Understanding the Nature and Significance of Waves
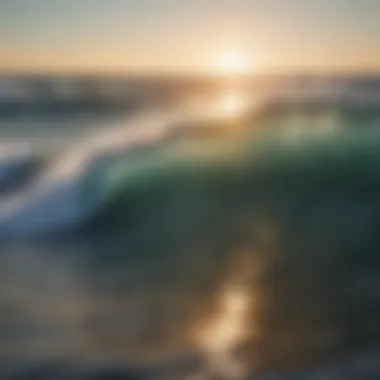
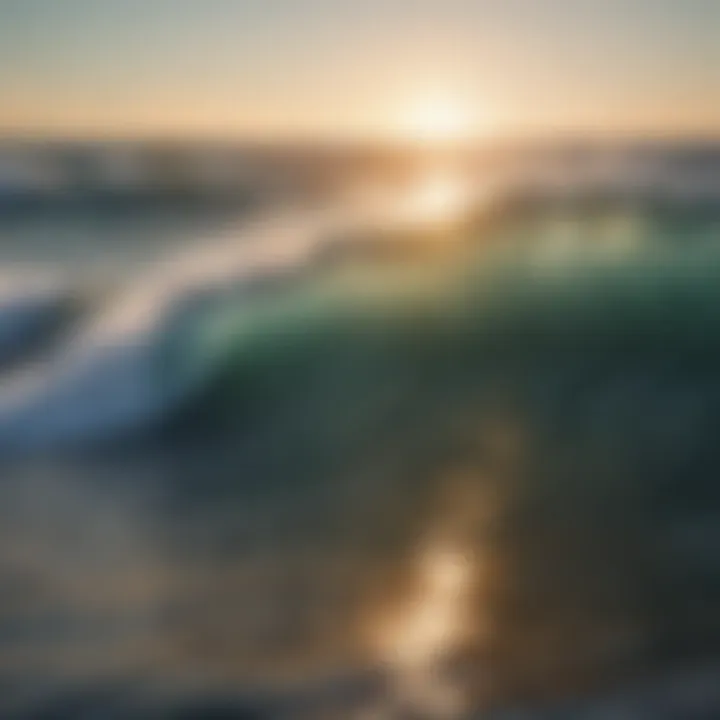
Key Research Findings
Overview of Recent Discoveries
Recent advancements in wave research have provided valuable insights into the nature of waves across various disciplines. Innovations in technology have enabled scientists to observe and analyze wave behavior with unprecedented precision. For instance, researchers have made significant strides in understanding gravitational waves, leading to a deeper comprehension of the universe and its formation. These findings impact not only astrophysics but also contribute to fields like quantum mechanics and signal processing.
In acoustics, breakthroughs in sound wave manipulation have allowed for more effective noise reduction techniques. This not only benefits engineering applications but also enhances the quality of life by improving sound environments in urban settings.
Furthermore, advances in optical wave research have propelled innovations in fiber optics, further extending the reach and capability of telecommunications. These discoveries highlight the omnipresent role of waves in our daily lives and their significance in modern science.
Significance of Findings in the Field
The implications of these recent discoveries extend beyond theoretical knowledge. The understanding of wave mechanics is crucial for various applications, such as medical imaging, wireless technologies, and environmental monitoring.
For professionals and researchers, staying abreast of these developments facilitates the integration of new techniques into existing frameworks. Such integration fosters innovation and allows for the continuation of research projects, enhancing academic and practical applications in diverse areas, including education and engineering.
"Waves are not just phenomena to study; they are tools that shape our understanding of reality and exist in every corner of science."
Breakdown of Complex Concepts
Simplification of Advanced Theories
Understanding waves often involves grappling with complex theories and principles. One notable example is the wave-particle duality, which describes how particles exhibit properties of both waves and particles. By utilizing straightforward language and consistent definitions, educators can better convey these concepts to learners. Simplifying terms like frequency, amplitude, and wavelength facilitates improved comprehension of fundamental principles.
Moreover, visual aids play a vital role in this simplification process. Diagrams that illustrate waveforms can demystify otherwise abstract concepts. Graphs showing the relationship between frequency and wavelength are especially helpful for visual learners.
Visual Aids and Infographics
Visual elements can enhance learning significantly. Infographics can summarize key aspects of wave behavior effectively. For instance, an infographic depicting types of waves, such as transverse and longitudinal, can clarify distinctions for readers.
Visual representations allow readers of varied backgrounds to grasp intricate topics more readily. As a result, utilizing these resources in educational settings or professional presentations can cultivate a deeper understanding of wave phenomena. Incorporating links to additional resources, like those found on Wikipedia or Britannica, can provide readers with further context and detailed exploration of specific concepts.
In summary, waves are a foundational aspect of various scientific disciplines. By synthesizing current research findings and breaking down complex theories, this article aims to bridge the gap between advanced understanding and practical application for students, researchers, and professionals alike.
Prelims to Waves
Waves represent a central phenomenon in physics, possessing substantial importance across multiple fields of science and technology. Understanding waves is crucial not only for students and educators but also for researchers and professionals. Waves are involved in various everyday applications, from sound and light to radio signals and beyond. A solid grasp of wave mechanics can illuminate their influence on natural systems as well as engineered solutions.
In this section, we will explore the definition and historical background of waves, laying the foundation for an in-depth examination of their types and properties that follow. In essence, the study of waves spans across various domains, including acoustics, optics, and even quantum mechanics, making it a versatile topic.
Definition of Waves
A wave can be characterized as a disturbance or oscillation that travels through space and matter. It transfers energy from one location to another without a permanent displacement of the medium itself. This definition encompasses various forms of waves, such as mechanical, electromagnetic, and matter waves.
In more specific terms:
- Mechanical Waves require a medium, such as air or water, to propagate.
- Electromagnetic Waves do not need a medium and can travel through a vacuum, like light traveling from the Sun to Earth.
- Matter Waves, associated with quantum particles, exhibit wave-like behavior as described in quantum mechanics.
Thus, the definition of waves encapsulates a broad spectrum, underpinning many physical phenomena that shape our world.
Historical Background
The concept of waves has evolved significantly throughout history. Ancient civilizations observed various wave phenomena, though formal study began later. The study of waves can be traced back to the work of scientists like Pythagoras and Aristotle, who explored sound as a wave phenomenon.
In the 17th century, the understanding of waves advanced with the development of wave theory. Christiaan Huygens and other notable figures contributed greatly to wave mechanics.
"Waves are not just a mere curiosity; they are a fundamental aspect of nature itself."
Fast forward to the 19th century, James Clerk Maxwell contributed significantly by formulating the equations governing electromagnetic waves, establishing a connection between electricity and magnetism. This laid the groundwork for technologies we rely on today, such as radio and television.
In contemporary science, waves are pivotal in various technologies, ranging from medical imaging in healthcare to wireless communication. Thus, the historical context of wave study illuminates its significance in our shared scientific narrative.
Types of Waves
Waves can be classified into several categories, each with distinct characteristics and applications. Understanding the types of waves helps in grasping their behavior and effects across various domains. In this article, we will delve into two primary categories: mechanical waves and electromagnetic waves. Recognizing these types not only enhances our comprehension of wave mechanics but also highlights their significance in fields such as acoustics, telecommunications, and healthcare.
Mechanical Waves
Mechanical waves depend on a medium for transmission. They require particles in the medium to vibrate, thus transferring energy from one point to another. This transfer is crucial for understanding sound propagation and water wave behavior.
Transverse Waves
Transverse waves are distinguished by the deformation of the medium being perpendicular to the direction of energy transfer. A common example is a wave on a string. Each segment of the string moves up or down as the wave travels horizontally. The key characteristic of transverse waves is their crest and trough formation, which illustrates energy transfer visually.
Benefits of Transverse Waves:
Transverse waves contribute significantly to various fields. In seismology, they help scientists understand earthquake dynamics. Their ability to travel through solids but not fluids can aid in material testing and characterization.
Unique Feature:
A notable feature of transverse waves is their polarization ability. This allows certain applications in optics and communication, making them a popular subject in physics.
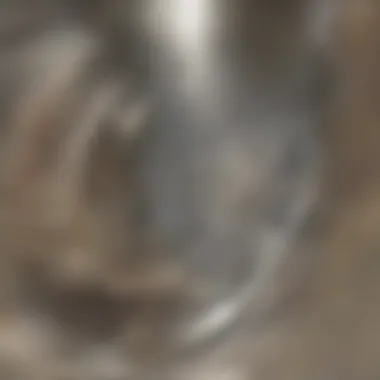
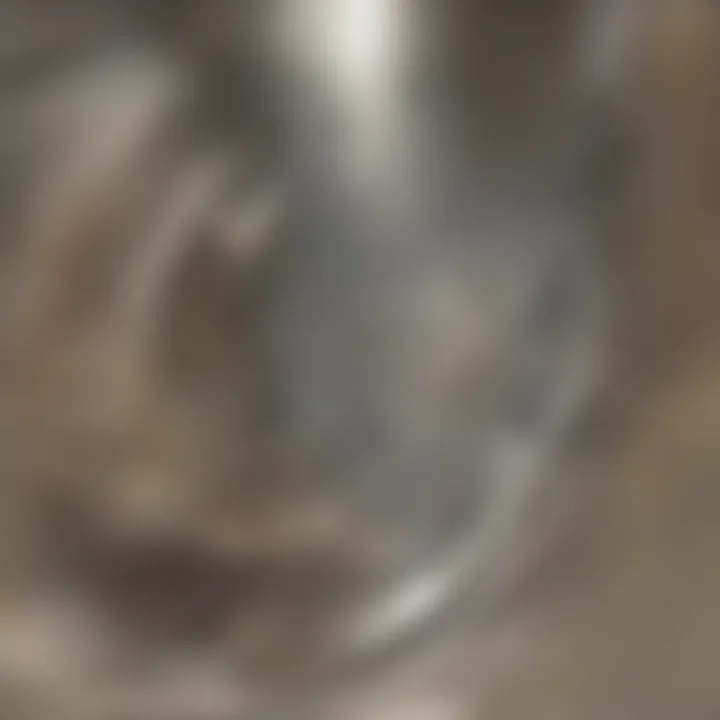
Disadvantages:
One disadvantage is that they cannot propagate through fluids, limiting their applications in those environments.
Longitudinal Waves
Longitudinal waves differ fundamentally in their structure. In these waves, the medium’s particles vibrate parallel to the direction of energy transfer, like in sound waves. It creates compressions and rarefactions in the medium, which effectively transmit sound.
Key Characteristic:
The main characteristic of longitudinal waves is their ability to travel through gases, liquids, and solids. This adaptability makes them essential for acoustics and other practical applications.
Benefits:
Longitudinal waves are critically important in many areas. They are the basis for sound transmission and also play an essential role in sonar and medical ultrasonography.
Unique Feature:
A unique feature of longitudinal waves is that they can travel faster in denser mediums, which is significant for various calculations in astrophysics and engineering.
Disadvantages:
However, longitudinal waves can be harder to visualize compared to transverse waves, which may complicate analysis in educational contexts.
Electromagnetic Waves
Electromagnetic waves do not require a medium and can propagate through a vacuum. They are essential to our understanding of light and various technologies that rely on these waves.
Radio Waves
Radio waves are part of the electromagnetic spectrum. They are widely used for communication purposes, including radio and television broadcasting. Their long wavelengths and ability to travel great distances without losing strength make them invaluable in telecommunication.
Key Characteristic:
The distinct feature of radio waves is their relatively low frequency, which allows them to carry information efficiently over vast distances.
Benefits:
The significant benefit is the capacity for communication without physical connections, which revolutionizes how information is transmitted globally.
Disadvantages:
However, radio waves can be susceptible to interference from other signals and obstacles, which affects signal clarity.
Visible Light
Visible light is the portion of the electromagnetic spectrum that can be detected by the human eye. This category encompasses a range of wavelengths, perceived as different colors. Understanding visible light is fundamental to optics and photography.
Key Characteristic:
Visible light’s main characteristic is its wavelength, which defines color perception.
Benefits:
It plays a critical role in vision, and it serves as the basis for many technologies, such as lasers and LEDs, widely used in everyday applications.
Disadvantages:
A limitation of visible light is that it is dependent on various factors like reflection and refraction, which can distort images and alter perception.
X-rays
X-rays possess sufficient energy to penetrate various materials and are primarily known for their medical applications in imaging. They provide important insights into the internal structures of objects, including human bodies.
Key Characteristic:
The unique characteristic of X-rays is their high energy, which is effective for visualizing bone structures and other dense materials.
Benefits:
X-rays have drastically improved medical diagnostics and treatment planning, making them a critical tool in healthcare.
Disadvantages:
On the downside, exposure to X-rays can pose health risks due to radiation, necessitating careful usage and safety protocols.
Matter Waves
Matter waves, rooted in quantum mechanics, describe the wave-like behavior of particles. This concept is fundamental in understanding phenomena like electron behavior in atoms and is crucial for fields such as quantum computing and nano-technology.
By categorizing waves into these types and understanding their properties, we gain insight into their relevance in daily life and advanced technological applications. Each wave type brings unique advantages and challenges, shaping our technological landscape and scientific exploration.
Wave Properties
Wave properties are essential for comprehending the behavior and characteristics of different types of waves. Understanding these properties helps in the analysis of wave phenomena in various applications. Wave properties allow us to describe waves quantitatively, differentiating between them based on their specific attributes. This section focuses on three key properties: wavelength, frequency, and amplitude.
Wavelength
Wavelength is the distance between two consecutive points that are in phase on a wave. These points can be peaks, troughs, or any other equivalent markers. Wavelength is typically denoted by the Greek letter lambda (λ). The importance of wavelength cannot be overstated; it plays a critical role in determining the wave's behavior and its interaction with the environment.
In the context of electromagnetic waves, for instance, different wavelengths correspond to different types of radiation.
- Radio waves have longer wavelengths, promoting effective long-distance communication.
- Conversely, ultraviolet rays have much shorter wavelengths that can lead to chemical changes.
Thus, the wavelength influences both the capabilities and limitations in fields such as optics and acoustics.
Frequency
Frequency refers to the number of wave cycles that pass a given point in one second. It is measured in hertz (Hz). High frequency indicates many cycles within a short time, while low frequency signifies fewer cycles. Frequency is inversely related to wavelength, meaning as one increases, the other decreases.
Frequency is crucial in various applications. For instance, in sound waves, frequency determines pitch. A high-frequency sound wave results in a high-pitched sound, while a low-frequency wave produces a lower pitch. In communications, radio frequency dictates the different channels we use, enabling varied signals to travel simultaneously without interference.
Amplitude
Amplitude represents the maximum displacement of points on a wave from its rest position. In simpler terms, it measures how "tall" or "strong" a wave is. This property largely determines the energy carried by the wave. A wave with a larger amplitude conveys more energy than one with a smaller amplitude.
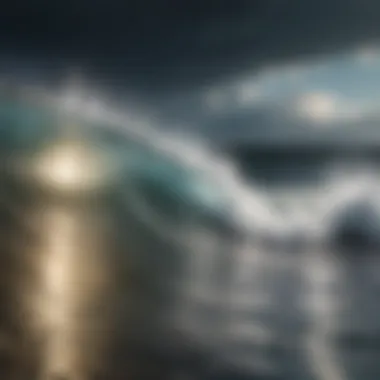
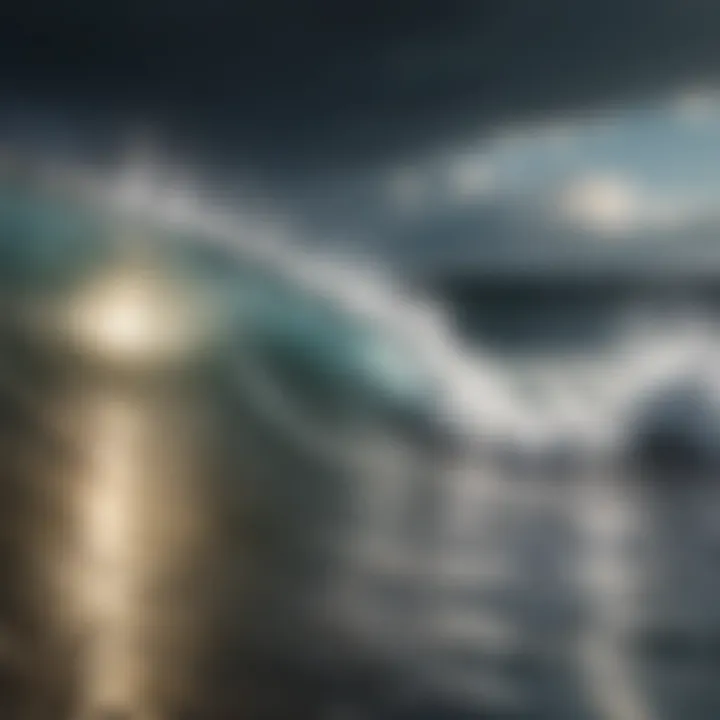
In sound waves, greater amplitude correlates with louder sounds. In contrast, smaller amplitude yields quieter sounds. For light waves, amplitude is associated with brightness. High amplitude means brighter light, whereas low amplitude results in dimmer light. Understanding amplitude is vital for engineers designing speakers, lasers, or even medical imaging devices.
"Wave properties connect fundamental physics concepts to real-world applications, shaping the technologies we use daily."
Exploring wave properties allows for deeper insights into wave behavior across various disciplines, from music to telecommunications. Each property has distinct significance and applications that influence our understanding of the wave phenomenon.
Wave Behavior
Understanding wave behavior is essential as it reveals how waves interact with various mediums and obstacles. The details of wave behavior shape our comprehension of numerous phenomena such as sound propagation, light bending, and signal transmission. It has profound implications in various fields ranging from acoustics to optics.
Reflection
Reflection occurs when a wave encounters a boundary or an obstacle and bounces back into the original medium. This phenomenon is crucial in understanding how waves behave in different environments. For instance, when a sound wave hits a wall, it reflects back, allowing humans to hear echoes. The law of reflection states that the angle of incidence is equal to the angle of reflection.
Key considerations regarding reflection include:
- The material properties of the surface, which influence how much energy is reflected.
- The angle at which the incoming wave strikes the surface.
- The smoothness or roughness of the surface, which can affect the clarity of the reflected wave.
Refraction
Refraction is the bending of waves as they pass from one medium to another. This bending occurs due to a change in the wave's speed. For example, light traveling from air into water travels slower, resulting in the change of direction. Refraction is responsible for various optical phenomena, such as the distortion of objects underwater.
Factors influencing refraction include:
- The wavelength of the wave.
- The physical properties of the mediums involved.
- The angle of incidence and the refractive index, which is a measure of how much a wave's speed changes in different media.
Diffraction
Diffraction refers to the spreading of waves as they encounter obstacles or openings. This phenomenon helps explain why sound can be heard around corners and how light can create patterns when passing through narrow slits. Diffraction is typically more noticeable with waves that have longer wavelengths, relative to the size of the obstacle or aperture.
Important aspects of diffraction:
- The amount of spreading depends on the wavelength and the size of the obstacle.
- Diffraction patterns can provide insights into the wave properties, such as wavelength and coherence.
Interference
Interference occurs when two or more waves overlap and combine. The result of this interaction can be constructive or destructive. Constructive interference happens when waves meet in phase, amplifying the overall amplitude, while destructive interference occurs when they meet out of phase, reducing the amplitude. This is observed in various contexts, such as thin film patterns and noise-canceling technology.
In interference, it's important to understand:
- The phase relationships between the waves involved.
- The principle of superposition, which dictates how overlapping waves affect amplitude.
- Applications in technology, such as in telecommunications and acoustics.
Understanding wave behavior is fundamental to many scientific and engineering applications, optimizing designs for better performance and efficiency.
Recognizing these key elements of wave behavior provides a foundational understanding applicable in many fields, from environmental science to communications technology.
The Wave Equation
The wave equation is a fundamental relation in physics that describes how waves propagate through different media. It serves as the cornerstone for understanding various physical phenomena related to waves, including sound, light, and other types of vibrations. By establishing a mathematical framework, the wave equation lays the groundwork for deeper explorations into the nature and behavior of waves.
Understanding the wave equation is crucial for both theoretical and practical applications. It provides insights into how waves interact with their environment, and predicts how changes in initial conditions can affect wave behavior. This makes it particularly relevant for researchers and professionals in fields such as acoustics, optics, and engineering. Moreover, mastering the wave equation helps in comprehending more complex systems in quantum mechanics and electromagnetism.
Deriving the Wave Equation
Deriving the wave equation typically begins with representing the displacement of a wave as a function of both position and time, denoted as ( y(x,t) ). The simplest wave equation is [ ] where ( v ) is the wave speed. This equation states that the second derivative of the displacement concerning time is proportional to the second derivative concerning space.
To derive this equation, consider that the wave properties remain consistent as they travel through space. We can begin with Newton's second law. For a small segment of the medium, applying this law results in equations that describe how the medium’s particles vibrate, leading to the conclusion that the acceleration of particles is proportional to the spatial gradient of the wave function. In essence, the wave's speed is dictated by the medium’s properties, such as tension in strings or the bulk modulus in fluids.
Once these equations are established, applying techniques from calculus, such as separation of variables, allows for the eventual conclusion that such systems adhere to the wave equation.
Applications of the Wave Equation
The wave equation has significant applications in various domains, reflecting its versatility and importance. Some of the major applications include:
- Acoustics: Understanding sound propagation enables advancements in audio engineering, architectural design, and noise control.
- Optics: Light behavior is analyzed through the wave equation, leading to innovations in optical devices such as lenses, microscopes, and lasers.
- Telecommunications: The principles derived from the wave equation are essential in developing technologies for efficient signal transmission in devices like radios and smartphones.
- Physics Research: The wave equation is integral in quantum mechanics, particularly when describing particle-wave duality and understanding phenomena like tunneling.
- Medical Imaging: Techniques like ultrasound rely on wave principles, informed by the wave equation, to visualize internal body structures.
Applications of Waves
Waves manifest in various forms and have far-reaching implications across numerous fields. Understanding the applications of waves facilitates deeper insights into their fundamental nature. In this section, we will explore the specific applications of waves in different domains, highlighting their importance and the benefits they provide.
Acoustics
Acoustics is the branch of physics that studies sound waves and their behavior in different mediums. The application of sound waves is pivotal in multiple areas, including music, architecture, medicine, and engineering.
- Music: Sound waves are essential in music production. Musicians manipulate sound waves to create instruments, voice, and various sound effects. In acoustics, the design of concert halls or theaters considers wave behavior to ensure optimal sound quality.
- Medicine: In healthcare, ultrasounds use high-frequency sound waves to image the inside of the body. This non-invasive technique has revolutionized prenatal care and diagnostics.
- Engineering: Acoustic wave sensors are used in engineering for structural health monitoring. They detect changes in materials by analyzing the propagation of sound waves.
Deepening our understanding of sound waves in acoustics not only enhances auditory experiences but also improves safety and human health through medical imaging.
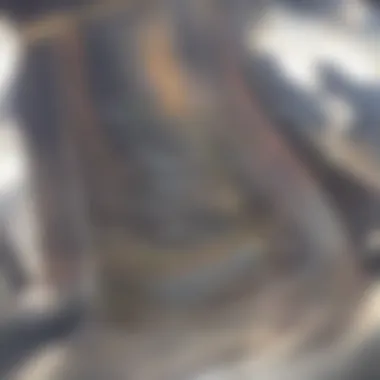
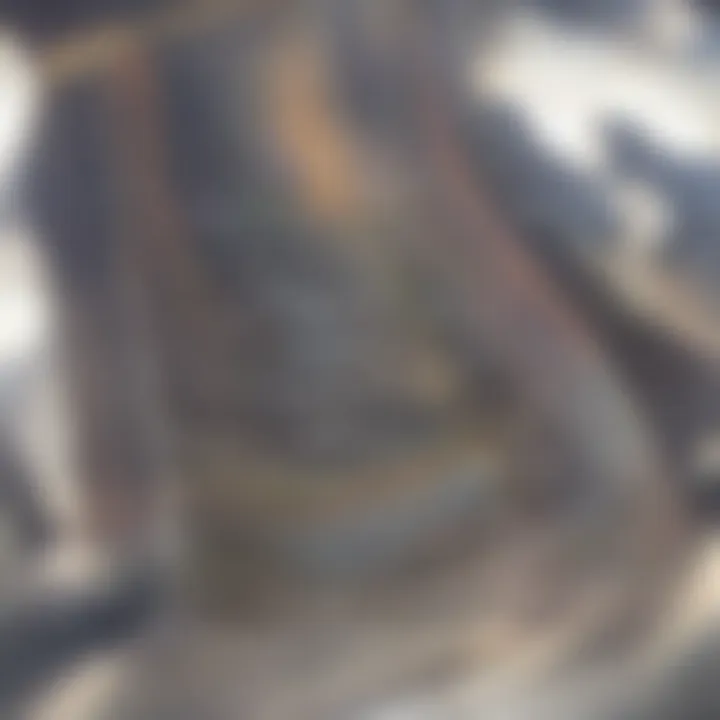
Optics
Optics involves the study of light waves and their interactions with different materials. The applications of optics are wide-ranging, affecting our daily lives profoundly.
- Lenses and Imaging: Optical lenses are foundational in developing cameras, glasses, and microscopes, enabling better vision and imaging capabilities.
- Fiber Optics: This technology utilizes light waves to transmit data at high speeds over long distances. It has transformed telecommunications, enabling faster internet and clearer communication.
- LASER Technology: The development of lasers relies on the principles of optics. Applications include surgery, manufacturing, and data storage.
Light waves are not just a means to see; they play a crucial role in communication, medicine, and technology, shaping modern society.
Communications Technology
The role of waves in communications technology cannot be underestimated. From radio to the internet, electromagnetic waves form the backbone of our global communication networks.
- Radio Waves: They facilitate wireless communication, allowing messages to be transmitted across large distances without the need for physical connections.
- Wi-Fi and Mobile Networks: These technologies use electromagnetic waves to provide internet access. Understanding wave behavior is essential for optimizing speed and signal strength.
- Satellite Communication: Satellites rely on wave transmission for broadcasting and connecting remote areas. They enable global positioning systems and weather forecasting services.
In the realm of communication, waves enable not only convenience but also connectivity of the modern world.
Waves, in all their forms, are fundamental to the fabric of our technology and society.
In summary, the applications of waves influence numerous sectors, enriching our understanding and enhancing our lives. Through their study, we gain insights into diverse fields, assisting in advancements that benefit both individuals and society at large.
Challenges in Wave Physics
The study of wave physics encounters numerous challenges that compel researchers and educators to rethink existing theories. This section outlines the significance of understanding these challenges. Wave phenomena are not merely abstract concepts; they underpin various technological advancements and scientific explorations. Such an understanding aids in designing better systems and enhancing communication technologies.
The interplay between theoretical mechanics and real-world applications sheds light on the necessity for continued research in wave physics. Considering elements like quantum mechanics and phenomena such as the Doppler Effect can lead to solutions for complex problems in diverse fields. This analysis not only engages students and researchers but also provides useful frameworks for professionals to address practical challenges.
Quantum Mechanics and Waves
Quantum mechanics introduces a set of challenges that reshape traditional views in wave physics. At its core, this theory indicates that waves exhibit particle-like properties, leading to a duality that defies classical intuition.
Key aspects include:
- Wave-Particle Duality: Objects behave as waves and particles, demanding a new way of understanding their behavior.
- Heisenberg's Uncertainty Principle: It states that certain pairs of properties, like position and momentum, cannot be precisely measured simultaneously. This provides a unique lens to analyze waves on a quantum level.
- Applications in Technology: Quantum mechanics leads to innovations in fields such as quantum computing and secure communications.
These challenges force scientists to reconsider how waves interact with matter at a fundamental level. Are they merely phenomena, or do they signify deeper properties of reality? Each advance in quantum theory contributes to this discourse, suggesting a connection between waves and the universe's fabric.
The Doppler Effect
The Doppler Effect provides another challenge in wave physics, affecting how waves are perceived and understood. It describes the change in frequency or wavelength of waves due to the relative movement of the source and observer. This effect becomes evident in various contexts:
- Sound Waves: The noticeable variation in pitch from a passing siren illustrates the classic Doppler Effect. When the source approaches, the frequency appears higher; as it moves away, the frequency drops.
- Light Waves: The redshift and blueshift in astronomy exemplify the Doppler Effect, offering insight into the movement of stars and galaxies. These shifts inform our understanding of cosmic expansion and the universe's evolution.
Understanding this effect holds practical implications for numerous applications such as radar and satellite technology. It is essential in analyzing astronomical data, enhancing communication systems, and more. Recognizing the Doppler Effect enhances our comprehension of wave dynamics and assists in solving real-world problems.
Ultimately, unraveling these challenges in wave physics not only enriches our theoretical knowledge but is vital for impactful technological advancements.
Future Directions in Wave Research
Understanding the future directions in wave research is essential due to its potential impacts on various scientific and technological domains. Waves are not just a fundamental concept in physics; they are integral to innovations that can redefine communications, medical imaging, and energy systems. Focusing on the advancements in wave research allows us to better appreciate how waves can be harnessed for practical applications.
Emerging Technologies
The advancement of technology continues to influence wave research significantly. One of the critical areas is quantum computing, which explores how wave phenomena can optimize computing processes. Technologies such as quantum cryptography rely on wave behaviors to achieve secure communication channels that are practically immune to interception.
Another emerging field is ultrafast optics. This area employs short laser pulses to capture the fastest phenomena in physical processes. By studying light waves at such short time scales, researchers can observe molecular interactions and understand fundamental chemical reactions in real time. This could lead to advancements in pharmaceutical development, where knowing a reaction's dynamics can help design better drugs.
In telecommunications, 5G technology is leveraging higher frequency waves to enhance connectivity. This requires extensive research into wave propagation and its impact on infrastructure. The role of waves in this technological leap is not just about speed, but also about exploring the potential of the electromagnetic spectrum.
Interdisciplinary Applications
Waves cross the boundaries of various disciplines, which is what makes them unique and valuable. The interplay between physics, biology, and engineering often leads to innovative applications. One critical area is in medical imaging; technologies like MRI rely on understanding wave behavior in magnetic fields. Researchers are constantly exploring ways to refine these imaging techniques by analyzing wave interactions at finer scales.
The use of waves extends into environmental science as well. Acoustic waves are employed in sonar technology to measure ocean depths and map underwater geological structures. This has a significant impact on understanding marine ecosystems and the effects of climate change on ocean environments.
Moreover, wave applications in artificial intelligence and data processing are emerging. Researchers are investigating how wave patterns can improve algorithms designed for analyzing large data sets. This interdisciplinary approach enhances efficiency in data processing and could lead to breakthroughs in various sectors.
"The study of waves is at the forefront of many technological advancements, shaping the future landscape of multiple disciplines."
Ending
In this concluding section, we encapsulate the essence of waves as discussed throughout the article. Waves represent a vital aspect of both natural and technological phenomena. They serve as a bridge connecting various scientific fields, providing insights that underpin both theoretical and practical applications. The relevance of waves extends far beyond traditional physics, influencing areas such as medicine, telecommunications, and even art.
One important element discussed is the versatility of wave types. Mechanical waves, electromagnetic waves, and matter waves each offer unique characteristics and applications. Understanding these variations helps in grasping how waves interact with environments, which is critical in fields like acoustics and optics.
Additionally, the properties of waves such as wavelength, frequency, and amplitude were analyzed. These properties are foundational in predicting wave behavior and are crucial in real-world scenarios, from audio engineering to the design of laser systems. Such knowledge equips students and professionals alike with essential tools for innovation.
Moreover, the behavior of waves—encompassing reflection, refraction, diffraction, and interference—provides a clear insight into their practical implications. This understanding is paramount when developing technologies that rely on wave interactions, such as communication devices and imaging techniques.
As we look to the future, emerging technologies and interdisciplinary applications of wave physics signify promising areas for further exploration. From advancements in quantum technologies to new materials in acoustics, the potential for discovery is vast.
Waves are not only essential for elevating our understanding of physics but also serve as critical components in the technological advancements shaping our world today.
In summary, this article serves not only as a resource for students and educators but also as a call to arms for researchers and professionals to appreciate the underpinnings of wave phenomena in their respective fields. The significance of waves cannot be overstated, as they are fundamental to our understanding of the universe and our daily lives.