Recent Breakthroughs in Quantum Physics Explained
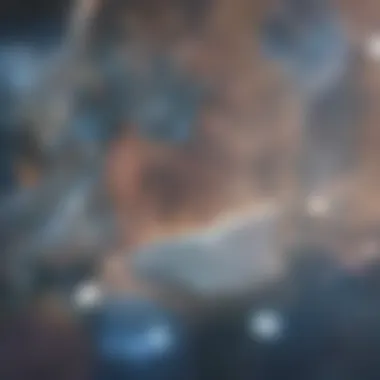
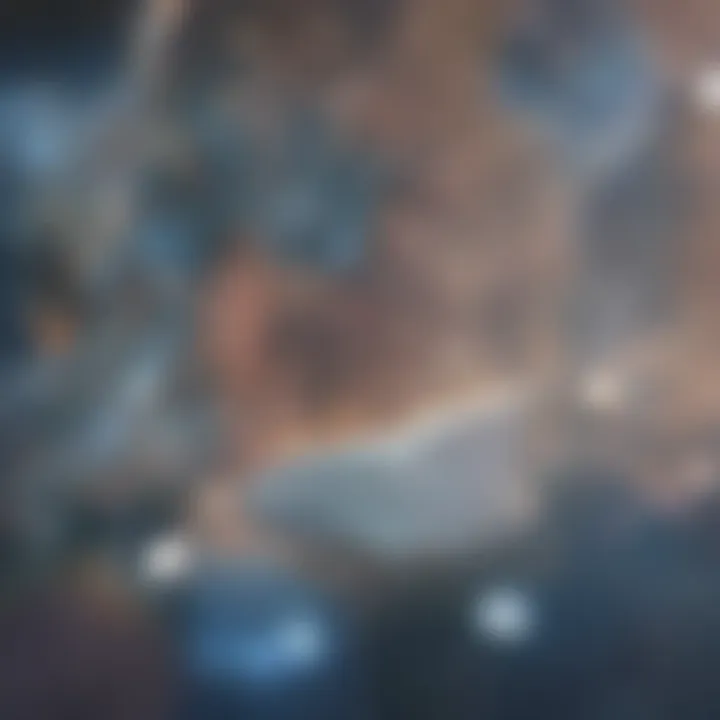
Intro
Quantum physics is a rapidly evolving field that continuously reshapes our understanding of the physical universe. Recent advancements drive both theoretical and experimental research forward, offering fresh insights into the behavior of matter and energy at the quantum level. The latest developments have implications that reach far beyond academia, influencing emerging technologies, communication systems, and even our fundamental comprehension of reality itself.
Key Research Findings
Overview of Recent Discoveries
In the past few years, several breakthroughs have captivated the attention of researchers and enthusiasts alike. Notably, the advancements in quantum entanglement and quantum computing stand out. Researchers are now able to create more stable qubits, which enhance the reliability of quantum computers. The work by companies like IBM, particularly their development of the Quantum Hummingbird architecture, illustrates this progress. They aim to improve error correction methods and increase the number of qubits available for computation.
Furthermore, recent experiments by scientists at the University of Science and Technology of China demonstrated quantum entanglement across unprecedented distances, further proving the feasibility of quantum communication.
Significance of Findings in the Field
These findings are not merely academic; they hold profound significance for various sectors. Better quantum computers can solve problems currently intractable for classical computers. This will impact fields ranging from cryptography to drug discovery and artificial intelligence. The ability to maintain entangled states over longer distances may revolutionize telecommunications and create secure channels for data transmission.
"Quantum physics not only challenges our conventional understanding of reality but also propels advancements across diverse industries."
Breakdown of Complex Concepts
Simplification of Advanced Theories
Quantum physics is inherently complicated, often requiring nuanced comprehension for even seasoned physicists. To make this accessible, concepts such as superposition and entanglement need clear explanations. Superposition allows particles to exist in multiple states at once until measured. This concept underpins the working principles of quantum computers.
Entanglement, another key concept, describes a situation wherein particles become interconnected such that the state of one instantaneously affects the state of another, no matter the distance separating them. This phenomenon has sparked new research avenues in quantum networks.
Visual Aids and Infographics
Using visual aids can aid in digesting these advanced theories. For example, an infographic illustrating the interaction between entangled particles may provide a clearer understanding than text alone.
Some helpful resources for more information include:
Understanding these concepts is crucial as they form the backbone of recent advancements in the field. As quantum physics continues to revolutionize our technological landscape, staying informed about these topics becomes imperative.
Prologue to Quantum Physics
Quantum physics represents a pivotal domain of modern scientific inquiry. Its principles underpin the fundamental behavior of matter and energy at the microscopic scale. As technology advances, understanding these principles has become increasingly important, establishing quantum physics as a cornerstone not only of physics but also of technology and philosophy. This section delves into critical elements of quantum physics, its significance, and its far-reaching implications.
Fundamental Concepts
At the core of quantum physics lies a set of concepts that challenge classical interpretations of the universe. These include wave-particle duality, superposition, and entanglement.
- Wave-Particle Duality: This principle asserts that particles, such as electrons and photons, exhibit both wave-like and particle-like properties. This duality shifts our understanding of how light and matter should be perceived.
- Superposition: Quantum systems can exist in multiple states at once until measured, fundamentally altering classical ideas of object states. This leads to phenomena such as interference and uncertainty.
- Entanglement: When particles become entangled, the state of one particle becomes dependent on the state of another, regardless of the distance separating them. This challenges traditional notions of locality and connectivity.
These fundamental concepts not only reshape scientific thought but also form the foundation for many quantum technologies emerging today.
Historical Context
The evolution of quantum physics has its roots dating back to the early 20th century. Pioneers such as Max Planck and Albert Einstein were instrumental in laying the groundwork for this field. Planck's introduction of the concept of quantization in 1900 marked a significant turning point. His work laid the foundation for understanding thermal radiation.
In 1915, Einsteinβs explanation of the photoelectric effect reinforced the notion that light has particle characteristics, thereby contributing to establishing quantum theory. Fast forward a few decades, and the development of quantum mechanics, formulated by figures like Niels Bohr and Werner Heisenberg in the 1920s, revolutionized physics by introducing new frameworks to discern atomic and subatomic behavior.
As quantum theory matured, it fueled advancements in various scientific domains, giving rise to applications in chemistry, material sciences, and emergent technologies. The historical context illuminates how quantum physics evolved from an abstract theory into an essential pillar of scientific and technological progress. In summary, understanding quantum physics through its fundamental concepts and historical evolution is vital, not just to grasp scientific phenomena but also to appreciate its influence on contemporary technology and future developments.
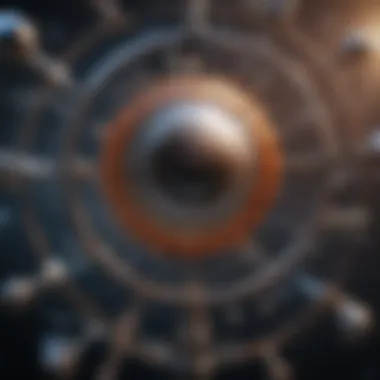
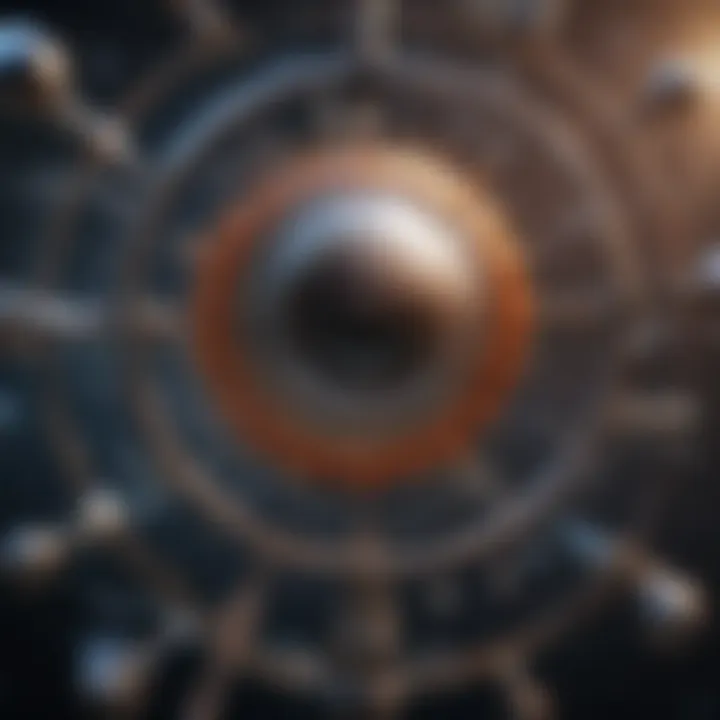
Recent Breakthroughs in Quantum Research
Recent breakthroughs in quantum research represent a transformative phase in the realm of physics and technology. This focus on advancements highlights crucial developments with far-reaching implications across various fields. As we delve into the specifics, it becomes clear that these breakthroughs are not merely academic but directly influence the practicalities of industries, from computing to telecommunications.
Advancements in Quantum Computing
Current state of quantum processors
The current state of quantum processors is marked by significant progress. Companies like Google, IBM, and Rigetti Computing have developed processors that utilize qubits instead of classical bits. Qubits can represent and process more information due to their ability to exist in multiple states simultaneously. This characteristic is fundamental to enhancing computational power. The trend toward larger and more stable quantum processors suggests a future where complex problems can be solved more efficiently. However, challenges such as error rates and operational temperatures need addressing.
Key players in the industry
In the quantum computing landscape, key players include Google, IBM, Microsoft, and D-Wave Systems. Each organization contributes uniquely. For instance, Google's quantum supremacy claim in 2019 marked a pivotal point in the field. IBM's development of the Qiskit framework encourages collaboration through open-source practices. Each player's distinct approach fosters innovation while also presenting challenges. The competitive nature of these companies drives rapid advancements but may also lead to fragmentation in technology standards.
Quantum Entanglement Studies
New experiments and findings
New experiments in quantum entanglement have expanded our understanding of quantum mechanics. Recent studies reveal how entangled particles can affect each other's states, no matter the distance. This phenomenon supports theories that challenge classical intuitions about locality and causality. The findings not only validate long-standing hypotheses but also inspire new methods of quantum information transfer. As experimental techniques improve, our grasp of the underlying processes continues to evolve. However, the implications of these studies also spur ethical discussions about the potential misuse of such technologies.
Applications in communication
Applications arising from quantum entanglement in communication offer promising avenues. Quantum key distribution ensures secure communication by enabling two parties to share encryption keys that are fundamentally secure from eavesdropping. This capability provides a stark contrast to classical encryption methods. Moreover, advancements in quantum communication protocols can lead to more robust networks. Yet, the integration into existing infrastructures poses significant challenges that require further research and collaboration across disciplines.
"Quantum communication has the potential to revolutionize how we share information securely, unbridling the constraints of classical methods."
The exploration of recent breakthroughs in quantum research not only provides insight into emerging technologies but also positions us at the frontline of understanding the universe. As these developments unfold, it is critical to navigate both the opportunities and challenges they present.
Quantum Information Theory Innovations
Quantum Information Theory has evolved significantly in recent years. This section sheds light on how its innovations are reshaping our understanding of information processing and security. Quantum Information Theory serves as the backbone for many advancements, particularly in computing and cryptography. The impact of these innovations can be seen in various sectors, from telecommunications to data science.
Development of Quantum Algorithms
Quantum algorithms have shown remarkable capabilities. They harness the principles of quantum mechanics to perform calculations at unprecedented speeds, solving problems that classical computers would take impractically long to address.
Shor's and Grover's Algorithms
Shor's algorithm revolutionized the field of cryptography. It enables the factoring of large integers efficiently, posing a significant threat to traditional encryption methods. Grover's algorithm, on the other hand, offers a way to speed up searches in unsorted databases. Both algorithms are considered foundational in providing a glimpse into the potential of quantum computing.
The key characteristic of these algorithms lies in their efficiency. For instance, Shor's algorithmβs speed in finding prime factors could render current encryption methods obsolete. This distinctive feature suggests a beneficial potential in the context of cybersecurity, making a compelling case for further research in quantum algorithms despite their complexity.
Recent implementations of these algorithms have made significant strides. Improved error correction has enabled them to function better on existing quantum hardware. The unique advantage of these advancements is the possibility of reaching practical applications sooner, which could catalyze quantum technology adoption across industries.
Recent Improvements
Recent improvements in algorithms focus on optimizing performance and reliability. Enhanced error resilience is a major aspect of these developments, ensuring that quantum calculations maintain accuracy. Several research teams have produced methods that increase the threshold for noise tolerance in quantum systems.
The key characteristic of these improvements is their focus on real-world applicability. Researchers are primarily concerned with making quantum algorithms more usable in practical scenarios, which is a significant step forward for the field.
This unique emphasis on practicality makes the improvements particularly relevant to the industry. However, challenges remain, such as developing suitable hardware to implement these sophisticated algorithms effectively.
Cryptography in Quantum Context
The intersection of quantum mechanics and cryptography is an area of intense research interest. Quantum cryptography promises to radically enhance data security by utilizing the principles of quantum physics.
Quantum Key Distribution
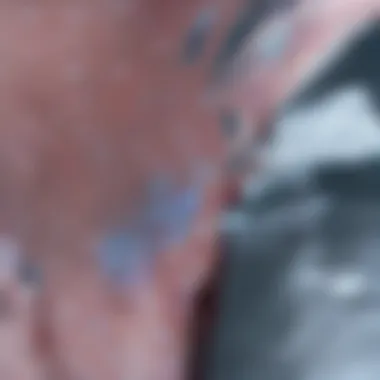
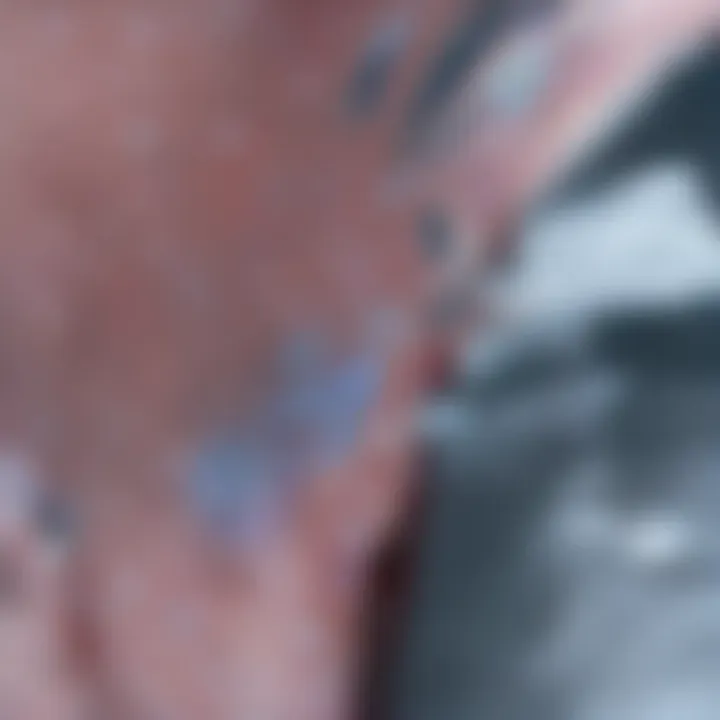
Quantum Key Distribution (QKD) stands out as a key method in quantum cryptography. It allows two parties to generate a shared, secret key for encrypted communication, protected by the laws of quantum physics. The unique feature of QKD is its theoretical security; any attempt to intercept the key will disturb the quantum state, alerting the parties involved.
This characteristic makes QKD an attractive solution for secure communications in sensitive domains. However, its deployment faces challenges, including technical limitations and the need for a robust infrastructure.
Implications for Security
The implications for security are profound. As quantum technology develops, the need to revisit existing security measures becomes urgent. Classical encryption methods may no longer be safe, emphasizing the importance of transitioning to quantum-resistant algorithms.
The key aspect of this shift is the potential for enhanced security protocols that quantum technologies offer. This transition can protect sensitive information in an increasingly digital world. Nonetheless, the threat of quantum computing also brings about disadvantages, as vulnerabilities in current systems could be exploited before quantum-safe solutions are thoroughly developed.
"Quantum cryptography offers a new paradigm in ensuring data security, but the technological landscape is rapidly evolving, necessitating timely adaptations in our defense strategies."
The ongoing advancements in quantum information theory underscore the need for critical awareness among professionals and researchers alike. Understanding these developments is essential to harness their full potential, ensuring a secure and efficient future.
Experimental Techniques in Quantum Physics
Experimental techniques play a vital role in advancing quantum physics. They allow researchers to test theories, validate hypotheses, and explore phenomena that define the quantum realm. By employing precise measurement methods and sophisticated tools, scientists can not only investigate fundamental principles but also push the boundaries of application in various fields. This section delves into the innovations in measuring techniques and the role of quantum simulators in current research, highlighting their significance in the continued evolution of this field.
Innovations in Measurement Techniques
Precision measurement advancements
Precision measurement advancements are crucial in quantum experiments. These techniques focus on achieving accuracy beyond what classical methods can provide. A key characteristic of these advancements is their ability to minimize errors, which is essential when observing quantum states. These high-level measurements can capture minute changes, revealing insights that were previously undetectable. This focus on precision makes advancements extremely beneficial for this article, as it underpins various research efforts in quantum physics.
One unique feature of precision measurement advancements is their reliance on interferometry. This method uses the wave nature of quantum systems to detect extremely small changes in phase. This approach opens doors to many applications, including gravitational wave detection and fundamental constant measurement. However, the complexity involved in conducting these measurements can be a disadvantage, as it requires highly controlled environments. Despite this, the insights gained are often worth the effort involved.
Tools and technologies
The tools and technologies employed in quantum physics research have evolved remarkably. These innovations include advanced lasers, superconducting materials, and high-speed electronics, which enable researchers to manipulate quantum systems with great precision. The key characteristic of these tools is their ability to produce consistent results under varying conditions. This makes them popular choices for the experiments discussed in this article.
A unique aspect of these technologies is the integration of various disciplines such as engineering and computer science. These fields contribute significantly to the development of quantum technologies, enhancing their performance and applicability. However, one challenge faced is the high level of expertise required to operate sophisticated equipment. This might limit accessibility for some researchers. Nevertheless, the potential benefits they provide in exploring quantum phenomena far outweigh these concerns.
Role of Quantum Simulators
Quantum simulators are becoming increasingly essential in understanding complex quantum systems. They allow researchers to mimic the behavior of quantum particles using controllable systems. This role is crucial in predicting outcomes that would otherwise be impossible or extremely difficult to calculate using classical methods. With quantum simulators, scientists can explore interactions within quantum materials and gain deeper insights into phenomena like superfluidity or quantum phase transitions.
One significant contribution of quantum simulators is their ability to model systems with many-body interactions. This complexity often presents significant challenges when analyzed through traditional computational methods. By creating controlled environments, researchers can simulate various conditions and study their effects on quantum behavior. While these simulators hold great promise, one consideration is the accuracy of the models employed. If the simulator cannot accurately replicate the system being studied, the results may lead to misleading conclusions.
In summary, the advancements in experimental techniques and the introduction of quantum simulators are pivotal in currently advancing quantum physics. They are essential tools for researchers aiming to decode the complexities of the quantum world. As technology continues to evolve, the potential for groundbreaking discoveries remains vast.
Interdisciplinary Applications of Quantum Physics
The field of quantum physics is rapidly evolving and intersecting with various disciplines, leading to remarkable applications. Understanding the interplay between quantum theories and practical implementations provides valuable insights. This section outlines how quantum physics is influencing diverse fields and highlights specific contributions in material science and medicine.
Impact on Material Science
Quantum materials research
Quantum materials research focuses on materials that exhibit quantum mechanical effects. These materials show unique properties such as superconductivity and magnetoresistance, which are essential for developing advanced technologies. For instance, materials like graphene and topological insulators present significant potential due to their unique electronic properties.
A key characteristic of quantum materials research is its emphasis on nanoscale phenomena, where quantum effects dominate. This aspect makes it a popular choice for exploring new technological frontiers in materials science. Researchers aim to manipulate these properties for applications ranging from quantum computing to energy storage.
Nevertheless, this field does face challenges. Understanding the underlying physics can be complex, and the fabrication of quantum materials in a controllable manner requires advanced techniques and equipment. However, the advantages of discovering and harnessing new materials are substantial. They have the potential to revolutionize industries and enhance our technological capabilities.
Potential for new technologies
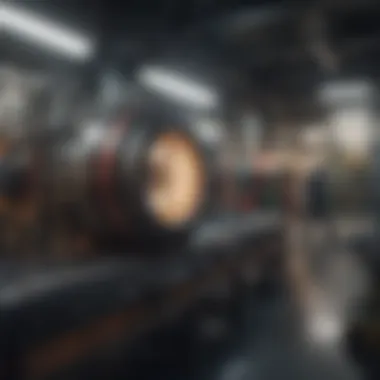
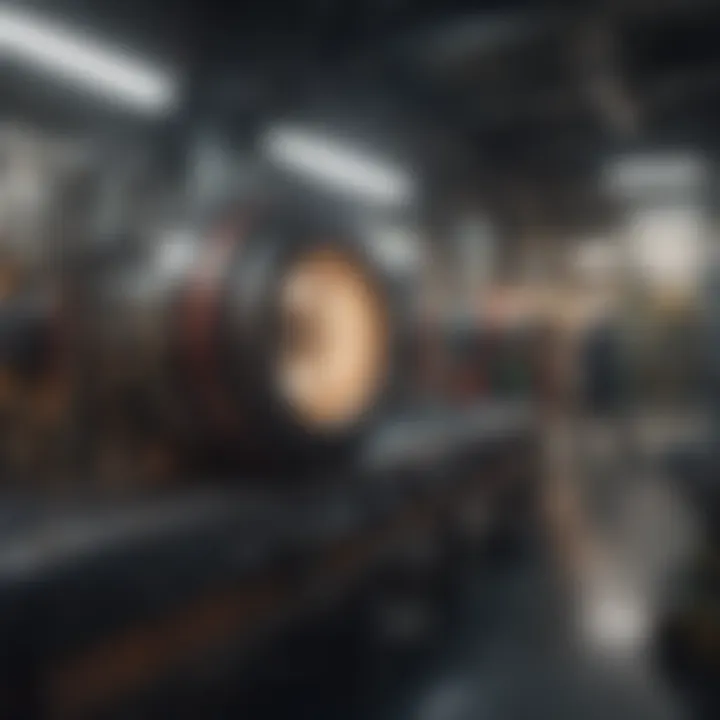
Quantum materials hold promise for a new wave of technologies that can change existing systems. The potential spans across various sectors, including energy, electronics, and communication. For example, the use of quantum dots in solar cells has been identified as a method to increase efficiency compared to traditional materials.
A defining feature of this potential is its integration into existing technology infrastructures. This aspect makes it beneficial for addressing real-world problems while also pushing the boundaries of innovation. However, scaling these technologies for mass production remains a significant hurdle.
The unique feature of potential new technologies is their capability to enhance operational efficiencies and capabilities. While the advantages include increased performance and sustainability, there are disadvantages related to cost and the need for specialized knowledge for implementation. The research continues to expand in this area, providing a promising landscape for future advancements in various technologies.
Applications in Medicine
Quantum imaging techniques
In medicine, quantum imaging techniques allow for high-resolution imaging, crucial for accurate diagnostics. By utilizing principles from quantum mechanics, these methods enhance the clarity of images obtained from scans, enabling better identification of diseases.
The key characteristic of quantum imaging is its ability to capture images with minimal radiation exposure. This aspect adds to its attractiveness as a safer alternative to traditional imaging techniques, such as X-rays. The unique feature of this technique is its reliance on entangled photons, providing advantages in sensitivity and resolution. However, the complexity of quantum imaging systems can pose challenges in practical adoption.
Insights into biological systems
Quantum physics also offers unique insights into biological systems via quantum biology, studying the influence of quantum processes on biological phenomena. This intersection provides enhancement in our understanding of complex biological functions, like photosynthesis and enzyme efficiency.
A critical characteristic of insights into biological systems is that it encourages a deeper investigation into how quantum effects influence life at the molecular level. As a subject of growing interest, its inclusion in this article reflects its importance in facilitating novel therapeutic strategies. However, navigating this frontier requires an interdisciplinary approach, drawing knowledge from both quantum physics and biology to unlock new possibilities.
The Future of Quantum Physics
The future of quantum physics is a domain of both excitement and speculation. It holds the potential to redefine our understanding of the universe and our place within it. This section delves into predictions for quantum technology and the philosophical implications that arise from the advancements in this field.
Predictions for Quantum Technology
Possible breakthroughs
The trajectory of quantum technology in the coming years can lead to several significant breakthroughs. One highly anticipated advancement is the enhancement of quantum computing. Unlike traditional computers, which perform calculations using bits, quantum computers use qubits. This allows them to process vast amounts of data simultaneously. The transformation in computational power could revolutionize sectors like cryptography, materials science, and artificial intelligence.
A key characteristic of these possible breakthroughs is their potential to solve problems beyond the reach of classical supercomputers. For instance, drug discovery could benefit immensely due to the ability to simulate molecular interactions at unprecedented speeds. This is a beneficial aspect of quantum technology, making it an attractive area for investment and research.
However, the challenges presented by such powerful technology cannot be overlooked. The unique feature of quantum computing is its inherent complexity. While it offers substantial advantages in speed and efficiency, it also requires specific conditions, such as extremely low temperatures, to function effectively.
Challenges to overcome
Despite the potential breakthroughs, several challenges remain in the field of quantum physics. One major challenge is developing stable qubits. Currently, qubits are highly sensitive to their environment. This sensitivity leads to errors in calculations, limiting the reliability of quantum processors. The importance of overcoming this obstacle is paramount for practical applications of quantum technology.
A significant characteristic of these challenges is their multifaceted nature. They involve not only scientific hurdles but also engineering and technological limitations. Addressing these issues is crucial for the realization of practical quantum computers.
Uniquely, these challenges can serve as catalysts for innovation. Solutions developing from the need to manage qubit stability could lead to breakthrough technologies in both quantum and classical computing environments. Thus, the pursuit of overcoming these challenges is as valuable as the breakthroughs themselves.
Philosophical Implications
The advancements in quantum physics also provoke important philosophical questions regarding the nature of reality. Traditional views of determinism are challenged by quantum phenomena. The superposition and entanglement behaviors suggest that particles can exist in multiple states and influence each other instantaneously, regardless of distance.
These concepts force us to reconsider the fabric of reality. If our understanding of physics is rooted in classical mechanics, then quantum physics introduces a level of unpredictability and interconnectedness that is difficult to grasp fully.
Moreover, debates regarding the observer effect and the role of consciousness in measurement have emerged. Are observers necessary to collapse a quantum state into a defined outcome? Such inquiries lead to deeper discussions about perception and existence.
In summary, the future of quantum physics promises to reshape both technology and philosophy. It compels us to question not only how we understand the universe but also our fundamental interpretation of existence.
Closure
Summary of Current Developments
The recent breakthroughs in quantum research highlight a transformation in quantum computing capabilities. Enhanced processing power is being achieved through innovations such as superconducting qubits and trapped ions, which improve both speed and efficiency. Moreover, studies on quantum entanglement reveal new dimensions in communication systems. Researchers are uncovering fresh experimental findings that bolster the potential for secure information transfer. These advancements not only showcase scientific prowess but also open avenues for future exploration across myriad fields.
The Importance of Ongoing Research
Ongoing research in quantum physics is critical. It drives innovation and contributes significantly to technological evolutions in sectors like cryptography and materials science. As the field continues to evolve, the collaborative efforts between academia and industry are essential to sustaining progress. This synergy not only fosters breakthroughs in theoretical understanding but also promotes practical applications that can address global challenges. The importance of quantum physics cannot be understated; its implications for security, technology, and fundamental science echo through every layer of modern society.
"The pursuit of knowledge in quantum physics is not just academic; itβs redefining the landscape of technological possibility and leading us to an era of unprecedented advancement."