Understanding Particle Entanglement in Quantum Physics
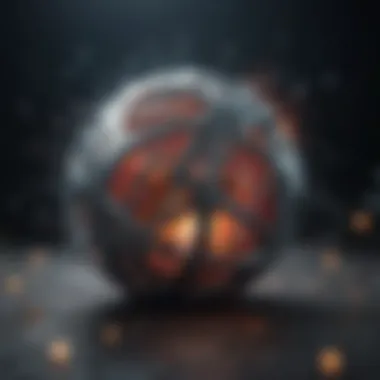
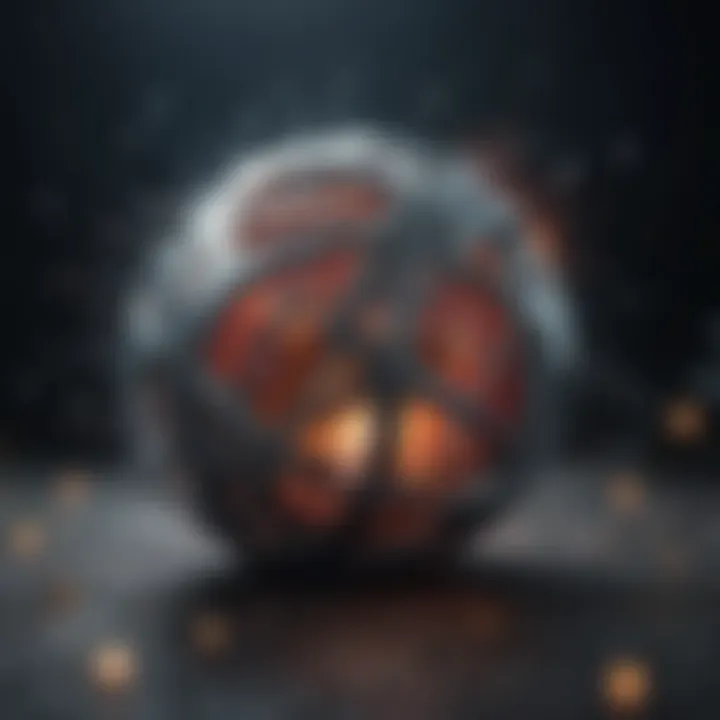
Intro
Particle entanglement is a puzzle that has fascinated physicists for decades. This phenomenon is central to quantum mechanics and raises questions about the nature of reality itself. When two particles become entangled, their states become interdependent, no matter the distance between them. This leads to implications that challenge our understanding of classical physics.
In this article, we will explore the intricate details of particle entanglement. From examining key research findings to breaking down complex theories, the aim is to provide a comprehensive overview for students, researchers, and professionals. As we unravel these topics, we will highlight how entanglement not only impacts quantum computing but also reshapes the way we perceive the universe.
Prologue to Particle Entanglement
Particle entanglement stands as a pivotal concept in quantum physics, embodying the intricate relationship between particles that transcends classical boundaries. Understanding this phenomenon is crucial for deciphering the fundamentals of quantum mechanics. The significance of entanglement extends beyond mere theoretical interest; it triggers revolutionary advancements in technology and philosophical thought.
This section aims to elucidate the essence of entanglement, its historical backdrop, and its implications. The exploration of this topic introduces fundamental principles that govern the quantum realm, providing insights essential for students, researchers, and educators.
Through a rigorous examination of particle entanglement, one uncovers the layers that contribute to its complex nature. The dialogue surrounding entanglement prompts inquiries into the nature of reality itself, instigating various interpretations of quantum mechanics. This nuanced understanding influences emerging technologies in quantum computing and cryptography, granting practical advantages over classical approaches.
As we journey into the depths of this subject, we will first establish a clear definition of entanglement, followed by a historical context that highlights its evolution from ancient theories to contemporary discussions. This structured narrative not only informs but also prompts critical thinking regarding the implications of entangled states on both technology and philosophy.
Theoretical Foundations of Particle Entanglement
Understanding the theoretical foundations of particle entanglement is crucial for deeper insights into quantum physics. This section lays the groundwork for grasping how entangled particles interact in ways that defy classical mechanics. The principles discussed here are vital in various applications such as quantum computing, secure communication, and even philosophical explorations into the nature of reality.
Quantum States and Superposition
At the core of quantum mechanics lies the concept of quantum states. A quantum state describes the properties of a particle at a given moment. Superposition is a fundamental principle, allowing particles to exist in multiple states simultaneously until measured. For instance, an electron can be both spinning up and spinning down until a measurement forces it into one state. This duality is crucial for understanding entangled states, as it reveals the underlying complexity of particle relationships.
Any entangled particles demonstrate superposition, enabling them to affect one another instantaneously regardless of the distance separating them. Understanding these concepts aids researchers in leveraging quantum mechanics for technological advancements.
Entangled States Explained
Entangled states are a special kind of quantum state where the properties of two or more particles become interlinked, meaning the state of one particle cannot be described independently of the state of the other. This relationship persists even if the particles are physically separated.
The notion of entanglement challenges classical intuitions about locality and separability. For example, if one particle is measured and its state is found, the second particle's state will change instantly, regardless of the distance. This non-locality raises intriguing questions about the fundamental nature of reality. The implications are not just theoretical; they also fuel practical applications, particularly in fields such as quantum computing and cryptography.
Mathematical Representation
Mathematics provides the tools necessary to describe and quantify quantum states and their entanglement.
Density Matrices
Density matrices offer a way to represent quantum states, especially when dealing with mixed statesโsituations where there is uncertainty about which state a system is in. A density matrix encapsulates all the statistical properties of a quantum system, effectively summarizing the information available about it.
One key characteristic of density matrices is their ability to represent entangled states compactly. This makes them a beneficial choice for analysis in entanglement research. A unique feature of density matrices is their trace, which sums the diagonal elements and equals one, ensuring normalization of probabilities.
However, density matrices can be complex to manipulate mathematically, posing challenges with larger systems. Despite this, their advantages in describing entangled systems make them an indispensable part of quantum theory.
Bell States
Bell states are particular examples of maximally entangled quantum states. They are named after physicist John Bell, who formulated a way to explore the nature of entanglement through thought experiments. A key characteristic of Bell states is that they exhibit perfect correlations in measurement outcomes.
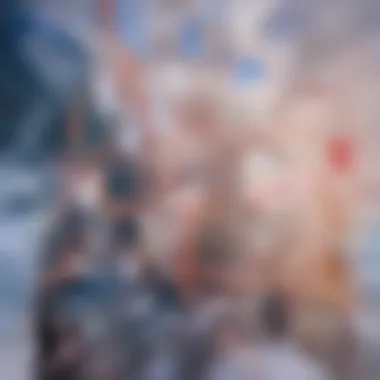
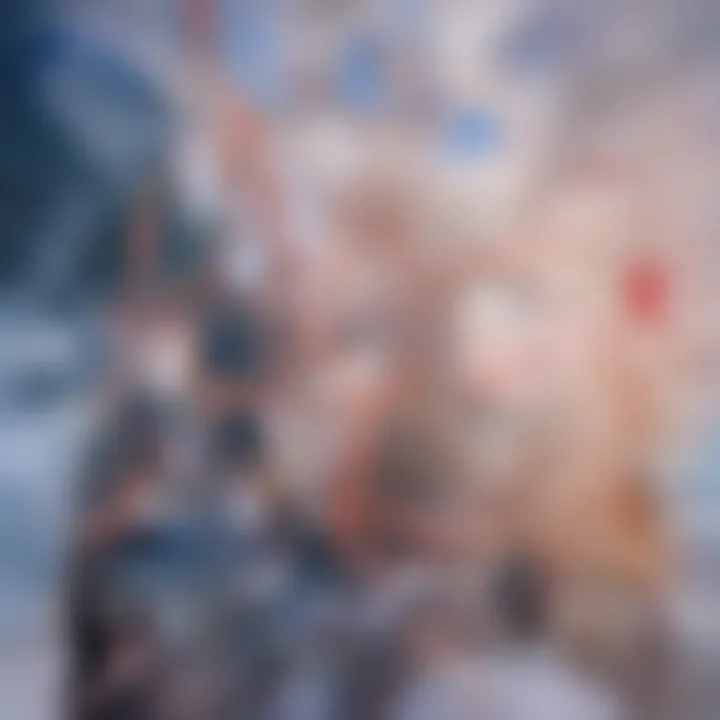
This feature makes Bell states a popular choice for illustrating the principles of entanglement in both theoretical and experimental contexts. The unique aspect of Bell states is their ability to be transformed into one another through local operations and classical communication, which is essential for quantum communication protocols.
However, working with Bell states may introduce challenges in scaling to more complex systems with multiple entangled particles. Nonetheless, they remain a powerful concept within quantum physics, showcasing the intricate nature of particle entanglement.
Key Experiments in Particle Entanglement
Understanding the key experiments in particle entanglement is crucial for grasping its role in quantum mechanics. These experiments not only validate theories but also reveal the profound implications of entangled states on reality and technology. They serve as a bridge between abstract theoretical concepts and empirical validation, illuminating the strange interplay of quantum particles.
Each major experiment contributes distinct insights that enrich our comprehension of entangled particles. Notably, they demonstrate how entanglement can be measured, manipulated, and harnessed for applications in various fields, including computing and secure communication. Thus, engaging with these key experiments provides a deeper appreciation of quantum mechanics and its revolutionary potential.
Aspect's Experiment
Aspect's Experiment, conducted by Alain Aspect in the 1980s, is a landmark study that tested the predictions of quantum mechanics against classical local realism. This experiment involved measuring the polarization states of pairs of entangled photons, showing that the results were consistent with quantum mechanical predictions.
Aspect's work reaffirmed the existence of non-local correlations and challenged the intuitive notion of separability between entangled particles. This experiment highlighted that measuring one particle instantly affects the state of its partner, regardless of the distance separating them. Aspect's Experiment has profound implications for our understanding of reality, suggesting it does not adhere to classical intuitions.
Entanglement in Quantum Cryptography
Entanglement is also crucial for quantum cryptography, which leverages the principles of quantum mechanics to secure communication. Quantum Key Distribution (QKD) is a major application that showcases the benefits of entanglement in creating secure keys for encryption.
Quantum Key Distribution
Quantum Key Distribution enables two parties to share a secret key with a guarantee of security. This is accomplished through entangled particles. One of the key characteristics of QKD is its ability to detect eavesdropping. If a third party tries to intercept the key exchange, the entangled state changes, alerting the legitimate users. This unique feature makes QKD a beneficial choice for secure communications, as it leverages the inherent unpredictability of quantum mechanics.
However, the challenges include the need for advanced technology to maintain entanglement over long distances. There can be losses in signal quality, which can complicate secure transfers. But recent progress in repeating methods and improved technology shows potential for QKD to become more widely accessible.
Recent Developments
Research in entanglement is rapidly evolving, leading to significant discoveries and technological advancements.
Experimental Confirmation
Experimental confirmation of particle entanglement further supports theoretical claims. Repeatable experiments and improved measurement techniques have produced solid evidences of entanglement phenomena.
The key characteristic of these confirmations lies in their ability to dismantle classical assumptions about locality. As various studies confirm quantum predictions, this integration emphasizing experimental data makes the findings robust. Advances in experimental methods enhance the resolution of measurements and tightens agreement between theory and observation. However, challenges remain in replicating conditions for reliable results.
Technological Advances
Technological advances in quantum entanglement are reshaping how we approach both foundational physics and practical applications. Innovations in qubit technology allow for more efficient entanglement processes, which are vital for quantum computing.
The key characteristic of these advances is their ability to expand the scope of entanglement into areas such as quantum networks for secure communication. The unique feature of this technology is that it not only enhances the capabilities of quantum processors but also increases stability and scalability in real-world applications. Nonetheless, the complexities of quantum systems remain, requiring careful management and understanding of decoherence.
Applications of Particle Entanglement
Particle entanglement has become a foundational concept in many modern scientific and technological initiatives. It not only helps bridge gaps in our understanding of quantum mechanics but also offers tangible benefits in sectors such as computing, secure communication, and beyond. As this article discusses various applications, it will be evident that entanglement plays a pivotal role in shaping future technologies while challenging our perceptions of connectivity and interaction.
Quantum Computing
Qubits and Entanglement
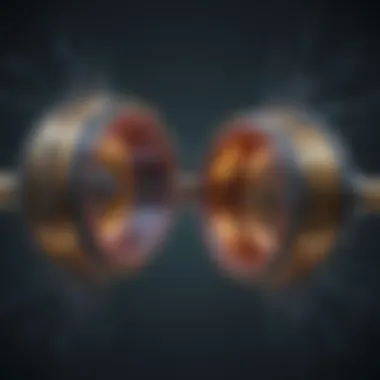
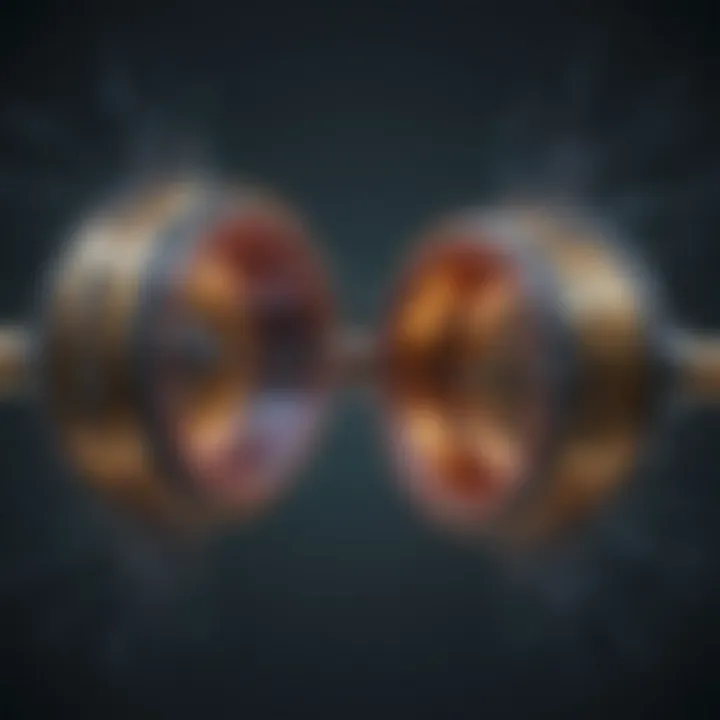
Qubits, or quantum bits, represent the basic unit of information in quantum computing. Unlike classical bits, which take the form of either 0 or 1, qubits can exist in a state of superposition due to entanglement. This property allows qubits to perform multiple calculations simultaneously, making them highly efficient for complex problem-solving tasks.
This trick of entanglement enhances computation speed and ability. There is, however, a trade-off in stability; manipulating qubits requires precise conditions to maintain coherence. If not handled well, the superposition state collapses, leading to potential errors in calculations. Nonetheless, harnessing this feature is essential for future advancements in algorithm speed and optimization.
Processing Power
Processing power in quantum computing is fundamentally linked to the concept of entanglement. When qubits are entangled, the state of one qubit becomes dependent on the state of another, regardless of the spatial separation between them. This characteristic leads to an exponential increase in computing power as the number of entangled qubits rises.
The key attraction of harnessing this ability is in tasks that involve large data processing or simulations. Quantum computing could outperform traditional supercomputers by executing vastly complex computations in a fraction of the time. The disadvantage, however, remains the challenge of error correction and the need for operating in low-temperature environments which may hinder accessibility.
Quantum Teleportation
Quantum teleportation uses entanglement to transfer quantum information between particles instantaneously across distances. It does not involve the physical transport of particles but rather the transfer of their states. This phenomenon has profound implications for quantum networks, allowing for secure transfer of information without loss of fidelity. Understanding this process contributes to advancements in communication technology and could redefine protocols in data transmission.
Cryptography
Secure Communication
Secure communication is another crucial area where particle entanglement demonstrates its potential. The principles of quantum key distribution, which rely on entanglement, ensure that communication between parties remains confidential. Intercepting the information changes the outcome due to the nature of quantum mechanics; any eavesdropping can be detected. This unique feature elevates the security factor in digital communications, making it a powerful tool for sensitive transactions.
Future Prospects
The future prospects of quantum cryptography appear significantly dependent on entangled states. Continued research can unveil more refined methods for securing data. The development of more sophisticated entanglement-based communication systems could lead to a new standard for digital privacy. Each step forward in this area opens doors to more complex networks that could be essential in a world increasingly reliant on secure electronic communications.
"Quantum entanglement could be the key to revolutionizing information security, redefining how data is shared globally."
As we explore these varied applications, it becomes clear that particle entanglement is much more than a theoretical concept; it is a transformative force with real-world implications across numerous disciplines.
Philosophical Implications of Entanglement
The exploration of particle entanglement encompasses not only scientific concepts but also deep philosophical questions. It challenges our fundamental understanding of reality, locality, and the nature of consciousness. The implication of entanglement on philosophical inquiries allows us to reconsider what we know about the universe. Discussions around entanglement elicit questions of causality and connection in ways that are fundamentally incompatible with classical physics. Hence, it serves as an essential topic in broader discourses about the nature of existence and knowledge.
Non-locality and Reality
Einstein-Podolsky-Rosen Paradox
The Einstein-Podolsky-Rosen, or EPR, paradox provoked significant discussion about the nature of reality and locality in quantum physics. In their 1935 paper, Albert Einstein, Boris Podolsky, and Nathan Rosen devised a thought experiment highlighting how entangled particles could exhibit correlations that defy classical logic. They posited that either quantum mechanics is incomplete, or that distant particles can instantaneously affect each other, known as non-locality. This paradox illustrates a critical tension between quantum mechanics and relativistic norms implying that information cannot travel faster than the speed of light.
The EPR paradox is beneficial to this article because it showcases a fundamental challenge to our understanding of reality. It emphasizes conflicting notions of information transfer and locality as the teaching of classical physics would suggest. The unique feature of this paradox lies in its ability to provoke debates regarding the interpretation of quantum mechanics, raising questions about what it really means for two particles to be entangled, and whether our perception of reality is fundamentally flawed.
Interpretations of Quantum Mechanics
Copenhagen Interpretation
The Copenhagen Interpretation offers a way to understand the peculiarities of quantum behavior, particularly regarding measurement and observation. According to this framework, quantum systems exist in a superposition of states until measured. When an observation occurs, the wavefunction collapses into a definite state. This interpretation emphasizes the role of observers in defining physical reality, suggesting that without observation, particles do not have definitive states.
Copenhagen's perspective is widely accepted among physicists. It benefits this article by providing a fundamental viewpoint on the interpretation of quantum physics. The interpretation's unique feature is its assertion that reality is not determined independently of observation. A possible disadvantage, however, is its reliance on the observer, leading to philosophical dilemmas about objectivity in science.
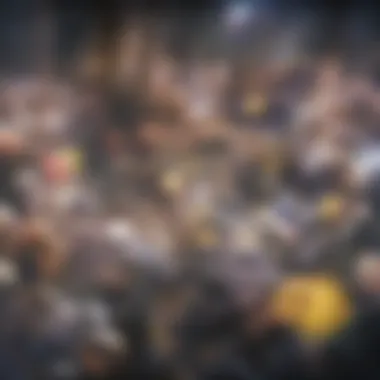
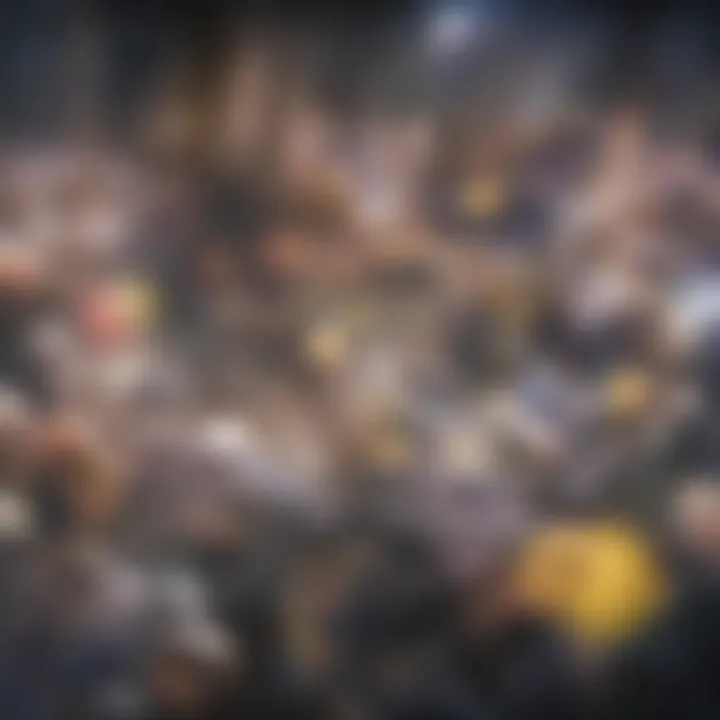
Many-Worlds Interpretation
The Many-Worlds Interpretation proposes an alternative view, asserting that all possible outcomes of quantum measurements exist in separate, branching universes. Each measurement produces a split in the universe, where each possible result is realized in its timeline. It thus eliminates randomness by maintaining that every possibility is a part of reality.
This interpretation is beneficial for the discourse surrounding quantum mechanics within this article as it challenges traditional notions of singular outcomes and encourages exploration into the nature of existence. The unique feature of the Many-Worlds Interpretation lies in its embrace of determinism by suggesting that everything happens at once, in parallel realities. A downside could be the difficulty in experimentally validating these branches or universes, leading to debates about its practical implications in real-world applications.
The examination of these interpretations deepens our understanding of entanglementโs implications for the nature of reality and perception, emphasizing quantum physics's transformative impact on philosophy.
Future Directions in Entanglement Research
The future of entanglement research is rich with possibilities. As quantum technology continues to evolve, understanding particle entanglement becomes even more crucial. This section focuses on new and emerging areas that promise to expand our grasp of quantum interactions. The significance of these directions lies not only in technological advancements but also in their potential to bridge gaps in our current understanding of quantum mechanics.
Emerging Technologies
Quantum Networks
Quantum networks represent a significant leap in the field of quantum communication. These networks are designed to enable secure, high-speed data transmission using the principles of quantum entanglement. One key characteristic of quantum networks is their ability to create entangled states between distant particles. This property guarantees that any change to one particle affects its entangled partner, regardless of the distance separating them. As a result, quantum networks emerge as a popular choice for developing secure communication systems.
However, one unique feature to consider is their dependence on a stable environment for maintaining entangled states. External disturbances can lead to decoherence and loss of information, making network reliability a challenge.
Advancements in Qubit Technology
Advancements in qubit technology are foundational for the progress of quantum computing. Qubits serve as the basic units of information in quantum systems, and their ability to exist in superpositions is essential for quantum algorithms. One notable aspect of recent developments is the enhancement of qubit coherence times. A significant improvement in coherence allows for more complex computations over extended periods. This is beneficial for the realm of quantum information processing.
Still, it's important to mention that integrating qubits into larger systems remains complex. The challenge of scaling up while preserving their quantum nature is a hurdle that researchers must overcome.
Potential Discoveries
Testing the Limits of Entanglement
Testing the limits of entanglement could lead to groundbreaking discoveries in understanding the fundamental nature of quantum phenomena. This area focuses on exploring the constraints of entangled particle behavior under varying conditions. A key characteristic of this research is its experimental nature, as it often involves rigorous testing of quantum theories. This approach is a valuable tool for physicists seeking to refine the principles of quantum mechanics.
Nonetheless, one unique aspect is that while experiments yield insights, they may also challenge existing theories. Discrepancies between predictions and experimental results can lead to paradigm shifts in our understanding of quantum mechanics.
Exploring Quantum Gravity
Exploring quantum gravity seeks to combine the principles of quantum mechanics with general relativity. This line of inquiry is vital for understanding how gravity behaves at quantum scales. One significant characteristic is the investigation into unifying quantum physics and gravitational theory. This endeavor could answer pressing questions about the universe's fundamental structure.
The unique feature of this exploration lies in its interdisciplinary approach, merging physics with mathematics, and cosmology. A challenge remains, however, in the form of theoretical frameworks that are sometimes difficult to test experimentally. Theoretical advances often appear far ahead of empirical verification, creating a gap in immediate practical applications.
"Emerging technologies and potential discoveries represent the forefront of quantum research, driving us toward a more profound understanding of the universe."
In summary, these future directions in entanglement research open new avenues for exploration. They provide essential frameworks for understanding quantum interactions that transcend classical perspectives.
Ending
The topic of particle entanglement holds significant importance in the context of quantum physics. Understanding this phenomenon not only illuminates the intricate nature of reality but also shapes future advancements in technology and science. Particle entanglement challenges conventional perspectives, suggesting a level of connectivity between particles that transcends classical descriptions. By examining how entangled particles relate, scientists can uncover aspects of quantum behavior that have both practical and philosophical implications.
Summary of Insights
In summarizing the insights gained from the exploration of particle entanglement, it is evident that this concept is central to grasping quantum mechanics as a whole. Key points include:
- Particle entanglement reveals deep correlations between particles regardless of distance.
- It plays a crucial role in developing quantum computing and secure communication through quantum key distribution.
- Philosophical implications arise from entanglement, particularly in discussions about non-locality and the interpretation of reality.
These insights build on a foundation that enriches the understanding of particle interactions and their consequences for technology and theoretical physics.
The Ongoing Journey of Quantum Understanding
The field of quantum physics is dynamic, and the study of particle entanglement continues to evolve. Key aspects of this ongoing journey include:
- Emerging discoveries: Scientists are constantly finding new ways to test the limits of entanglement, which could lead to revolutionary applications.
- Interdisciplinary connections: The principles of entanglement influence various fields, such as information theory, cryptography, and materials science.
- Philosophical dialogues: As new findings emerge, discussions surrounding the meaning and implications of entanglement persist, prompting re-evaluation of existing theories.
The journey toward a deeper understanding of particle entanglement not only advances the field of quantum physics but also enriches our comprehension of nature itself.