Exploring the Depths of Quantum Physics
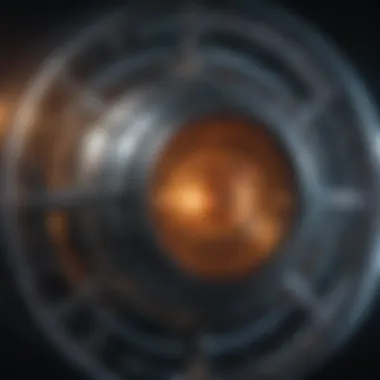
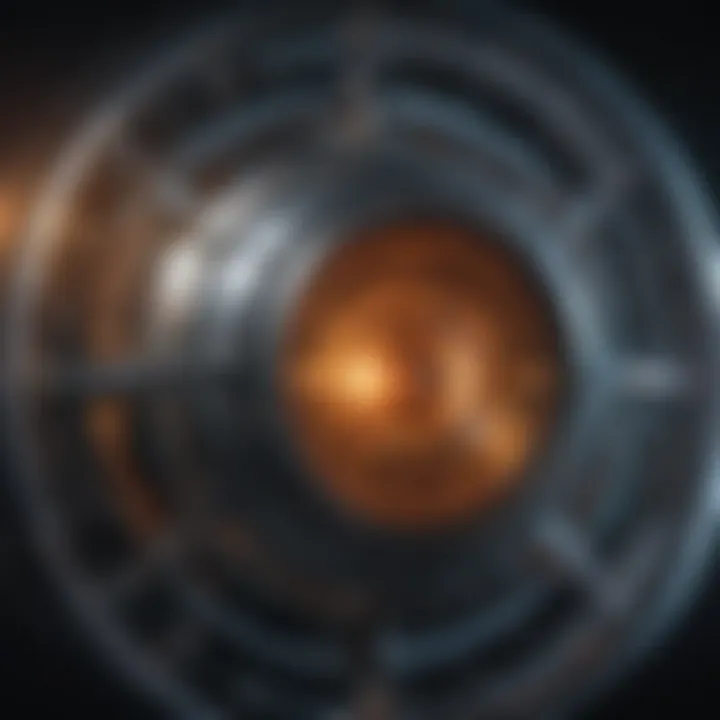
Intro
In a world governed by the rules of the very small, quantum physics emerges as both a framework of understanding and a catalyst for innovation. This discipline, while dense and often intimidating, invites students, researchers, educators, and professionals alike to explore a dimension that fundamentally alters our perception of reality. The laws of quantum mechanics, stretching back to the early 20th century, have sparked a revolution in our comprehension of the universe, unearthing profound implications that intertwine theoretical nuances with practical consequences.
Quantum physics compellingly addresses the enigma of behavior at microscopic levels. It navigates the peculiar characteristics of particles which often do the unexpected—like being in two places at once. This article embarks on an exploration of quantum mechanics, breaking down its foundational principles, significant theories, and the myriad advancements that characterize this cutting-edge field.
By offering an insight into this complex domain, we aim to cultivate a deeper understanding of how quantum theories have evolved and their relevance in contemporary science. In pursuit of that understanding, we find ourselves peeling back the layers of reality, guiding through the fog of perplexities that quantum phenomena present.
In the sections that follow, we will delve into the latest happenings in quantum research and the significance of these findings within the larger scientific narrative. Subsequently, we will deconstruct intricate concepts so they become accessible to a broader audience, ultimately revealing the practicality that quantum physics holds in our lives.
That said, let's embark on this intricate journey into the quantum realm.
Prologue to Quantum Physics
Quantum physics, a domain that challenges our understanding of the universe, serves as the foundation for many modern scientific advancements. Whether one is developing quantum computers or delving into quantum cryptography, grasping the fundamentals of this complex field is crucial. This section aims to initiate readers into the essential concepts of quantum physics and emphasize its significance across various disciplines.
Understanding quantum physics is not merely an academic pursuit. For students, researchers, and professionals alike, it lies at the heart of many technologies we often take for granted. Development in materials science, electronics, and even drug development increasingly relies on quantum principles. The benefits are profound, promising faster computing speeds, enhanced security in communication, and innovations that reshape our daily lives.
Moreover, the myriad of concepts in quantum physics raises deep philosophical questions about the nature of reality itself. This interplay between the tangible applications and their abstract foundations provides a fertile ground for exploration. As we navigate through this article, the historical evolution, key principles, and contemporary applications will be dissected to unveil the profound impacts of quantum physics on our understanding of the universe.
Historical Context
In order to appreciate quantum physics in its current state, it's necessary to rewind the clock and look at its origins. The evolution of quantum theory began in the early 20th century, a time when classical physics was deemed wholly sufficient to explain day-to-day phenomena. Think about it; Einstein’s theory of relativity dominated, and scientists believed they had a firm grip on the laws governing nature.
However, anomalies started cropping up. Classic phenomena, such as blackbody radiation, led to questions that classical laws couldn't answer. Max Planck's 1900 proposal of quantized energy levels represented the first crack in the classical foundation. He introduced the concept of 'quanta'—discrete packets of energy—leading to a wave of thought that fundamentally changed physics.
Following Planck, notable figures like Niels Bohr, Werner Heisenberg, and Erwin Schrödinger contributed to developing quantum mechanics, each bringing in their unique perspectives and equations that challenged the accepted norms. These pioneers presented a mosaic of ideas fractured but interrelated, leading to quantum mechanics as we know it today.
This historical framework reveals not only a scientific evolution but also a cultural shift—one that encourages questioning and reimagining the nature of reality. The significance of these initial discoveries cannot be overstated, as they laid the groundwork for demystifying the intricacies of the microscopic world.
Basic Definitions and Concepts
Diving into quantum physics requires unpacking some fundamental notions that serve as building blocks for more complex ideas. First, let's talk about what we mean when we say "quantum." Simply put, it refers to the smallest possible discrete unit of any physical property. Concepts such as energy and matter behave quite differently at this microscopic scale compared to what we observe daily.
One of the core tenets of quantum physics is superposition: the idea that particles can exist in multiple states simultaneously until observed. Imagine flipping a coin; while in the air, it could be seen as both heads and tails—it's only upon landing that reality takes shape. This bizarre phenomenon challenges our traditional understanding of existence and reality.
Moreover, we cannot ignore entanglement, another fascinating concept. It implies that particles can be interconnected, such that the state of one particle instantaneously affects the state of another, regardless of the distance separating them. This kind of spooky action at a distance raises eyebrows and questions about causality and locality.
To bring it all together, grasping these foundational terms equips readers to delve deeper into quantum discussions throughout this article. Each concept builds upon the last, forming a tapestry of quantum behavior that exists both in theory and application, inviting further examination into the uncharted territories of the quantum world.
Key Principles of Quantum Mechanics
Quantum mechanics stands as the cornerstone of modern physics, shaping our understanding of the universe at its most fundamental levels. The principles of quantum mechanics are not merely theoretical constructs; they have profound implications on technology, philosophy, and our perception of reality itself. The importance of these principles cannot be overstated, as they serve as pivotal guidelines in navigating the enigmatic waters of quantum phenomena.
Wave-Particle Duality
At the heart of quantum mechanics lies wave-particle duality, a concept that suggests particles, such as electrons and photons, exhibit both wave-like and particle-like properties. This principle ushers in a new way of thinking that bends our traditional notions of physical objects. In some experiments, such as the famous double-slit experiment, light displays interference patterns characteristic of waves. Yet, in others, it behaves as discrete packets of energy, or particles.
One might ask, what does this duality mean in practical terms? For one, it lays the groundwork for understanding phenomena such as quantum tunneling, where particles pass through potential energy barriers as if they are waves, rather than being restricted by classical mechanics. This bizarre aspect is why your smartphone can operate on principles that, at first glance, seem far removed from everyday experiences.
"Wave-particle duality reveals that nature does not adhere to the binaries we impose on it; it’s a dance of probabilities, much like the varied rhythms of life itself."
Quantum Superposition
Another foundational element of quantum mechanics is quantum superposition. This principle states that a quantum system can exist in multiple states simultaneously until measured or observed. This fundamental uncertainty draws a stark contrast to classical mechanics, where objects exist definitively in one state or another.
To illustrate, consider Schrödinger's cat— a thought experiment where a cat in a box exists in a state of being both alive and dead until someone opens the box. This thought experiment shines a light on the puzzling implications of superposition, which surface frequently in technologies such as quantum computing. In quantum computing, data is not just a series of binary states (0 or 1) but can exist in multiple states at once, allowing for computational processes far more complex than classical counterparts.
Quantum Entanglement
Last but certainly not least is quantum entanglement. This principle describes a phenomenon where particles become interconnected in such a way that the state of one immediately influences the state of another, regardless of the distance separating them. It is as if they are making instant decisions together, transcending the limitations of space.
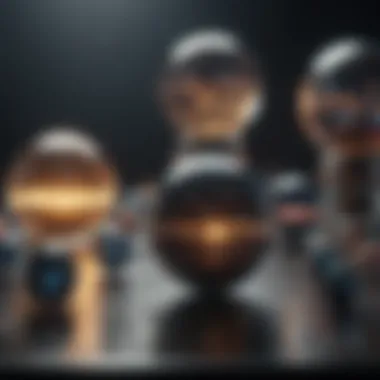
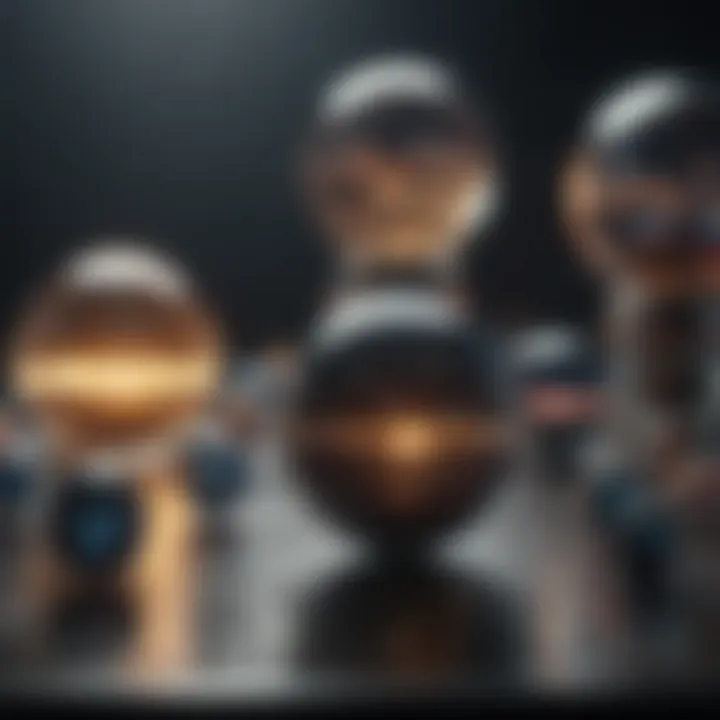
Entanglement has profound implications for information transfer and communication systems. This is the backbone of quantum cryptography, where the security of information is fortified due to the instantaneous nature of particle state changes. Practically, if one were to observe or measure one of the entangled particles, the complementary state of the other particle becomes evident, rendering eavesdropping attempts detectable.
In summary, the principles of quantum mechanics—wave-particle duality, quantum superposition, and quantum entanglement—provide a framework that not only explains the behavior of matter and energy but also opens the door inviting revolutionary advancements in technology and our understanding of the universe. As we journey deeper into the realms of quantum physics, these principles will continue to shed light on the intricate tapestry of reality.
Mathematical Framework of Quantum Physics
The mathematical framework of quantum physics serves as the backbone of its theoretical structure. Without it, the very concepts that underpin quantum mechanics would remain abstract and largely unfathomable. This framework enables researchers to describe quantum phenomena through precise mathematical language, thereby bridging gaps between theory and measurable results. By using well-defined mathematical constructs, scientists can capture the complexities of quantum behavior in a form that can be analyzed, predicted, and, most importantly, verified.
In this domain, the elegance of mathematics is not merely an aesthetic but an essential tool for understanding how quantum systems operate. Key elements of this framework include not only equations but also intricate constructs like wave functions and matrix mechanics. Together, these elements allow us to explore the bizarre, often counterintuitive realm of quantum phenomena.
The Role of Schrödinger Equation
At the heart of quantum mechanics lies the Schrödinger equation. Formulated by the physicist Erwin Schrödinger in the 1920s, this equation describes how the quantum state of a physical system changes over time. Simply put, it provides the mathematical means to understand the evolution of wave functions.
The Schrödinger equation is pivotal because it unites the abstract concepts of quantum physics with tangible results, allowing experiments to verify theoretical predictions.
The time-dependent Schrödinger equation, which governs a changing system, can be expressed as:
[ i \hbar \frac\partial\partial t \Psi(\mathbfr, t) = \hatH \Psi(\mathbfr, t) ]\
Where:
- ( \hbar ) is the reduced Planck constant,
- ( \Psi(\mathbfr, t) ) represents the wave function, and
- ( \hatH ) is the Hamiltonian operator, depicting the energy of the system.
This equation allows one to make predictions about the behavior of particles, such as electrons, and leads to insights about their potential locations, energies, and other properties. Understanding this is crucial for anyone diving into the nitty-gritty of quantum physics.
Operators and Observables
In quantum mechanics, operators play a significant role as they are used to extract information from the wave functions. Unlike classical physics, where variables can be directly measured, quantum systems are probabilistic at their core. Observables like position, momentum, and energy are not merely identifiable attributes; they are represented mathematically by operators.
This representation introduces a unique twist in how we understand measurement in the quantum realm. When an observable is measured, it yields a value from a range of possibilities, with the corresponding probabilities dictated by the wave function. For instance, the momentum operator is defined as:
[ \hatP = -i \hbar \nabla ]\
Where ( \nabla ) is the gradient operator. By applying operators to wave functions, one can retrieve measurable outcomes, which makes mastering these operators essential for anyone engaged in quantum physics.
In summary, the mathematical framework of quantum physics—a synthesis of equations, operators, and states—not only enriches our understanding of quantum mechanics but also empowers scientists and engineers to explore and apply these principles in innovative ways.
Quantum Theories and Models
In the broad tapestry of quantum physics, the significance of Quantum Theories and Models cannot be overstated. They serve as the backbone of our understanding, providing frameworks through which we interpret the peculiar behaviors observed at the microscopic level. These models not only pave the way for groundbreaking discoveries but also influence emerging technologies in various fields, including computing, cryptography, and even medicine.
Quantum theories offer a lens to examine phenomena that challenge our classical intuitions. They encapsulate abstract concepts and translate them into mathematical structures and predictive frameworks. For practitioners, be it students or seasoned researchers, grasping these theories unlocks a deeper appreciation for the quantum reality that underpins our universe.
Quantum Field Theory
Quantum Field Theory (QFT) stands at the intersection of quantum mechanics and special relativity. This framework extends quantum principles to fields rather than just particles. In essence, particles are excitations of these underlying fields, allowing for a more nuanced understanding of interactions.
One crucial aspect of QFT is its treatment of virtual particles. These particles exist for brief moments, allowing forces to mediate interactions between real particles. It’s a rather abstract idea; still, its implications are profound. For example, QFT underpins the Standard Model of particle physics, which encapsulates our best understanding of the forces that govern fundamental particles.
Additionally, QFT provides tools for predicting the outcomes of high-energy collisions in particle accelerators. These predictions resonate on the experimental front, making QFT not just an elegant theoretical construct, but also a practical framework on which to build experiments.
Quantum Electrodynamics
Diving deeper, we encounter Quantum Electrodynamics (QED), the quantum theory that describes how light and matter interact. QED is one of the most accurately tested theories in physics, successfully explaining phenomena like the fine structure of hydrogen and the anomalous magnetic moment of the electron.
QED posits that light consists of photons, which act as exchange particles between charged particles, such as electrons. When these charged particles interact, they 'emit' and 'absorb' photons. It’s an intricate dance where virtual photons facilitate interactions. The meticulous calculations involved in QED, although complex, yield predictions that align almost perfectly with experimental results, often to an astonishing degree of precision.
The richness of QED is not just in its predictive power. It also offers insights into the nature of reality itself, blurring the lines between particles and waves, and prompting philosophical discussions about the essence of existence at quantum scales.
String Theory and Quantum Gravity
String Theory ventures into a realm where it attempts to unify quantum mechanics with gravity, offering a broader perspective beyond current models. Unlike point particles, String Theory posits that the fundamental building blocks of the universe are one-dimensional strings. These strings can vibrate at different frequencies, giving rise to various particles we observe.
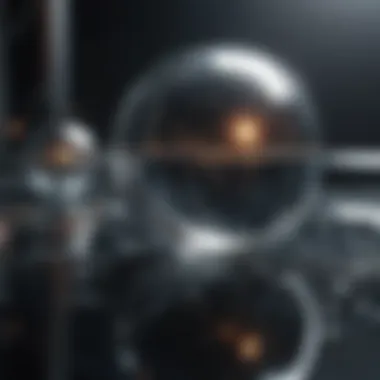
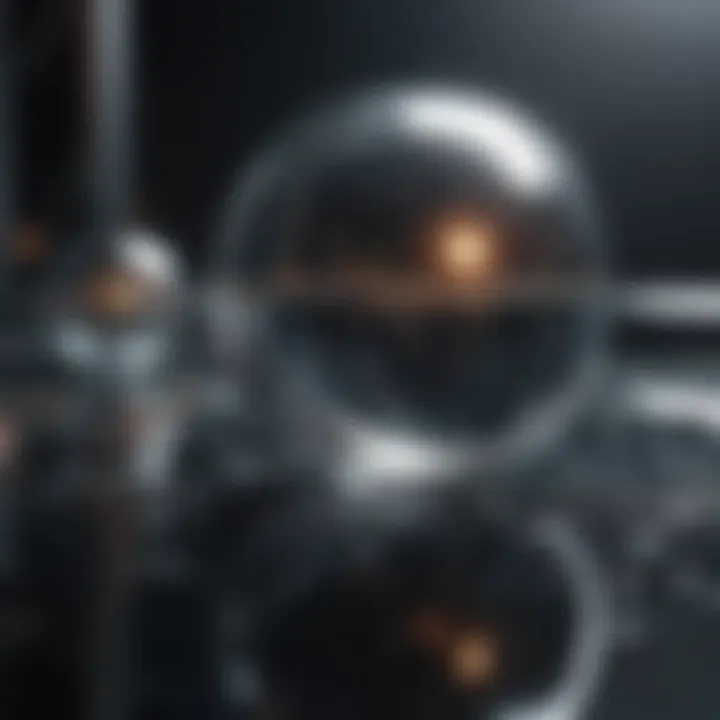
The crux of String Theory lies in its ambition to reconcile quantum mechanics with general relativity. Traditional quantum models falter when gravity is incorporated, leading to inconsistencies. Here, String Theory shines, presenting a framework that naturally incorporates gravity through multidimensional spaces and the concept of branes.
It is important to note, however, that String Theory remains largely theoretical, with numerous dimensions, such as 10 or 11, posing a challenge to experimental verification. Nonetheless, its potential for explaining the foundational forces of our cosmos keeps it at the forefront of physicists' minds.
In summary, Quantum Theories and Models form an essential part of our scientific lexicon, enabling us to navigate and make sense of the surreal landscape of quantum mechanics. As we continue to tease apart these complex concepts, we inch closer to a comprehensive understanding of everything from the smallest particles to the cosmos itself.
"The deepest truths of the universe may be woven into the fabric of the smallest strings."
As we probe these theories further, we build a more profound connection with the universe, illuminating paths toward new technologies and deeper questions about existence.
Applications of Quantum Physics
In the realm of modern technology and scientific advancement, quantum physics stands at the forefront. The principles derived from this complex field have shaped innovations that influence various aspects of our lives. Understanding the applications of quantum physics not only deepens our grasp of the subject itself but also reveals its monumental significance in practical uses that are changing the world.
The moment we mention quantum physics, terms like quantum computing, quantum cryptography, and even quantum teleportation spring to mind. Each of these applications hinges on the unique principles governing particles at the subatomic level, offering novel solutions to complex problems. Here, we delve into these applications and their implications.
"Quantum physics may unlock doors to the future that were once inconceivable. The gateway is open, but it takes the curious mind to traverse it."
Quantum Computing
Quantum computing represents a paradigm shift in how we process information. Unlike classical computers, which rely on bits as the smallest unit of data, quantum computers utilize quantum bits or qubits. Qubits have a unique ability to exist in multiple states simultaneously, thanks to the principle of superposition. This means they can carry more information at a faster rate compared to their classical counterparts.
For instance, a quantum computer could solve complex problems—be it simulating molecular interactions for drug discovery or optimizing logistics in real-time—at speeds that would take classical computers millions of years. Industries like finance, pharmaceuticals, and artificial intelligence are already eyeing quantum computing as a potential game-changer.
Key benefits of quantum computing include:
- Enhanced Computational Power: Solving problems that have long perplexed traditional computing methods.
- Speed and Efficiency: Performing massive calculations in seconds, facilitating breakthroughs in research and development.
- Improved Simulations: Allowing scientists to model intricate systems and phenomena more accurately.
Quantum Cryptography
As data breaches and cyber threats loom larger in our digital age, quantum cryptography offers a strong shield. At its core, it utilizes the principles of quantum mechanics to secure information. A key element is quantum key distribution (QKD), which ensures that any attempt at eavesdropping disrupts the communication itself—alerting both parties involved.
Imagine sending a password using classical methods; if intercepted, it can be copied without either party knowing. In contrast, quantum encryption makes it such that an eavesdropper alters the state of the data, thus instantly revealing their presence. This level of security promises not just to protect sensitive information, but to enhance trust in digital communications fundamentally.
Some notable features of quantum cryptography include:
- Unconditional Security: The security is not based on the computational power or algorithms but on the laws of quantum physics.
- Practical Implementations: Organizations like banks and government institutions are exploring quantum encryption methods for securing their communications.
Quantum Teleportation
One of the most mind-boggling applications tied to quantum physics is quantum teleportation. Don’t be misled by the sci-fi connotations; this process doesn’t allow for instantaneous travel through space. Instead, it refers to the ability to transfer the quantum state of a particle from one location to another without moving the particle itself. This incredible concept arises from the phenomenon of quantum entanglement, where pairs of particles become interconnected in ways that challenge our traditional views of locality.
Though it sounds surreal, it has substantial implications for future technologies. Quantum teleportation could revolutionize communication systems, allowing for the transmission of data across vast distances without the risk of interception. It's worth noting that current experiments are in preliminary stages, yet the potential permeates fields ranging from networking to quantum computing.
In summary, the applications of quantum physics not only illustrate its principles in action but also showcase pathways to future innovations. Through these technologies, we stand on the brink of a significant transformation that may redefine our understanding and interaction with the world.
Quantum Physics and Philosophy
Quantum physics is not just a field woven from mathematical equations and empirical data; it's a frontier where science and philosophy intersect, challenging our deepest intuitions about reality. The complexities inherent within quantum mechanics prompt profound questions that transcend traditional scientific discourse, compelling philosophers and physicists alike to grapple with fundamental concepts of existence, observation, and understanding the nature of reality.
By delving into quantum physics, one unavoidably confronts puzzles that ignite philosophical debates. How do we truly know what we observe? What is the nature of reality when it can be both a wave and a particle, depending on how we choose to measure it? The implications of quantum theory extend far beyond theoretical musings; they influence how we perceive, understand, and interact with the world around us.
The philosophical discussions surrounding quantum physics highlight significant elements such as:
- The Role of the Observer: Central to quantum mechanics is the notion that the act of observation can influence physical systems. This raises questions about the nature and purpose of observers in scientific experiments.
- Reality and Existence: What does it mean for something to exist if it can be fundamentally altered upon observation? Such inquiries drive a wedge into the very definition of reality itself, as quantum entities behave in ways that starkly contrast with classical physics.
- Determinism vs. Indeterminism: Classical physics operates under a deterministic framework, where outcomes are predictable given initial conditions. In contrast, quantum mechanics introduces elements of randomness, challenging the traditional belief in causality.
In contemplating these concepts, the benefits are manifold. Engaging with the philosophical ramifications of quantum physics enriches our understanding and fosters interdisciplinary collaboration. Students and researchers alike can benefit from contemplating these questions, as insights from philosophy may illuminate complex quantum phenomena.
"The butterfly effect in quantum physics suggests that a small change could have far-reaching effects on the system as a whole."
Moreover, examining quantum mechanics through a philosophical lens can help broaden the dialogue regarding ethical considerations in technology, particularly with advancements in quantum computing and cryptography.
As we navigate through this intricate web of ideas, it's essential to remain attentive to the considerations unique to this domain:
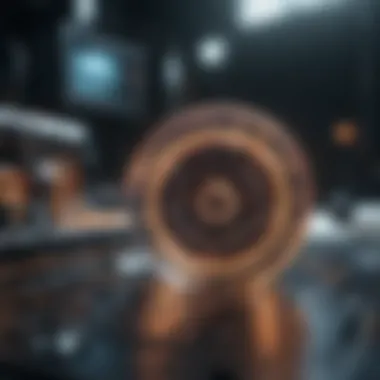
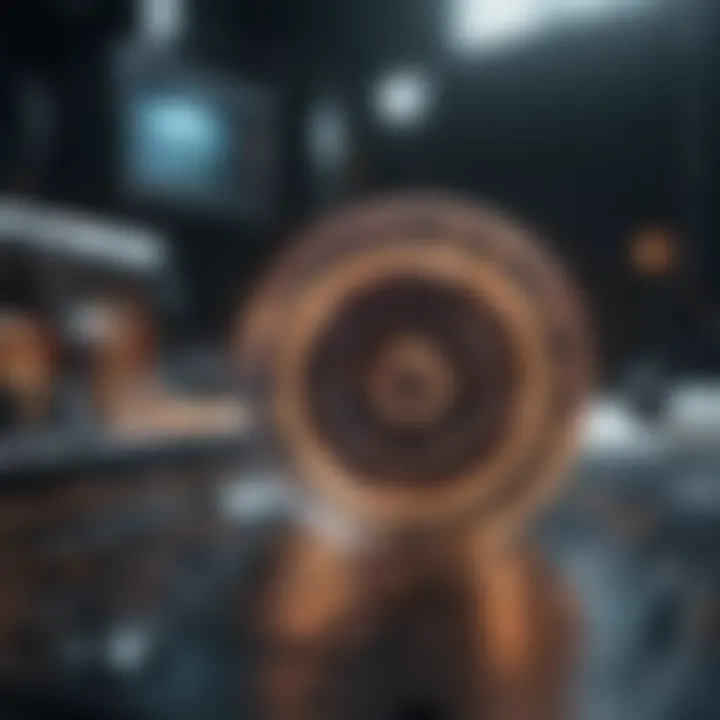
- The Uncertainty Principle: This principle doesn't just reflect uncertainty in measurements—it's a profound shift in our understanding of certainty itself. The more precisely you know one aspect of a system, the less precisely you can know another.
- Philosophical Interpretations: Disparate interpretations of quantum mechanics—ranging from the Copenhagen interpretation to the many-worlds interpretation—serve not only as scientific theories but also as gateways into deeper philosophical discussions about parallel realities and the nature of existence.
In summary, the interplay between quantum physics and philosophy invites a richer dialogue that challenges our cognitive frameworks. It is through this discourse that we can hope to arrive at not just a greater understanding of quantum mechanisms but also an appreciation for the intricate tapestry of reality itself.
Challenges and Limitations
In the vast landscape of quantum physics, understanding the challenges and limitations that researchers face is crucial. These obstacles reshape our approach to the quantum realm, influencing not only theoretical frameworks but also experimental practices. Grasping these hurdles provides a complete picture of the field’s current status and sets the stage for future breakthroughs.
The Uncertainty Principle
One of the pillars of quantum mechanics is the Uncertainty Principle, famously articulated by Werner Heisenberg. This principle asserts that certain pairs of physical properties, particularly position and momentum, cannot be simultaneously measured with arbitrary precision. In layman's terms, the more accurately you try to pin down one property, the less certainty you have about the other.
To fully appreciate its impact, consider a scenario involving subatomic particles. If a scientist attempts to measure the position of an electron incredibly precisely, they will find that the corresponding measurement of its momentum remains vague. This is not just a limitation in measurement tools; it is a fundamental aspect of nature. The implications are vast, extending into fields like quantum cryptography and quantum computing, where certainty and predictability are key.
"Heisenberg's Uncertainty Principle acts like a cloak hiding the true nature of the microcosm, challenging everything we thought we understood about the universe."
While researchers continue to delve into this principle's consequences, it raises philosophical inquiries about determinism and the nature of reality itself. In essence, the uncertainty encapsulates a paradoxical duality; it’s both a barrier and a gateway to deeper understanding.
Decoherence and Classicality
Another significant aspect affecting quantum physics is decoherence. This phenomenon explains the transition from quantum superposition—where particles exist in multiple states—to classical behavior, where these states collapse into a single outcome. Imagine a cat in a box; in quantum terms, it's both alive and dead until someone opens the box. Decoherence can be thought of as that moment when the box is opened, and the cat's fate is revealed.
This transition represents a challenge for scientists, as they struggle to isolate quantum systems from their environments.
- Environmental Interactions: These interactions, with air, light, or thermal fluctuations, can cause a quantum system to lose its superposition, leading to classical predictability.
- Scalability Issues: As systems grow more complex and entangled, maintaining quantum states becomes progressively difficult, limiting the practical applications of quantum technology.
The implications of decoherence are profound. Understanding how and why it occurs is essential for the development of quantum computation and the realization of quantum networks capable of revolutionizing communication.
In summary, grappling with the challenges of the Uncertainty Principle and decoherence sheds light on the limitations that scientists must navigate in their pursuit of knowledge. While these elements may present challenges now, they also signal deep mysteries waiting to be unraveled in the future.
Future Directions in Quantum Physics
As we navigate further into the realm of quantum physics, it's essential to realize that we’re merely scratching the surface of its vast potential. Understanding future directions in this dynamic field requires an appreciation of not just the technology we have today, but also the innovative avenues that lie ahead. This section strives to shine a light on emerging trends and interdisciplinary approaches that promise to reshape our understanding and application of quantum mechanics.
Emerging Technologies
Emerging technologies act as the rocket fuel for the progress of quantum physics. These advancements push the boundaries of what we know and how we can apply it. Some critical areas include:
- Quantum Computing: Companies like IBM and Google are racing to build faster and more efficient quantum computers. Such machines have the potential to solve problems in seconds that would take classical computers thousands of years. Imagine cracking encryption codes or simulating molecular structures for drug development in a heartbeat!
- Quantum Sensors: These devices exploit quantum states to measure physical quantities like gravitational waves or magnetic fields with unprecedented precision. The implications of these sensors could revolutionize fields such as geology, navigation, or even healthcare.
- Quantum Networks: The pursuit of building quantum communication networks promises to improve data security. Through the principles of quantum entanglement and superposition, these networks could allow for virtually unbreakable encryption, safeguarding our online interactions.
It’s clear that as these technologies continue to advance, they will not just improve existing capabilities but also lead to entirely new domains of inquiry and application.
"The future is quantum, and it offers paths we have yet to walk."
Interdisciplinary Approaches
The increasingly complex problems that arise within quantum physics can often benefit from perspectives outside its traditional boundaries. Interdisciplinary approaches are essential in cultivating innovative solutions and advancements. Some noteworthy intersections include:
- Physics and Computer Science: The fusion of these fields is cultivating a new generation of researchers proficient in both quantum mechanics and coding, advancing quantum algorithms and computational theories, enhancing problem-solving abilities.
- Chemistry and Quantum Physics: Quantum chemistry applies quantum mechanics to chemical systems, offering new insights into reactions on a molecular level. This understanding can lead to breakthroughs in material science and nanotechnology.
- Philosophy and Quantum Mechanics: As we push the envelope of scientific understanding, philosophical inquiries into the implications of quantum mechanics—such as determinism vs. randomness—become critical for framing future directions and ethical considerations.
In summation, future directions in quantum physics are rich with promise and possibility. The technologies on the horizon and the interdisciplinary collaboration could lead us to insights previously thought unattainable, forging a deeper understanding of not just the micro realm but the universe as a whole.
The End
In the grand tapestry of quantum physics, the conclusion serves as a vital thread that weaves together the countless themes discussed throughout this work. Understanding this field isn't just an academic exercise; it’s about grasping the complexities of reality itself.
The insights into quantum mechanics provide a perspective shift for scholars, educators, and professionals alike. Each principle uncovered—from wave-particle duality to quantum entanglement—opens a window into a world that challenges our intuition and redefines our understanding of the universe.
Key Takeaways:
- Relevance: Acknowledging the vast implications of quantum theory is crucial. It affects various domains such as technology, philosophy, and fundamental science, offering a crossroad where ideas meet.
- Interconnections: The relationships drawn between concepts like decoherence and the uncertainty principle emphasize the inherent complexities within quantum phenomena, urging a deeper inquiry into their implications.
- Future Directions: As quantum research advances, the potential for innovations in fields like computing and cryptography is enormous. This provides hope and excitement for future generations of researchers.
"The greatest discoveries are often born from uncertainty; embracing it leads us towards the extraordinary."
Moreover, this conclusion spotlights the necessity for interdisciplinary approaches, as grasping the implications of quantum physics can no longer be confined to isolated realms. Instead, collaboration across various scientific and philosophical boundaries strengthens our collective understanding of nature’s secrets.
In summary, while this article has traversed numerous facets of quantum physics, the journey does not end here. Each concept poses further questions, inviting ongoing discussion and exploration. For students and professionals enthused about the potentials of quantum mechanics, initiatives are afoot to encourage greater engagement with this pivotal area of science. Through continued inquiry and dialogue, we can forge pathways toward profound discoveries, ensuring that the narrative of quantum physics evolves alongside our own understanding of the universe.