Understanding Quantum Physics: Principles and Impact
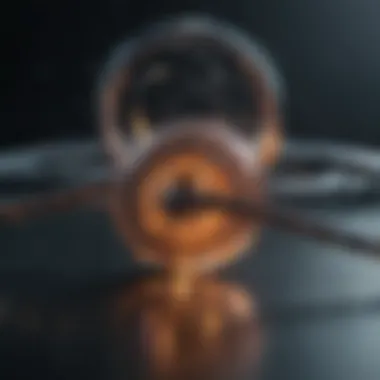
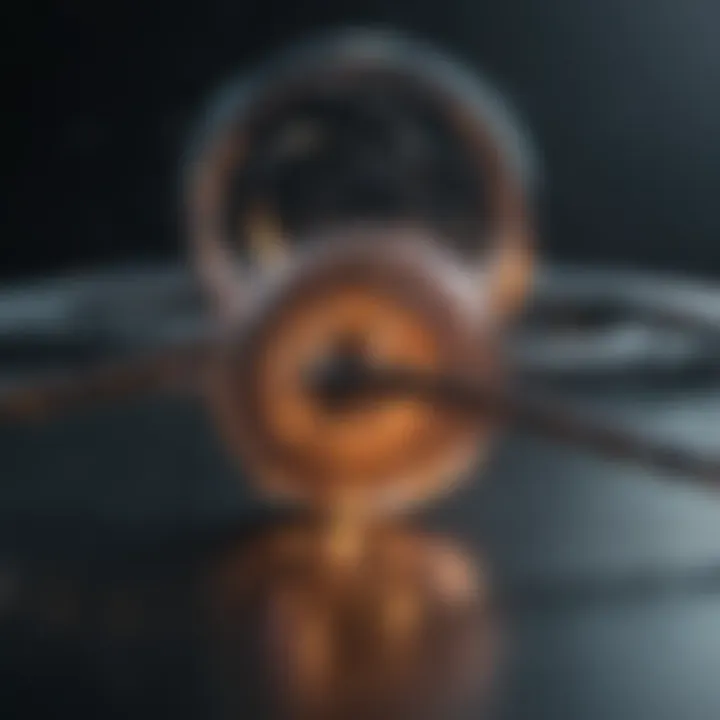
Intro
Quantum physics, often considered the most abstract of all scientific fields, delves into phenomena beyond our everyday experience. With its roots tracing back to the early 20th century, this discipline seeks to explain how the tiniest particles interact with one another and, ultimately, how they constitute the very fabric of our universe. For students, researchers, educators, and professionals, understanding quantum theory is essential for grasping the complexities of modern science.
This article aims to provide a comprehensive exploration of quantum physics, removing the barriers often associated with its intricate principles. By breaking down sophisticated theories into manageable concepts and analyzing recent research findings, it becomes easier to appreciate how quantum mechanics continues to revolutionize our understanding of nature. With a focus on both theoretical implications and practical applications, this journey into the quantum realm offers insights that touch various domains, from computing to medicine.
In the following sections, we will unfold the layers of quantum mechanics, highlight key research findings, and simplify complex ideas for better comprehension.
Key Research Findings
Overview of Recent Discoveries
Recent years have brought forward a trove of discoveries that advance our comprehension of quantum mechanics. For instance, the development of quantum entanglement has opened pathways for technologies like quantum computing and secure communication systems. The pivotal experiments conducted by physicists, such as the ones led by Alain Aspect in the 1980s, confirmed that entangled particles could affect one another instantaneously, regardless of distance. These findings hint at a reality we barely understand, where time and space seem to bend under quantum laws.
Important Discoveries Include:
- Quantum Superposition: The principle that a particle can exist in multiple states simultaneously until it is measured.
- Quantum Teleportation: A method of transferring information through quantum states, showcasing the potential for data transmission at phenomenal speeds.
- Quantum Computing Breakthroughs: Innovations in qubit manipulation that promise faster and more efficient computing.
Significance of Findings in the Field
The significance of these findings reaches far beyond academic discourse. They drive technological innovations that promise to reshape industries, particularly in computing and cryptography. As nations strive for competitive advantages in technology, understanding quantum mechanics becomes increasingly vital. Research labs worldwide hustle to develop quantum networks that could allow for instantaneous communication, promising a paradigm shift in how data is transmitted.
"The discoveries in quantum mechanics not only challenge our understanding of physics but also lay the groundwork for the future functionality of technology as we know it."
Breakdown of Complex Concepts
Simplification of Advanced Theories
To truly grasp quantum mechanics, one must tackle its foundational concepts. Terminologies often muddled with scientific jargon can create barriers. Here's a simple overview of essential theories:
- Wave-Particle Duality: The concept that every particle or quantum entity can exhibit both particle-like and wave-like behavior.
- Heisenberg's Uncertainty Principle: States that the position and momentum of a particle cannot be precisely measured at the same time.
- The Observer Effect: Indicates that the act of measurement affects the quantum system, highlighting the heavily interactive nature of quantum particles.
Visual Aids and Infographics
In an effort to demystify these concepts, visual representations are invaluable. They assist in bridging the gap between abstract theories and tangible understanding. Learners often benefit from diagrams that show particle interactions, entangled states, or even simple animations that illustrate wave functions.
By leveraging such resources, one can significantly enhance their grasp of these elusive principles while engaging more deeply with the content presented.
In sum, quantum physics stands at the forefront of scientific exploration. It challenges conventional wisdom and invites inquiry, not just into the universe's fundamental mechanics, but also into its very essence.
Foreword to Quantum Concepts
In the vast landscape of physics, quantum concepts play a crucial role in shaping our understanding of the universe at its core. Without a doubt, these principles not only provide insights into the behavior of matter and energy on the smallest scales but also reveal the profound implications that arise from this study.
When we look at the quantum realm, we are not merely examining particles; we are grappling with the very fabric of existence itself. This section aims to elucidate what quantum physics means, grounding our exploration in essential definitions and historical context. By doing so, we lay the groundwork for deeper discussions on core principles, applications, and the ongoing debate within the scientific community surrounding quantum mechanics.
The importance of grasping quantum concepts goes beyond the theoretical. It opens the door to practical applications that affect daily life, from advancements in technology to insights into the fundamental nature of reality. Understanding these principles equips students, researchers, educators, and professionals with the knowledge to navigate and contribute to a rapidly evolving field.
Defining Quantum
The term 'quantum' stems from the Latin word for 'how much.' In the realm of physics, it signifies discrete quantities of energy proportional to the frequency of radiation emitted by a physical system. Unlike classical mechanics, which describes the universe as a continuum, quantum mechanics introduces the idea that at microscopic scales, energy exists in specific, quantized packets. This contrasts sharply with the smooth, wave-like behaviors of larger scale entities.
To put it simply, quantum physics examines scenarios where the rules of classical physics fall short. When we enter this domain, phenomena that seem bizarre, such as particles existing in multiple states or being entangled across vast distances, start to make an appearance. The implications of defining quantum extend into multiple disciplines, reshaping not only physics but stretching into chemistry, computing, and even philosophy.
"Quantum physics reveals a world where intuition often leads one astray, compelling us to rethink our perceptions of reality."
Historical Background
The roots of quantum mechanics can be traced back to the early 20th century, a period marked by groundbreaking discoveries in physics. It all began when Max Planck, in 1900, proposed the idea that energy is emitted or absorbed in discrete units, or 'quanta.' This revolutionary idea fed into the work of Albert Einstein, who, in 1905, explained the photoelectric effect using Planck's concept of quanta, suggesting that light itself is made up of photonsβanother building block of quantum theory.
Subsequent developments followed swiftly through the years. Niels Bohr formulated his atomic model in 1913, introducing quantized energy levels for electrons around the nucleus. This model laid the foundation for further exploration of atomic structures, leading to the establishment of quantum theory.
In the following decades, luminaries such as Werner Heisenberg and Erwin SchrΓΆdinger contributed significantly, shaping the wave mechanics and the uncertainty principle, which posits that certain pairs of physical properties can't both be known to arbitrary precision.
As the 20th century progressed, quantum mechanics transcended mere scientific curiosity, permeating through technologyβushering in innovations like semiconductors and lasers, which are ubiquitous in modern life.
This historical backdrop sets the stage for our deeper dive into the core principles of quantum mechanics, which continue to challenge our perceptions and provoke essential questions. All these developments underscore how critical a firm understanding of quantum concepts is for anyone engaged in scientific endeavors today.
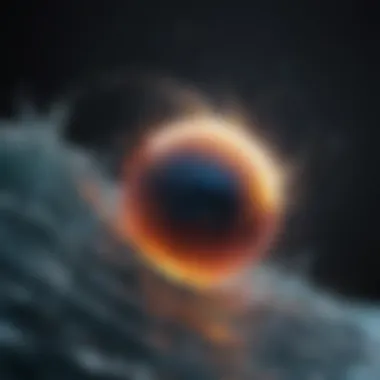
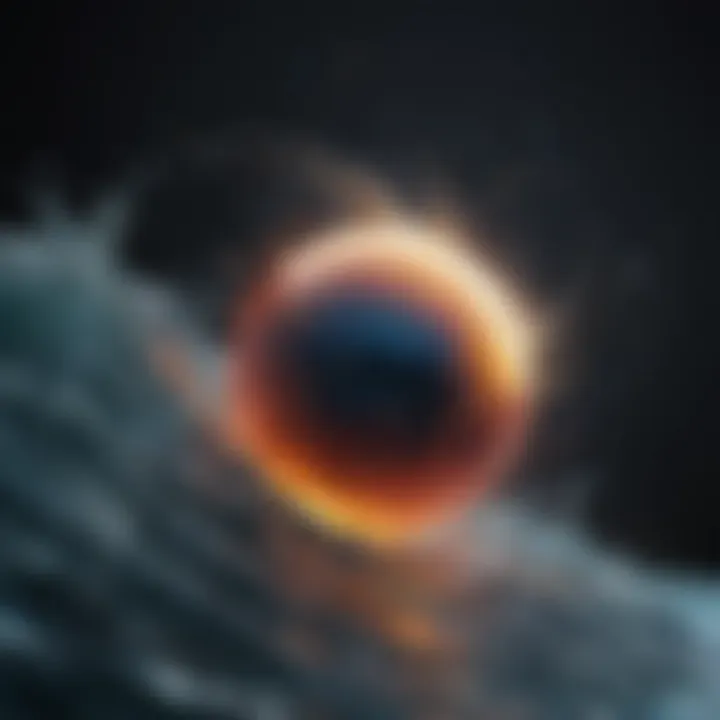
Core Principles of Quantum Mechanics
When delving into the intricate realm of quantum mechanics, understanding its core principles is akin to piecing together a complex puzzle. These principles not only define the behavior of particles at the minutest scale but also shape the very fabric of what we perceive around us. By comprehending these foundational ideas, we lay a crucial groundwork for exploring the broader implications of quantum theory in both technology and philosophy.
Wave-Particle Duality
Wave-particle duality stands as a cornerstone of quantum mechanics, challenging our long-held notions about the nature of light and matter. This principle indicates that every particle or quantum entity can exhibit both wave-like and particle-like properties. To illustrate, consider light: traditionally regarded as a wave, it also behaves as a stream of particles or photons in phenomena such as the photoelectric effect.
The acceptance of this duality raises numerous questions about the nature of reality itself. For instance, one must wonder: if particles can switch between forms, what does that say about their true existence? This dualistic nature is not just a theoretical construct; it has real-world applications that drive technologies ranging from semiconductors to lasers. As such, understanding wave-particle duality becomes essential for those attempting to navigate the quantum landscape.
Uncertainty Principle
Next comes the uncertainty principle, proposed by Werner Heisenberg, which states that one cannot simultaneously know both the position and momentum of a particle with absolute certainty. Simply put, the more precisely you try to measure one of these properties, the less precisely you can know the other.
This principle disrupts the classical expectation that objects can be observed and measured with complete accuracy. Instead, it introduces an inherent limitation to our knowledge. It's fascinating to consider how this principle has influenced various fields, not just physics but also philosophy, where it lends a layer of complexity to discussions about determinism and free will. The uncertainty principle illustrates that at the quantum level, probabilities reign supreme.
"The very act of observing a particle can alter its state, blurring the line between observer and observed."
Superposition
Superposition, another fundamental concept, deals with the way quantum systems can exist in multiple states simultaneously. Imagine flipping a coin; while it tumbles, it is neither heads nor tails but a mixture of both until you catch it. In quantum mechanics, particles do the same thing. They can exist in multiple states until measured.
This idea has profound consequences, particularly evident in quantum computing, where bits can represent 0, 1, or any combination of both, known as qubits. The ability of qubits to exist in superposition allows for incredibly fast computations, presenting an exciting frontier in technology that traditional computing can't even get close to. The implications of superposition extend into deeper philosophical realms as well, raising questions about reality and the nature of existence itself.
Entanglement
Entanglement is perhaps the most interestingβand perplexingβprinciple of quantum mechanics. When particles become entangled, the state of one particle becomes directly linked to the state of another, no matter how far apart they may be. A change to one entangled particle instantly affects the other, a phenomenon that Albert Einstein famously referred to as "spooky action at a distance."
This principle not only forces us to reconsider the concepts of space and locality but also underpins advances in quantum communication and cryptography. With entangled particles, secure information transfer becomes possible, paving the road for breakthroughs we'll likely come to rely on in future technologies.
In wrapping up this section, the core principles of quantum mechanics showcase a world fundamentally different from our everyday experiences. Each principleβwave-particle duality, uncertainty, superposition, and entanglementβplays a vital role in shaping modern physics and technology, urging us to question deep-seated beliefs about the physical world. Understanding these concepts not only enriches our knowledge but also illuminates the path ahead in both scientific inquiry and technological innovation.
Mathematical Foundations of Quantum Theory
The realm of quantum mechanics, while rich in phenomena and real-world applications, is fundamentally anchored in mathematical principles. Mathematical foundations provide the language and tools needed to understand and describe the complexities of the quantum world. They offer a systematic approach that allows physicists to express quantum concepts quantitatively, ensuring clarity and precision in a field often considered unintuitive.
Mathematics acts as a bridge connecting abstract theory to observable phenomena. It enables scientists to formulate hypotheses, design experiments, and interpret results with a level of rigor that is essential for advancing knowledge in this field. Without the robust mathematical frameworks, much of quantum theory would remain speculative, lacking the critical verification necessary for scientific validation.
Wave Functions and the SchrΓΆdinger Equation
At the heart of quantum mechanics lies the wave function, a mathematical function that encapsulates the quantum state of a system. The wave function, usually denoted by the Greek letter psi ((\psi)), provides a comprehensive description of a particleβs potential states and their associated probabilities. The pivotal SchrΓΆdinger equation governs the evolution of this wave function over time.
This equation, formulated by the physicist Erwin SchrΓΆdinger in 1925, is akin to Newton's laws in classical mechanics, providing a foundational framework by which we understand how quantum systems change. The equation can be expressed as:
[ i \hbar \frac\partial\partial t \Psi(t) = \hatH \Psi(t) ]\
where (\hatH) represents the Hamiltonian operator, encapsulating the total energy of the system. The resolution of the SchrΓΆdinger equation reveals the probabilities of finding a particle in various states, thus allowing physicists to predict outcomes in experiments.
Operators and Observables
In quantum mechanics, operators hold a critical position. They are mathematical entities that act on wave functions to extract measurable quantities known as observables. This relationship is deeply intertwined with the formulation of quantum theory. Observables such as position, momentum, and energy are represented by specific operators, which follow special rules of manipulation.
For instance, the position operator acts on the wave function to yield information about the position of a particle. The interplay between operators and observables gives rise to the concept of eigenvalues and eigenstates, crucial for understanding measurement outcomes in quantum experiments. As students or researchers dive into the quantum landscape, grasping the operator formalism is integral to their comprehension of the underlying mechanics.
Quantum State Representation
Quantum state representation is another layer of the mathematical foundation, defining how quantum states can be depicted in a rigorous manner. Two prevalent representations are the position representation and the momentum representation. While the position representation uses wave functions to illustrate the probability distribution across positions, the momentum representation communicates this information in the context of momentum values.
Moreover, the representation of quantum states extends to the bra-ket notation developed by Paul Dirac. This notation simplistically expresses states as vectors in a complex vector space, thereby facilitating easier manipulation of these states in calculations. It streamlines the notation for inner products and outer products, making transitions between states mathematically manageable.
Overall, grasping the mathematical foundations of quantum theory is not merely an academic exercise but an essential step in understanding the nuances and peculiarities that define quantum mechanics. By engaging with these mathematical structures, learners can illuminate the pathways that lead to discoveries and innovations within the field of quantum physics.
"Mathematics is the music of reason."
- James Joseph Sylvester
Understanding the layers of mathematical logic is pivotal for anyone embarking on a journey through the enigmatic world of quantum mechanics. The clarity, precision, and depth provided by these principles carry implications that resonate throughout science and technology today.
Applications of Quantum Mechanics
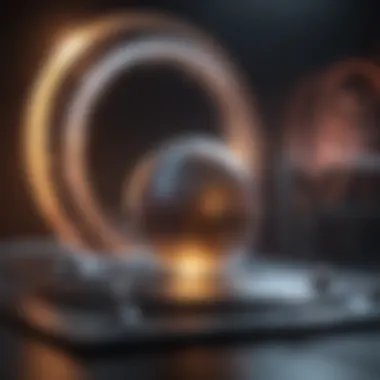
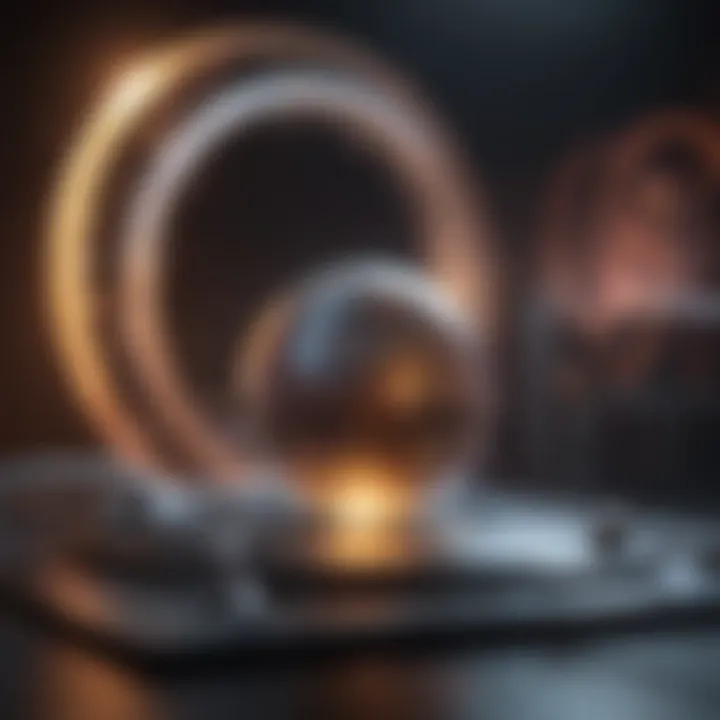
The applications of quantum mechanics are profound and far-reaching, redefining how we understand technology and its interaction with the physical world. This article unpacks key areas where quantum principles are not just theoretical musings but are deeply woven into the fabric of modern innovation. By shedding light on quantum computing, cryptography, and teleportation, we can appreciate how these concepts not only push the boundaries of traditional science but also present practical solutions to contemporary challenges.
Quantum Computing
Quantum computing stands at the forefront of technological evolution, bringing a fresh breath to computation. Unlike traditional computers that process bits as either 0 or 1, quantum computers use qubits, which can exist in multiple states simultaneously thanks to superposition. This characteristic enhances their computational power immensely.
The implications are vast:
- Efficiency: Tasks that currently take years on classical systemsβlike factoring large numbers or optimizing complex systemsβcan potentially be solved in minutes or even seconds.
- Machine Learning: Quantum computation can streamline data processing significantly, enhancing models and algorithms in machine learning, making them faster and more accurate.
- Simulations: In fields like chemistry and material science, quantum computing provides the ability to simulate molecular interactions that classical computers would struggle to model.
Consider IBM's Quantum Experience and Google's Quantum Supremacy demonstrations as the trailblazers paving the way into this new computational era. The potential for disruption is nothing short of astonishing.
Quantum Cryptography
In a world where data security is paramount, quantum cryptography emerges as a beacon of hope. By leveraging the principles of quantum mechanics, particularly the phenomenon of entanglement, it ensures secure communication in ways that were previously considered unattainable.
The key aspects include:
- Quantum Key Distribution (QKD): This method allows users to share keys securely, with any attempt to intercept the communication making the current key unusable. This entanglement feature ensures both parties are aware if their conversation is being snooped on.
- Immunity to Eavesdropping: Because of the fundamental laws of quantum mechanics, eavesdroppers canβt gather information without disrupting the transmission, triggering alarms.
Quantique and ID Quantique are leaders in this field, enabling institutions to adopt next-gen encryption methodsβmaking sensitive data not just secure, but quantum secure.
Quantum Teleportation
Quantum teleportation may sound like science fiction, yet it is a tangible result of quantum mechanics. It's not about teleporting physical objects, rather, it concerns the transfer of quantum states from one particle to another over distances. This phenomenon reveals fascinating implications for information transfer in quantum networks.
- Communication Beyond Distance: With the ability to transmit information instantaneously across vast distances, quantum teleportation offers tantalizing possibilities for a faster internet, devoid of traditional bandwidth limitations.
- Applications in Quantum Networking: As networks transition to quantum systems, teleportation could play a crucial role in developing a secure quantum internet, where information can be transmitted in a hack-free environment.
As researchers continue to push the envelope, entities like the University of Science and Technology of China are actively experimenting with teleportation, hinting at a future where such technology becomes integral to our communication systems.
"Quantum technology is not just a concept of the future; it is a reality that is shaping our present and will define our future."
In summary, the applications arising from quantum mechanics illuminate the potential for groundbreaking advancements. Each domainβbe it computing, cryptography, or teleportationβcarries the promise of transforming not only individual fields but also the broader scientific landscape. Understanding these applications is crucial as we stand on the brink of a quantum revolution.
Quantum Theory in Comparison with Classical Physics
The relationship between quantum theory and classical physics is a fascinating area of study that reveals a significant number of disparities. While classical physics outlines the macroscopic behavior of matter and energy, quantum theory operates at scale, diving into the minuscule world of atoms and subatomic particles. This distinction illustrates just how our understanding of reality shifts when we delve into the quantum realm.
Philosophical Implications
When we explore the philosophical implications of quantum mechanics compared to classical physics, it becomes clear that the foundational assumptions of how reality operates are challenged. Classical physics operates on a deterministic principle, which posits that if we know all variables in a system, we can precisely predict its future. In stark contrast, quantum mechanics introduces concepts such as randomness and probability.
A prime example of this change in outlook is represented through Heisenberg's Uncertainty Principle. This principle states that certain pairs of properties, like position and momentum, cannot both be accurately measured at the same time. This inherently indeterminate nature of quantum mechanics raises profound questions about the very fabric of reality. It forces us to reconsider the idea that the universe is a straightforward, predictable machine consistently adhering to fixed laws.
Furthermore, the notion of observer effectβa key idea in quantum mechanicsβsuggests that the act of measuring a quantum state can directly influence its outcome. This challenges classical views of observation as a passive act. So, instead of a clear, linear observation of the world, we find ourselves in a predictive realm where our interactions can fundamentally shape reality. Consequently, the philosophical quandaries brought forth by quantum mechanics are not merely academic; they usher in reflections about consciousness, reality, and free will.
Key Differences in Interpretations
The interpretations of quantum mechanics also diverge sharply from classical physics, resulting in an array of theories regarding how to understand quantum phenomena. Some noteworthy differences include:
- Copenhagen Interpretation: This is perhaps the most famous perspective, stating that physical systems generally do not have definite properties until they are measured.
- Many-Worlds Interpretation: Here, it's proposed all possible outcomes of quantum measurements are realized in some world or universe, leading to a multitude of coexisting realities.
- Pilot-Wave Theory: This interpretation insists there are hidden variables guiding quantum particles, providing a deterministic framework to understand their behavior.
Each interpretation carries different implications for our understanding of reality, scientific practices, and philosophical explorations. These diverse interpretations exemplify the ongoing debate within the scientific community regarding the nature of quantum mechanics.
"Quantum mechanics does not simply make strange predictions. It raises deep questions about the nature of reality itself."
Though classical physics provides a robust framework for understanding large-scale events, the incorporation of quantum principles alters our perspective fundamentally. A closer examination of these differences not only broadens our grasp of physics but also influences discussions about metaphysics and even the nature of consciousness. Engaging with both realms offers a richer narrative of the universe we inhabit.
Challenges and Controversies in Quantum Mechanics
Quantum mechanics, while revolutionary, is not without its challenges and controversies. Understanding these issues is essential for grasping the current landscape of quantum theory and what lies ahead. As quantum principles push the boundaries of traditional physics, they raise questions that philosophers, scientists, and even ethicists grapple with. Here, we will explore some of the key points surrounding the measurement problem, quantum nonlocality, and interpretational issues.
Measurement Problem
The measurement problem in quantum mechanics is a fundamental question that deals with the act of measuring a quantum system. In classical physics, measurements do not fundamentally disrupt the systems involved; however, when a quantum system is observed, it appears to change state from a superposition of possibilities to a single outcome. This raises pressing questions about the nature of reality itself. Does a quantum state exist as it is until measurement occurs, or do we create the reality by observing it?
"The act of measurement brings forth a reality in quantum mechanics, challenging our understanding of observation and existence."
Several interpretations have been proposed to tackle this problem:
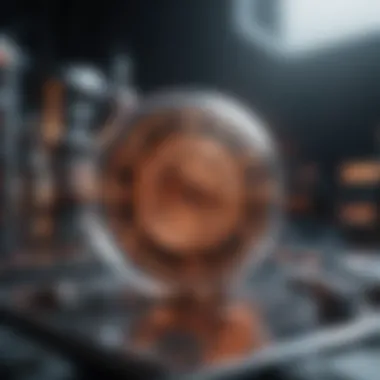
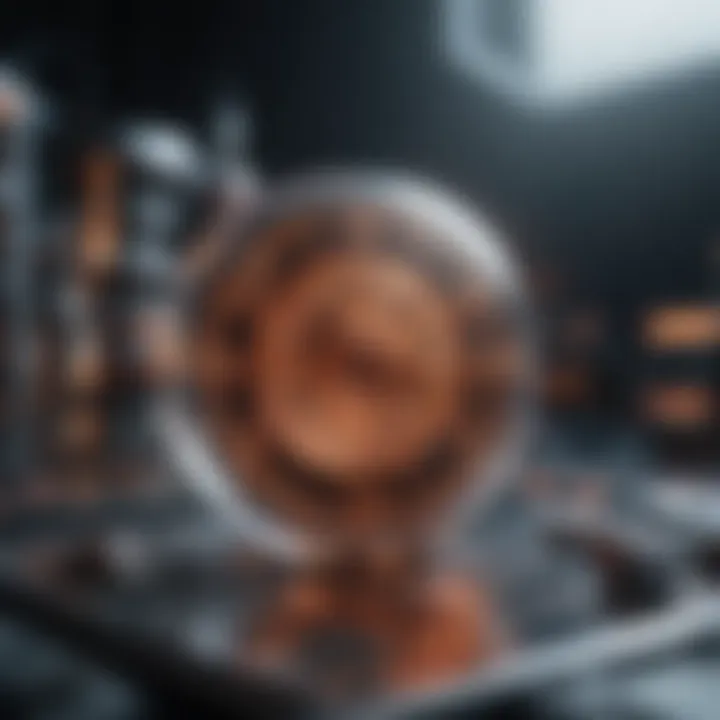
- The Copenhagen interpretation suggests that the wave function collapses upon observation.
- Many-worlds interpretation posits that all possible outcomes happen, each in its universe.
- Objective collapse theories argue that wave functions collapse spontaneously, independent of observation.
Rounding up the different interpretations highlights the ambiguity and challenges tied to measuring quantum phenomena. Thus, the measurement problem remains one of the central controversies, influencing debates in both physics and philosophy.
Quantum Nonlocality
Quantum nonlocality stands as a radical departure from classical intuition about separability and distance. It comes to life vividly in thought experiments like the famous Einstein-Podolsky-Rosen (EPR) paradox, where entangled particles appear to affect one another instantaneously, regardless of the distance between them. This challenges the very notion of locality that underpins classical physics.
Nonlocality hints that particles can be connected in ways that seem to defy common sense. Imagine two particles, say A and B, light-years apart. When you measure the state of particle A, it instantaneously affects the state of particle B. This leads to the conclusion that information is shared between them faster than the speed of light, which challenges the relativistic view of causality.
Some scientists argue that nonlocality might allow for faster-than-light communication; others dismiss this idea as mere philosophical speculation. Nevertheless, the phenomenon has been experimentally verified, and it raises profound questions about the interconnectedness of the universe.
Interpretational Issues
Interpretational issues are another layer of complexity in quantum mechanics. The interpretation of quantum mechanics is deeply philosophical, diving into debates about what quantum mechanics says about reality itself. For instance, the question of whether quantum mechanics is complete or merely an approximation of a deeper theory looms large.
Key interpretations include:
- Copenhagen Interpretation: It suggests reality is defined by measurement, leaving non-observed phenomena ambiguous.
- Many-Worlds Interpretation: Every possible outcome occurs in separate branches of reality, raising questions about the nature of existence and experience.
- Pilot-Wave Theory: Advocates for a deterministic approach, positing hidden variables that dictate the behavior of particles.
These interpretations are more than academic exercises; they have implications for how we understand causality, free will, and the very fabric of reality. Given that quantum mechanics is successful in providing accurate predictions, the issue now is not if quantum mechanics works but how we interpret what it means.
By laying bare these challenges and controversies, we can better appreciate the nuanced and often perplexing world of quantum mechanics. Each of these elements enriches the discourse around quantum theory, inviting further exploration and understanding.
Recent Developments in Quantum Research
Recent developments in quantum research have stirred considerable excitement in both scientific circles and everyday discussions. The implications of new discoveries extend beyond the laboratory, sparking conversations about technology, ethics, and the role of science in our lives. The rapidly evolving field of quantum mechanics is reshaping our understanding of reality and opens doors to technologies once thought to be mere science fiction.
Advancements in Quantum Technologies
One of the most notable advancements in quantum technology is the development of quantum computers. Unlike classical computers that operate using bits as the smallest unit of data, quantum computers utilize qubits. A qubit can exist in multiple states at once due to the principle of superposition, enabling quantum computers to perform complex calculations much faster than traditional computers.
Recent breakthroughs in this area include:
- Increased Qubit Stability: Researchers are now developing methods to stabilize qubits for extended periods, reducing errors that arise from decoherence. Innovations include using superconducting materials, which present lower resistance, thereby preserving quantum states longer.
- Quantum Supremacy: Google claimed to have achieved quantum supremacy in 2019, solving a problem in a fraction of the time it would take the most powerful classical supercomputer. While this claim is still debated, it marks a significant milestone in potential applications of quantum technology.
- Quantum Networking: The groundwork for quantum internet is being laid, allowing secure communication that leverages the principles of quantum entanglement. This could revolutionize data security, making it virtually unhackable.
Notable Scientific Experiments
As quantum research progresses, various experiments stand out for their ingenuity or implications. For instance, a team at the University of Science and Technology of China managed to demonstrate quantum teleportation over a distance of more than 100 kilometers. This experiment not only underscores the capabilities of quantum entanglement but also nudges us closer to a fully functional quantum communication network.
In another remarkable endeavor, researchers have conducted experiments observing states of light that challenge classical assumptions.
- Bell Test Experiments: These experiments reinforce the existence of quantum entanglement by illustrating how particles separated by vast distances can instantaneously affect each other's states, defying classical logic.
- Quantum Eraser Experiments: These shed light on the nature of measurement in quantum mechanics, suggesting that the past can be altered by current actions, hence blurring the lines between causality.
"Quantum experiments not only test our theoretical models but also challenge our very understanding of reality itself."
Future Directions in Quantum Research
Looking ahead, the future of quantum research holds immense potential across various fields. Several key areas are emerging as focal points for inquiry and development:
- Material Science: Researchers are investigating quantum materials that could lead to breakthroughs in superconductivity, helping to create energy-efficient systems.
- Quantum Biology: There's an increasing interest in understanding quantum effects in biological processes, like photosynthesis. This could unlock new ways of harnessing energy from the sun.
- Quantum Machine Learning: The intersection of artificial intelligence and quantum computing may create algorithms that outperform today's classical models.
- Interdisciplinary Applications: As quantum technologies evolve, the collaboration between physics and other scientific disciplines will become critical in realizing new innovations.
Epilogue and Future Outlook
As we draw the curtain on our exploration of quantum physics, it becomes ever clearer that this domain is not merely an esoteric field reserved for theoretical physicists. Rather, it has woven itself into the very fabric of modern scientific inquiry, continually reshaping our understanding of the universe. The journey through the principles of quantum mechanics, its applications, and what lies ahead illustrates the profound importance of this subject not only for academia but for society at large.
The Evolving Landscape of Quantum Physics
The landscape of quantum physics is truly a shifting terrain, much like a river that carves its own path through solid rock over time. Recent advancements, such as the ongoing debates surrounding quantum information theory and quantum computing, underscore the vitality of this field. Scholars and practitioners are constantly pushing boundaries, challenging conventional thought, and, in turn, redefining what we understand about reality.
For instance, the advent of quantum computing offers a glimpse into a future where computational problems deemed intractable today could soon be tackled with unprecedented efficiency. This paradigm shift could drastically alter sectors from cryptography to drug discovery, making the implications of quantum research far-reaching. Schools of thought concerning the interpretations of quantum mechanics continue to thrive; each offering unique insights into the nature of reality, probability, and causation. The role of practice in quantum sciences, although occasionally a muddy one, remains crucial in clarifying and validating theoretical foundations. Even as the theories evolve, so too does the technology and its applications.
Additionally, educational institutions must not turn a blind eye to these developments. As quantum literacy becomes an essential element of a well-rounded scientific education, preparing the next generation of thinkers will be key. By integrating quantum principles into curricula today, we prepare a foundation for future breakthroughs.
Implications for Science and Society
The societal implications of quantum physics are immense. Consider, for example, the burgeoning field of quantum cryptography. As we move deeper into the digital age, the security of data becomes paramount. Quantum cryptography introduces concepts like quantum key distribution, presenting a significant step forward in safeguarding information in an era where data breaches are alarmingly commonplace.
Moreover, quantum technologies also go beyond mere data security. For healthcare, quantum sensors can enhance imaging techniques, improving diagnostic accuracy and outcomes. In climate science, powerful quantum simulations could model complex systems with far greater precision than traditional methods.
"The impact of quantum research will not be confined to laboratories; its ripple effects are destined to touch every aspect of our lives, from global health to ecological sustainability."
The web of interconnectedness woven by quantum mechanics highlights a crucial point for policymakers and society: the need for continued investment and interest in this field cannot be overstated. As quantum science continues to intertwine with emerging technologies, the opportunities for innovation are boundless.
As the curtain falls on our examination of quantum physics, itβs evident that the future holds a great deal of promise and potential for transformative change. The challenges remain real, but so do the opportunities. Engaging with quantum physics today lays the groundwork for a smarter, safer, and more efficient tomorrow. The final chapter in this scientific saga has yet to be written, but it undoubtedly holds the promise of something extraordinary.