thus lay the groundwork for monumental shifts in both technology and scientific understanding, indicating how crucial these principles are to advancing multiple disciplines.
Ongoing Research in Quantum Energy
The field of quantum energy is rapidly transforming as new discoveries emerge. Research in this area is essential for advancing our understanding of the physical world. It addresses fundamental questions and has applications across multiple disciplines such as physics, chemistry, and technology. Ongoing studies not only reveal deeper insights into the quantum realm but also foster innovation and applications that could reshape industries.
Recent Advances in Quantum Theory
Recent advancements in quantum theory have profound implications. These include the development of quantum field theories, which extend traditional quantum mechanics. Researchers are now focusing on integrating various frameworks, such as string theory and loop quantum gravity. These efforts aim at creating a more unified theory that could explain fundamental forces and particles.
For instance, scientists are investigating the nature of dark matter and dark energy through quantum approaches. This research aims to solve some of the most pressing cosmological questions. Moreover, developments in quantum entanglement and superposition have potential applications in quantum computing. Quantum entangled states can lead to significant speed-ups for certain computational problems, making this a hot topic in both theoretical and applied research.
Experimental Approaches to Energy Quantization
Experimental research plays a crucial role in testing and validating quantum theories. Researchers employ various methodologies to study energy quantization and its effects. Techniques such as laser cooling and trapping have allowed scientists to observe quantum states in a more controlled environment. Experiments using Bose-Einstein condensates provide insights into the behavior of particles at incredibly low temperatures, offering unique perspectives on energy quantization.
Additionally, advancements in nanotechnology have enabled the construction of precise quantum devices. Quantum dots and superconducting qubits are being used for experimental verification of quantum theories. These technologies can isolate and manipulate quantum states, making them invaluable in research.
"The essence of ongoing research in quantum energy lies in its capacity to challenge existing paradigms and foster a deeper understanding of nature's fundamental mechanisms."
In summary, the ongoing research in quantum energy is not merely an academic pursuit. It has significant implications for our understanding of the universe and practical applications that could revolutionize technology. By pushing the boundaries of what we know, researchers contribute to a body of knowledge that is essential for future innovations.
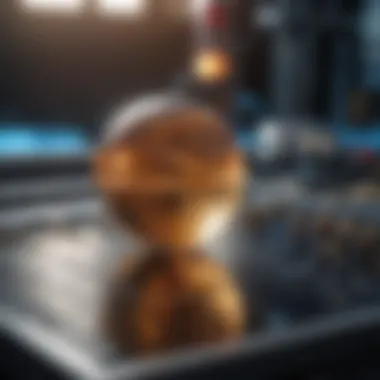
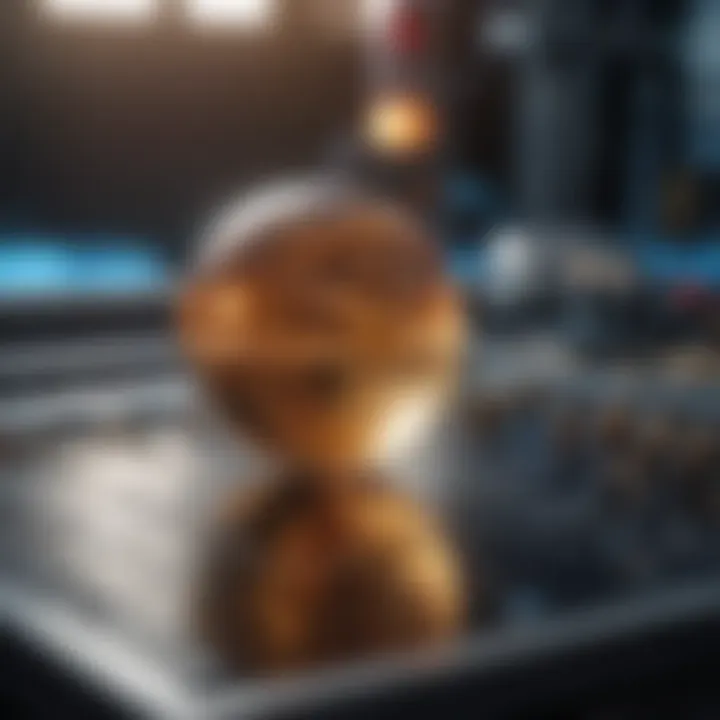
Philosophical Considerations
The exploration of philosophical considerations surrounding quantum energy offers profound implications. It prompts us to question the very foundations of our understanding of reality. With energy quantization and its discrete nature at play, the relationship between the observer and the observed becomes a central theme. This challenges traditional notions of determinism and the continuous nature of physical reality.
The Nature of Reality: Quantum vs Classical Views
In classical physics, reality adheres to principles of determinism and continuity. Objects possess definite states, defined by precise properties such as position and momentum. Conversely, quantum mechanics introduces a different paradigm. Reality is not fixed but probabilistic. The precise state of a particle cannot be determined until it is measured, leading to the idea that until observed, particles exist in a superposition of states. This fundamental shift highlights contradictions between our intuitive ideas and the actual behavior of the quantum world.
The implications ripple across various fields. Philosophy now encompasses themes from epistemology to metaphysics, delving into the boundaries between knowledge and reality. When particles can exist in multiple states simultaneously, it raises questions about the nature of existence itself. Are particles real when not observed? How does consciousness impact reality? The dialogue continues, examining the impact of quantum phenomena on philosophical frameworks.
Impact on Understanding the Universe
The interplay between quantum mechanics and our understanding of the universe can reshape scientific paradigms. By accepting that energy quantization influences the structure of space and time, we expand our understanding beyond classical mechanics. This leads to possibilities for unifying various theories in physics, like quantum gravity, challenging established beliefs.
Moreover, the philosophical implications extend to existential inquiries. If the universe is inherently probabilistic, how does this affect free will? Does it diminish human agency or empower it? The debate remains active, echoing through academic discussions and practical considerations.
Overall, engaging with these philosophical aspects invites deeper contemplation on the implications of quantum mechanics. It encourages scientists and thinkers alike to reevaluate not only the nature of energy but also fundamental perceptions of existence and reality.
"Understanding quantum mechanics is more than just a scientific pursuit; it shapes our very conception of the universe and our place within it."
Inviting interdisciplinary collaboration between philosophy and science allows us to examine these critical questions rigorously. As researchers investigate the depths of quantum energy, the conversations will undoubtedly continue, shaping future discoveries.
Future Directions in Energy Quantum Research
As the field of quantum energy continues to evolve, it is crucial to consider the future directions in research regarding energy quantization. The ongoing advancements promise not only to deepen our understanding of fundamental physics but also to introduce innovative applications across various sectors. Researchers are actively investigating new theoretical frameworks, experimental techniques, and potential practical implications of quantum energy. This area of study could unlock new avenues in technology, environmental preservation, and our grasp of universal laws.
Potential Developments and Innovations
In upcoming years, we may witness significant developments in quantum energy research. Some promising innovations include:
- Quantum Energy Storage: Improving energy storage methods with quantum capabilities. This could allow for more efficient batteries, leveraging principles of energy quantization to enhance performance and longevity.
- Quantum Communication Systems: Advancements in secure communication could spring from quantum encryption techniques. Such systems rely on the principles of quantum superposition and entanglement, making data transmission more secure.
- Photonic Devices: Developing devices that manipulate light at the quantum level for use in various applications, including computing and telecommunications.
Moreover, the collaboration between academic institutions and industries will be vital in translating theoretical concepts into practical applications.
Interdisciplinary Approaches and Collaborations
Addressing the complexities of quantum energy research demands interdisciplinary approaches. Merging insights from fields such as physics, engineering, and material science can foster comprehensive strategies for tackling challenges in energy quantization. Here are a few considerations:
- Collaboration Across Disciplines: Researchers from different scientific backgrounds can work together to share knowledge and techniques. This will lead to more holistic research outcomes and encourage innovation.
- Public-Private Partnerships: Engagement between universities and industry can facilitate the transition of research from laboratories to real-world applications.
- Global Research Initiatives: Coordinating international research efforts can enable pooling resources, funding, and expertise. Such initiatives would enhance collaboration on a larger scale, addressing global energy challenges.
Future directions in quantum energy research are not just about advancing science. They have profound implications for technology and society.
Ending
The conclusion serves as a pivotal section of this article, providing a lucid synthesis of the essential elements discussed in prior sections. By summarizing key insights into the quantum of energy, it reinforces the conceptual understanding of how this principle shapes modern physics.
The importance of this connection cannot be understated. The exploration of energy quantization clarifies how energy exists in discrete packets, fundamentally differing from classical perspectives. This nuanced understanding facilitates deeper insights into a range of phenomena, from atomic interactions to advanced technologies. Thus, an effective conclusion encapsulates not just what has been learned, but why it matters.
Summarizing Key Insights
In reviewing the core ideas presented throughout the article, several points emerge as particularly significant:
- Energy is Quantized: Unlike classical theories, energy at the quantum level is not continuous but rather exists in defined quantities.
- Planck's Constant: Serving as a cornerstone in quantum mechanics, this constant signifies the smallest unit of energy, crucial for understanding phenomena on atomic scales.
- Applications Beyond Physics: The principles of energy quantization influence various fields, including technology, chemistry, and philosophy, highlighting the interconnectedness of scientific inquiry.
These insights underscore the importance of a clear grasp of quantum energy concepts for various audiences, extending far beyond theoretical physics.
The Ongoing Importance of Energy Quantization
Energy quantization continues to play a transformative role in both theoretical and applied sciences. As modern research progresses, its relevance is affirmed by:
- Technological Innovations: Quantum computing and cryptography are beginning to manifest the practical applications of energy quantization, driving advancements that could reshape industries.
- Chemical Reactions: Understanding energy levels aids in deciphering molecular interactions, which is fundamental to advancements in materials science and pharmaceutical development.
- Philosophical Implications: The reconsideration of reality at a fundamental level encourages a new dialogue about the nature of existence and interconnectedness in the universe.
Thus, the significance of energy quantization is not confined to physics alone; it heralds a future where interdisciplinary collaborations are essential for understanding complex systems in science, technology, and beyond.