Understanding Quantum Computing: Principles and Prospects
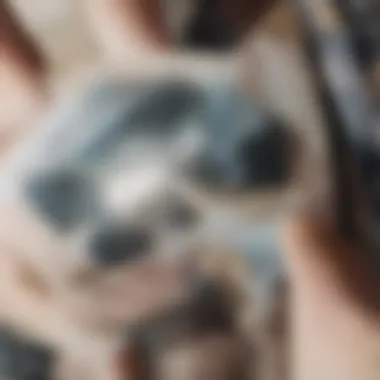
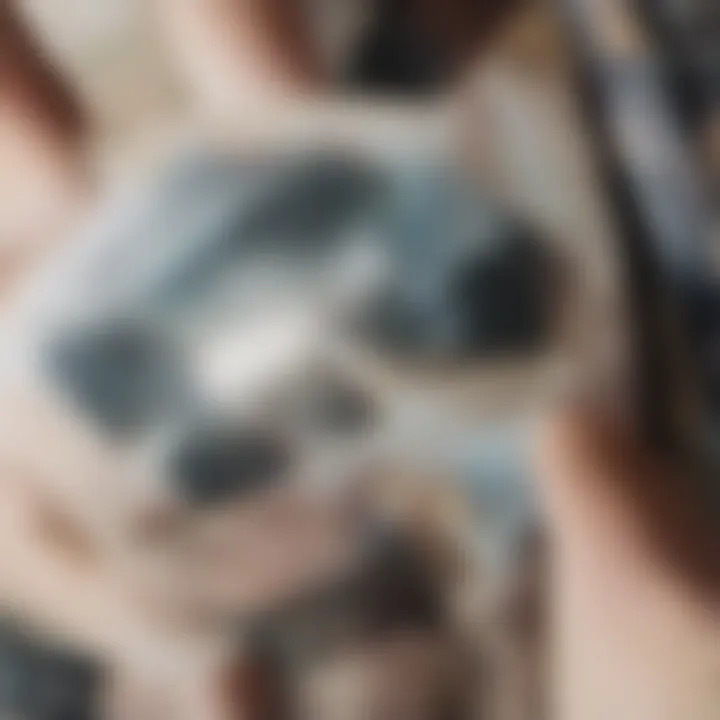
Intro
Quantum computing represents a notable departure from traditional computing paradigms. This revolutionary technology harnesses the principles of quantum mechanics, allowing for operations that far exceed the capabilities of classical computers. Unlike their classical counterparts, which use bits as the smallest unit of information, quantum computers utilize quantum bits, or qubits. This fundamental difference enables them to perform multiple calculations simultaneously, unlocking potential that could reshape various fields.
The ongoing research in the quantum computing landscape shows promising advancements. These developments could pave the way for breakthroughs in areas such as cryptography, materials science, and artificial intelligence. Given the complexity and intricate nature of quantum mechanics, a comprehensive understanding of quantum computing is essential for students, researchers, educators, and professionals.
While the theoretical framework surrounding quantum computing can seem daunting, this article aims to demystify the concepts and illustrate the relevance of quantum technologies in todayโs world. Thus, establishing a foundation of understanding is paramount as we delve deeper into this cutting-edge domain.
Prologue to Quantum Computing
The realm of quantum computing is pivotal in understanding the future of technology. This area of study is not merely a theoretical concept but a tangible field that is evolving rapidly. It focuses on the principles of quantum mechanics, offering a new approach to computation that significantly diverges from traditional methods. Quantum computing opens doors to solving complex problems that were previously inconceivable, spanning various fields like cryptography, artificial intelligence, and material science.
Defining Quantum Computing
Quantum computing can be defined as a type of computing that utilizes quantum bits, or qubits. Unlike classical bits that represent either a zero or one, qubits can exist in multiple states simultaneously thanks to the principle of superposition. This allows quantum computers to process vast amounts of information at unprecedented speeds. The shift from classical to quantum computing involves a profound change in how we perceive computational capabilities. Quantum computers function fundamentally differently by leveraging principles such as entanglement and superposition to perform calculations.
Historical Context
Early Theories
The foundations of quantum computing trace back to early theories in quantum mechanics, proposed by physicists like Richard Feynman and David Deutsch. These early theories showcased how quantum systems could simulate physical processes more effectively than traditional computers. The key characteristic of these theories was their ability to address the limitations of classical computation when it comes to modeling complex quantum phenomena. By laying the groundwork for a new computational paradigm, these theories positioned quantum computing as a viable and beneficial field of study.
The unique feature of early theories is their emphasis on the theoretical underpinnings of how quantum mechanics can be harnessed. This theoretical work has advantages such as inspiring future experiments and practical applications, but it also has disadvantages by requiring complex mathematical interpretations.
Development Milestones
As research progressed, several development milestones signified critical advancements in quantum computing. The introduction of quantum algorithms, such as Shor's algorithm and Grover's search algorithm, demonstrated the potential speed advantages quantum computers could achieve over their classical counterparts. These milestones underscore the key characteristic of quantum computingโits ability to outperform classical methods for specific problems.
The unique feature of these milestones is their application in real-world scenarios, sparking interest from both academia and industry. While they showed promise, challenges remained, particularly regarding technological implementation and error rates. This reflects an ongoing struggle between theoretical advancements and practical implementations in quantum computing.
Importance of Quantum Computing
The significance of quantum computing stretches beyond academic curiosity. It holds the potential to revolutionize numerous industries by providing solutions that are currently unattainable with classical computers. For instance, in cryptography, quantum systems can enhance security measures and develop cryptographic techniques like quantum key distribution. Applications in material science can lead to breakthroughs in drug discovery and materials development, as quantum simulations offer insights at the atomic level. Furthermore, in the field of artificial intelligence, quantum computing can facilitate faster data processing, enabling machines to learn and adapt more quickly. Understanding these implications is crucial for researchers, educators, students, and professionals as they navigate the evolving landscape of technology.
Key Concepts in Quantum Computing
Qubits
Definition of Qubits
A qubit, or quantum bit, represents the basic unit of quantum information. Unlike classical bits that can exist in a state of either 0 or 1, qubits can hold multiple states simultaneously. This is primarily due to the principle of superposition, which allows qubits to maintain a combination of both states. The ability of qubits to exist in superposition expands the computational capacity exponentially.
This characteristic makes qubits a beneficial choice for quantum computing, enabling it to perform massive calculations that would be infeasible through traditional methods. However, managing and maintaining qubits is challenging due to their delicate nature, making it essential to explore their properties carefully in this article.
Comparison with Classical Bits
In classical computing, bits represent information as discrete values: either a 0 or a 1. In contrast, qubits enhance this model significantly. An important aspect of qubits is their ability to represent both 0 and 1 at the same time, thanks to superposition. This duality allows quantum computers to analyze multiple possibilities concurrently and results in higher speed and efficiency in computation.
Superposition
Concept of Superposition
Superposition is a critical principle of quantum mechanics, signifying that a quantum system can exist in multiple states at once. For a qubit, this means it can represent not just a single value but simultaneously both values, 0 and 1. This unique feature underlies the power of quantum computing, pushing traditional boundaries of what can be calculated efficiently.
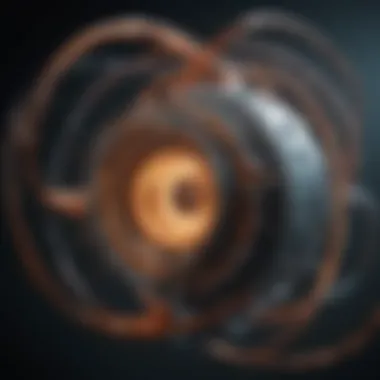
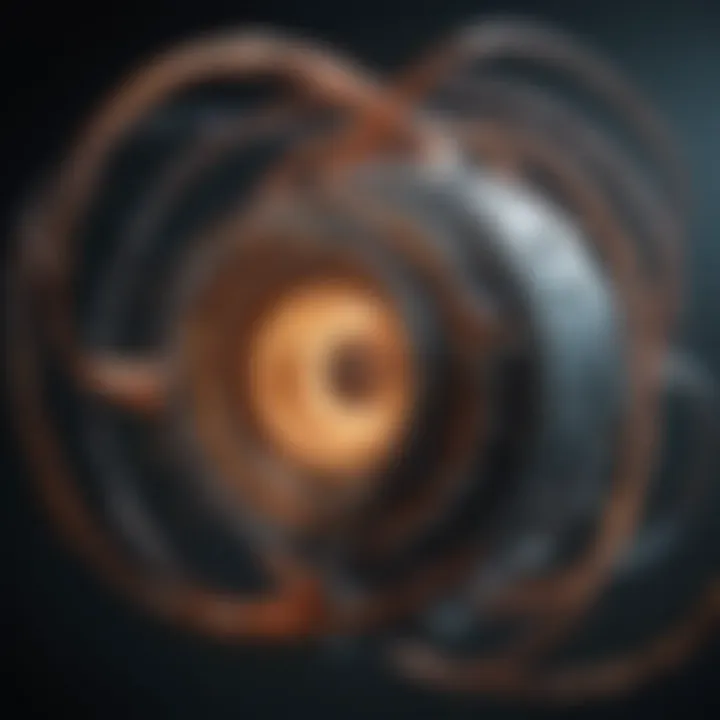
In this article, we will examine how harnessing superposition in quantum systems leads to substantial advantages over classical systems in processing and solving complex problems. However, even with its advantages, superposition presents challenges in terms of maintaining coherence in qubits, which we will address as well.
Implications for Computing
The implications of superposition for computing are profound. When a quantum system operates within superposition, it can conduct numerous computations at once, drastically reducing the time required for problem-solving. For instance, in optimization problems, rather than evaluating possibilities one by one as classical computers do, quantum computers can explore multiple paths simultaneously, unlocking a new realm of potential solutions.
Utilizing superposition has particularly beneficial effects in fields like cryptography and drug discovery. Nevertheless, the actual execution of algorithms that take advantage of superposition requires precision and effective error handling, which we will discuss further throughout this piece.
Entanglement
Understanding Quantum Entanglement
Entanglement is another cornerstone of quantum computing, wherein two or more qubits become interconnected in such a way that the state of one qubit directly affects the state of another, regardless of the distance separating them. Once entangled, measuring one qubit instantly determines the state of the other qubit. This phenomenon challenges classical intuitions about data and information flow.
Understanding entanglement adds to our grasp of how quantum computers can perform certain calculations much faster than classical ones. By leveraging this concept, quantum algorithms can effectively communicate and process information in ways that could lead to breakthroughs in various scientific fields.
Utilization in Quantum Systems
The utilization of entanglement in quantum systems manifests in various applications, particularly in quantum cryptography and quantum teleportation. In cryptography, entangled qubits enable secure communication channels that are theoretically immune to eavesdropping. Using entangled particles ensures that any attempt at interception alters the communication itself, providing a level of security unattainable in classical systems.
However, entanglement also poses challenges. It requires precise control and coherence, as disturbances can lead to loss of entanglement. This article will explore these trade-offs and their implications for the future of quantum systems.
The Mechanics of Quantum Computing
The mechanics behind quantum computing is foundational for understanding how this revolutionary technology operates. It articulates the specific methods and systems that allow quantum computers to process information differently from classical computers. By focusing on quantum gates, circuits, algorithms, and measurement principles, we can gain insight into the operational dynamics of quantum systems. This section highlights how these elements not only contribute to computational speed but also unlock unprecedented potential across various fields.
Quantum Gates and Circuits
Functionality of Quantum Gates
Quantum gates serve as the building blocks of quantum circuits, similar to how classical logic gates are used in their classical counterparts. A quantum gate manipulates an input quantum state, ensuring it evolves into a predetermined output state. The primary feature of quantum gates is their ability to exploit principles such as superposition and entanglement, which allows multiple calculations to occur simultaneously. This unique functionality makes quantum gates essential for performing complex operations that would be infeasible on classical systems.
Quantum gates enable manipulation of qubits in ways that classical bits cannot be manipulated, leading to more efficient processing.
One of the major advantages of quantum gates is their capacity to create intricate quantum circuits. This can lead to significant performance improvements in computation tasks compared to traditional methods. However, developing reliable quantum gates remains a challenge, as errors can accumulate rapidly during computations.
Circuit Construction
Constructing circuits in quantum computing involves arranging quantum gates in a specific sequence to perform desired computations. The key aspect of circuit construction is the design flexibility that allows researchers and engineers to craft circuits tailored to a variety of computational tasks. This adaptability is why circuit construction is a focal point of this article concerning quantum technology.
One unique feature of quantum circuits is that they often require fewer gates than their classical counterparts to achieve a similar outcome. This can lead to reduced energy consumption and increased processing speed. Nevertheless, constructing large and complex circuits remains an engineering challenge, especially when ensuring that they remain coherent and free from errors.
Quantum Algorithms
Famous Quantum Algorithms
Famous quantum algorithms such as Shor's algorithm and Grover's algorithm have highlighted the potential of quantum computing to solve problems markedly faster than classical methods. Shor's algorithm, for instance, can factor large numbers exponentially faster than the best-known classical algorithms. This characteristic underlines the relevance of these algorithms in fields like cryptography, where traditional security measures can be compromised.
The popularity of these algorithms stems from their ability to leverage quantum systems for significant speedups in processing tasks. Yet, the complexity of implementing these algorithms in practical applications raises questions and ongoing research focused on their effectiveness and real-world feasibility.
Algorithm Efficiency
Algorithm efficiency in quantum computing pertains to how well these algorithms utilize quantum resources to outperform their classical counterparts. The significant factor is the algorithm's scaling; while some algorithms show exponential speedups, others may offer diminishing returns depending on the problem complexity. Hence, assessing algorithm efficiency contributes critical insights into the overall capabilities of quantum systems.
A unique aspect of algorithm efficiency is the need for optimal quantum circuit design to maximize performance. While quantum algorithms are promising, they still face limitations in terms of robustness and the influence of noise, making this an active area of research.
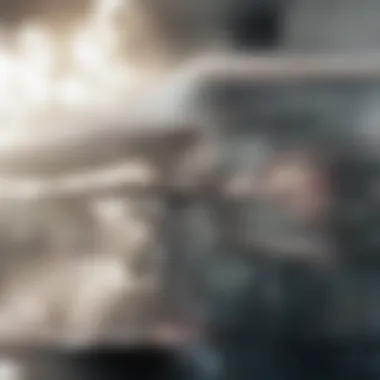
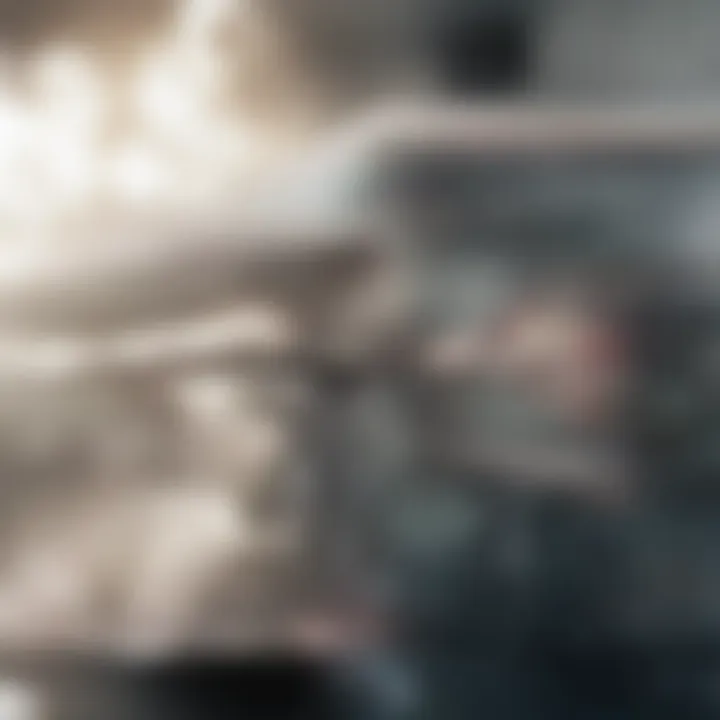
Measurement in Quantum Computing
Quantum Measurement Principles
Quantum measurement principles describe how information is extracted from quantum systems. Measurement collapses the quantum state into a definite outcome, a fundamental aspect differing significantly from classical measurement concepts. The core feature of quantum measurement is the probabilistic nature of outcomes, which emphasizes the non-deterministic character of quantum mechanics.
Understanding quantum measurement is crucial because it affects how data from quantum computers is interpreted. Notably, this process can introduce challenges, particularly if the measurements are not managed properly, leading to errors in computation.
Challenges in Measurement
Challenges in measurement revolve around the unpredictability that quantum systems exhibit during observation. Despite the theoretical understanding, there are practical issues related to achieving accurate and reliable measurements in real settings. Researchers face the dilemma of noise interference, which can skew results and compromise the integrity of computations.
The necessity of advanced techniques and technologies to improve measurement reliability makes this a vital discussion point. Addressing these challenges is essential for advancing practical applications of quantum computing, ensuring that the promise of quantum systems can be fully realized.
Applications of Quantum Computing
Quantum computing has extensive potential applications across various fields, reshaping our understanding and capabilities in numerous domains. Its significance lies not only in theoretical advancements but also in its practical implications. As industries explore quantum technologies, they seek ways to leverage its distinctive characteristics, such as qubit manipulation, superposition, and entanglement. This section delves into three prominent areas where quantum computing could make transformative changes: cryptography, material science, and artificial intelligence.
Cryptography
Impact on Current Cryptographic Systems
The rise of quantum computing introduces both challenges and opportunities for traditional cryptographic systems. Current cryptographic algorithms like RSA and ECC rely heavily on the difficulty of certain mathematical problems. However, quantum computers could efficiently solve these problems, rendering current encryption methods obsolete. This potential vulnerability is a significant aspect of modern cryptography. The shift from classical to quantum cryptosystems reflects the urgent need for new security protocols that can withstand quantum attacks. Thus, the impact on current systems is profound, requiring a proactive approach to security.
Quantum Cryptography Techniques
Quantum cryptography encompasses principles that promise secure communication channels, even in the face of quantum computing advancements. A notable technique is Quantum Key Distribution (QKD), which uses quantum mechanics to transmit encryption keys securely. The key characteristic of QKD is its ability to detect eavesdropping through quantum states, making it an essential choice for secure communications. This unique feature of ensuring secure key exchange leads to potential advantages, such as enhanced security protocols. However, practical implementation faces challenges in terms of distance and infrastructure.
Material Science
Simulations of Quantum Systems
In material science, simulations of quantum systems facilitate the understanding of complex materials and phenomena at the atomic level. Quantum computers can model interactions that classical computers find computationally expensive or unfeasible. The ability to simulate quantum systems accurately enables researchers to predict material properties and behaviors in ways that were previously out of reach. This essential characteristic makes quantum simulations a critical tool in advancing material science. Nevertheless, the development of effective simulation algorithms is still an ongoing research area.
Discoveries Enabled by Quantum Computing
Quantum computing holds potential for significant breakthroughs in discovering new materials with unique properties. By analyzing complex interactions within quantum systems, researchers can unlock new avenues for innovation. This characteristic supports the goal of creating more efficient materials for batteries, catalysts, and other technologies. Furthermore, these discoveries could lead to advances across various industries, from energy to electronics. However, integrating quantum computing with experimental science requires comprehensive collaboration and exploration.
Artificial Intelligence
Quantum Machine Learning
Quantum machine learning integrates quantum computing principles with machine learning algorithms. This combination enables faster processing of vast data sets, offering unprecedented capabilities for pattern recognition and predictive analysis. The essential feature of quantum machine learning is its potential to enhance the speed and accuracy of finding solutions to complex problems. As industries increasingly rely on data-driven insights, this application of quantum computing proves beneficial for improving decision-making processes. However, this field is still in its infancy, requiring extensive research and validation.
Benefits Over Classical Approaches
When comparing quantum machine learning with classical approaches, the potential benefits become evident. Quantum algorithms can outperform classical algorithms for specific tasks, offering speedups that are significant in large data environments. This advantage relates directly to the ability of quantum computers to process and analyze information differently, leveraging their unique properties. Despite the potential benefits, the practical implementation of quantum machine learning faces challenges in terms of algorithm development and hardware limitations.
As the field of quantum computing progresses, its applications will continue to evolve, impacting diverse sectors significantly.
Challenges in Quantum Computing
The realm of quantum computing is not without its obstacles. Understanding these challenges is crucial. They shape the development and practical application of this technology. The main areas of concern include error rates, scalability, and technological barriers. Each of these elements plays a pivotal role in determining the future of quantum computing. Addressing these challenges is essential for harnessing the full potential of quantum systems.
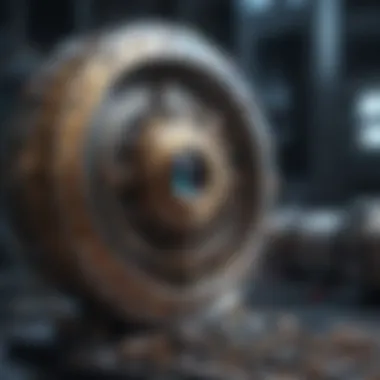
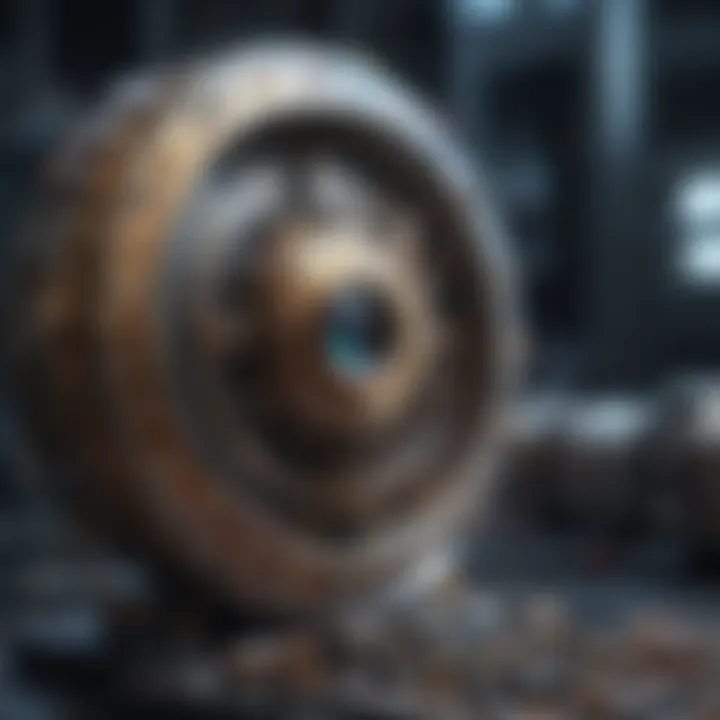
Error Rates
Sources of Errors in Quantum Systems
Quantum systems are inherently fragile. They are susceptible to various sources of errors, primarily due to their reliance on quantum superposition and entanglement. Environmental factors like temperature fluctuations and electromagnetic interference can disrupt quantum states. These disturbances can cause decoherence, leading to loss of information. Understanding these sources is vital for improving the reliability of quantum computations. A key characteristic of error sources is their unpredictable nature, making error management a significant concern in the field. If left unaddressed, these errors can undermine the advantages that quantum computing offers.
Error Correction Techniques
To combat errors, researchers have developed various error correction techniques. Quantum error correction is a fundamental aspect of ensuring computational reliability. It involves encoding information in a way that allows for the reconstruction of lost data. One notable method is the surface code, which is efficient in protecting qubits against errors. The unique feature of error correction techniques is their ability to maintain quantum information despite physical disturbances. Implementing these techniques can be resource-intensive. However, their benefits in enhancing system reliability cannot be overlooked. As quantum technology advances, these correction strategies will need constant refinement.
Scalability
Current Limitations
Scalability is another significant challenge in quantum computing. Current quantum systems are limited in the number of qubits they can effectively manage. The complexity of entangling multiple qubits grows exponentially with the number of particles involved. This limitation affects the potential for building larger, more capable quantum computers. One of the key characteristics of these current limitations is their impact on computational capability. Scalability remains a hot topic within the community, as it is directly linked to the power of quantum processors. It is clear that overcoming these limitations is paramount for future success.
Future Prospects
Looking forward, there are promising developments in quantum computing scalability. Researchers are exploring various architectures, such as trapped ions and superconducting circuits, to enhance system performance. The potential integration of quantum systems with classical computing could be a game-changer. A noteworthy feature of these prospects is the idea of hybrid systems that use both quantum and classical resources effectively. However, fostering such innovations will require significant investment and collaboration across disciplines. The advancement of scalable quantum computing is critical for addressing real-world problems where classical systems fall short.
Technological Barriers
Hardware and Software Challenges
Quantum computing faces considerable technological barriers. One main challenge lies in the hardware necessary to support quantum operations. Building stable qubits and maintaining the conditions for their operation is difficult. Similarly, the software designed to run on quantum systems is still in its infancy. The integration of hardware and software poses a unique characteristic that needs overcoming. The lack of mature tools for programming quantum computers is a notable disadvantage. Yet, developments in this area promise a rich avenue for innovation.
Investment in Research
Investment in research is essential for breaking down technological barriers. Increased funding can accelerate the pace of discovery in quantum technologies. Governments and private sectors are recognizing the importance of quantum research and are beginning to allocate significant resources. An important feature of heightened investment is the interdisciplinary nature of quantum computing. Bridging gaps between physics, engineering, and computer science creates a more robust research landscape. While the need for funding is clear, aligning these resources with specific research goals remains a complex task.
The Future of Quantum Computing
The landscape of quantum computing is poised for significant transformations in the coming years. As technological advancements intersect with increasing investments, the future holds great promise for realizing the potential of quantum systems. This section scrutinizes current research trends, the collaborative spirit among various disciplines, and the societal implications of quantum technologies.
Research and Development Trends
Research in quantum computing continues to evolve rapidly. Innovations span areas such as quantum hardware, algorithms, and error correction techniques. Quantum processors are becoming more sophisticated, promising enhanced qubit fidelity and coherence times. Several leading companies and academic institutes, such as IBM and Google, have committed substantial resources towards developing quantum supremacy and achieving practical quantum advantage.
Funding in quantum research has been escalating as governments recognize its strategic importance. National initiatives in countries such as the United States and China are evident. These efforts aim not only to advance technology but also to attract and retain top talent in the field. Moreover, breakthroughs in materials science are also significant, contributing to constructing more reliable quantum hardware.
Interdisciplinary Collaborations
Quantum computing is not a solitary domain; it thrives on interdisciplinary collaborations. Partnerships between physicists, computer scientists, engineers, and even domain experts in biology and finance are becoming commonplace. These collaborations help foster a broader understanding of the multifaceted challenges presented by quantum technologies.
For instance, efforts in quantum machine learning benefit greatly from input by data scientists who understand classical algorithms' limitations. Additionally, specialists in cryptography contribute knowledge on securing quantum communications, while physicists provide insights into quantum mechanics principles. Such collaborative dynamics push the forefront of quantum computing research, presenting more innovative solutions to existing problems.
Prospective Societal Impacts
Changes in Industries
One significant aspect of quantum computing's future is its ability to transform industries. Industries like finance, energy, and pharmaceuticals stand to gain substantially from quantum technologies. The key characteristic of these changes is efficiency. Quantum algorithms can process large data sets much quicker than classical counterparts. For example, complex financial modelling that takes days on classical systems could reduce to hours or even minutes. Not only does this save time, but it also leads to cost benefits and more informed decision-making.
However, the shift is not without its challenges. Existing workforce skills may not align with quantum computing needs. An emphasis on training will be necessary to bridge that gap. The unique feature of these industry changes is a substantial drive towards not just competitive advantage but also innovations in products and services, enhancing overall consumer experience.
Influence on Everyday Life
In considering the influence on everyday life, quantum computing may redefine what is achievable in daily technology. An important characteristic of this influence is the democratization of technology. Enhanced computational power can lead to smarter applications in healthcare, daily logistics, and personal technology. For instance, rapid drug discovery could become commonplace, resulting in improved health outcomes and cost reductions in healthcare.
Yet, the accessibility of quantum technologies also poses a dilemma. Ensuring that all sectors of society benefit equally remains a crucial concern. The unique aspect of this influence is its potential to eradicate previous technological barriers, but it may also widen the gap between those with access to advanced technologies and those without. Balancing innovation with social equity will be essential in shaping the future trajectory of quantum computing.
The rapid evolution of quantum computing brings not merely technological advances but also profound societal implications that must be managed responsibly.