Understanding Magnetic Computers: Future of Data Processing
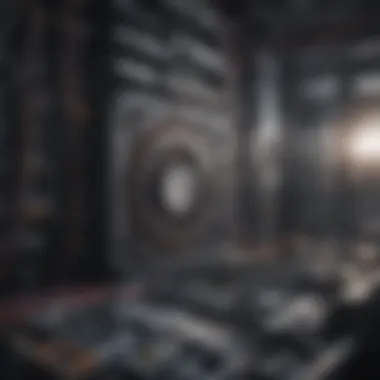
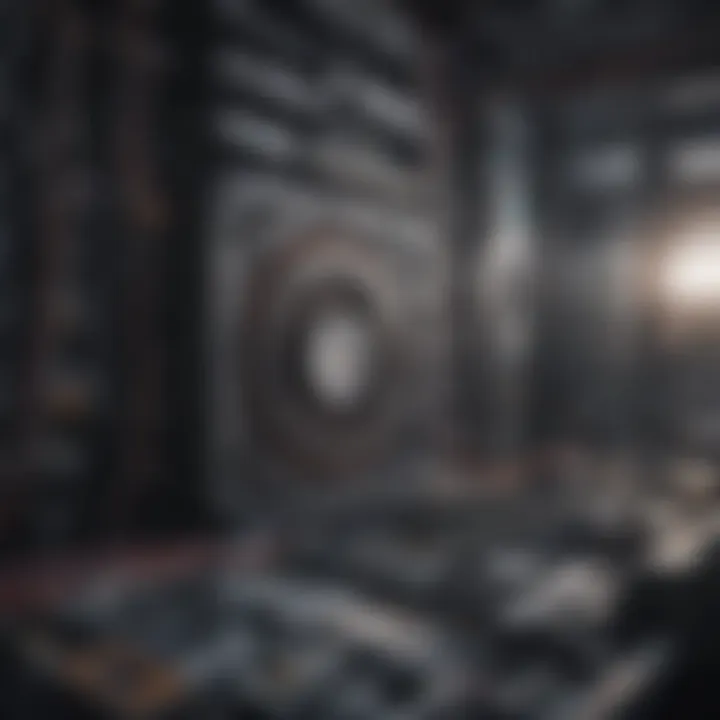
Intro
The realm of computing is constantly evolving, driven by the pursuit of higher efficiency and performance. In this context, magnetic computers emerge as a promising alternative to traditional silicon-based technologies. By harnessing the unique properties of magnetism, these computers are poised to reshape how data is processed and stored. This exploration begins with an examination of recent research findings and their significance in the field.
Key Research Findings
Overview of Recent Discoveries
Recent studies have unveiled compelling advancements in magnetic computing. Researchers have focused on developing magnetic components that can operate faster and consume less energy compared to conventional silicon counterparts. One notable development is the emergence of magnetic tunnel junctions (MTJs), which are essential for non-volatile memory applications. These structures enable the storage of information based on magnetic states, leading to faster read and write operations.
Another important discovery is the improvement in the scalability of magnetic computing systems. Researchers are exploring new materials and fabrication techniques that allow for miniaturization without compromising performance. This aspect is crucial as the industry shifts towards smaller, more efficient computational devices.
Significance of Findings in the Field
The implications of these findings are vast. First, magnetic computing presents a viable answer to the limitations of Mooreβs Law. As transistors reach their physical limits in miniaturization, exploring magnetic technologies could provide a pathway to sustained performance improvements. Furthermore, the energy efficiency of magnetic systems presents an opportunity to address growing concerns surrounding power consumption in computing.
The advancements in magnetic computing signify a potential paradigm shift in data processing technologies. High performance coupled with low energy usage lays a strong foundation for future innovations.
Breakdown of Complex Concepts
Simplification of Advanced Theories
Understanding magnetic computing requires familiarity with concepts like spintronics and quantum computing. Spintronics, short for spin transport electronics, leverages the intrinsic spin of electrons in addition to their charge. This dual utilization enables higher data processing speeds and storage capacity. Researchers are continually devising ways to utilize spintronics for practical applications.
Similarly, quantum computing is beginning to intersect with magnetic technologies. Quantum states, inherently tied to magnetic fields, offer the potential for exponentially faster computations. However, these theories are intricate and can be challenging to grasp without a solid foundation in quantum mechanics.
Visual Aids and Infographics
To aid in understanding these complex concepts, visual representation is instrumental. Diagrams showing the structure of magnetic tunnel junctions or flowcharts outlining the principles of spintronics can enhance comprehension. Utilizing such tools can demystify the various layers of magnetic computing technologies and their applications. Integrating these visuals in educational materials can greatly benefit students and professionals alike.
Preface to Magnetic Computers
The topic of magnetic computers is essential as we navigate the evolving landscape of data processing technologies. Unlike conventional computing architectures that primarily rely on semiconductors, magnetic computing presents an innovative approach to information storage and manipulation. This evolution in computing is significant because it may lead to faster, more energy-efficient systems with improved data integrity.
Understanding the basics of magnetic computers allows students, researchers, educators, and professionals to grasp the potential shifts in the computing paradigm. Key benefits include enhanced data retrieval speeds, a broader lifespan of storage media, and greater resilience to environmental factors. Moreover, magnetic computing holds the promise of integrating with emerging technologies, thus influencing ongoing research and development efforts within the industry.
Exploring the foundations of magnetic computers helps us to appreciate not only their advantages but also their historical journey, which informs current innovations and future prospects.
Definition and Overview
Magnetic computers utilize magnetic materials and phenomena to process information. The core principle relies on the manipulation of magnetic states, which can represent binary data as magnetized or demagnetized bits. This technology contrasts with traditional electronic circuits that employ electrical charge to represent data.
Magnetic materials, such as ferromagnets, exhibit special properties that enable data storage and retrieval through changes in magnetic orientation. Notably, magnetic random-access memory (MRAM) is one of the most progressive implementations. It offers several advantages over conventional memory types, such as static and dynamic random-access memory, by retaining information even when power is off.
The ability to store bits as magnetic states opens avenues for more compact systems with better performance. Data retention, speed, and energy efficiency are intrinsic advantages of magnetic computing, positioning it as a frontrunner in modern computational technology.
Historical Context
The development of magnetic computers can be traced back to the mid-20th century when the advent of magnetic storage devices began. Magnetic tapes and disks revolutionized the way data was stored and accessed, leading to widespread applications in businesses and research settings.
In the 1970s, magnetic core memory systems gained attention. They used tiny magnetic rings to hold individual bits of data, offering speed and reliability far surpassing preceding technologies. As semiconductors advanced, magnetic technologies faced stagnation; however, research has seen a resurgence in the 21st century. The interest has primarily shifted towards improving performance metrics of magnetic storage and exploring new architectures for computation.
Today, the landscape has changed significantly with the integration of magnetic computing technologies into emerging fields like quantum computing. Researchers are now exploring hybrid systems that enhance the capabilities of traditional computation, leading to what is considered the next phase in the evolution of data processing.
Understanding the intricate historical context of magnetic computing allows us to appreciate its role in shaping contemporary technology. As we move forward, analyzing its progression will provide valuable insights into the future trajectory of data processing innovations.
Fundamental Principles of Magnetic Computing
Magnetic computing represents a transformative shift in data processing paradigms. Understanding the fundamental principles of this technology is crucial. This understanding not only provides insights into how magnetic computers operate but also highlights the innovations that can emerge from this field. The core elements include the materials used and the encoding techniques that drive data storage and manipulation. Such knowledge forms the backbone of advancements and optimizations in magnetic computing, making it essential for anyone interested in the future of technology.
Magnetic Materials and Their Properties
Magnetic materials are at the heart of magnetic computing technology. These materials have unique properties that allow for effective data storage and retrieval. Common magnetic materials include iron, cobalt, and nickel, among others. These materials exhibit ferromagnetism, which is a property that presents potential for high-density data storage.
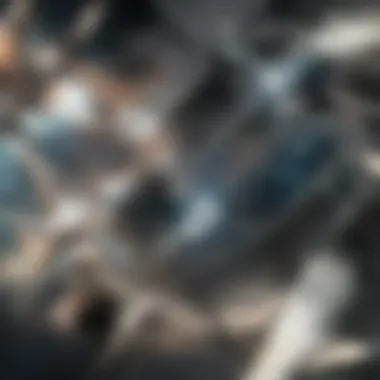
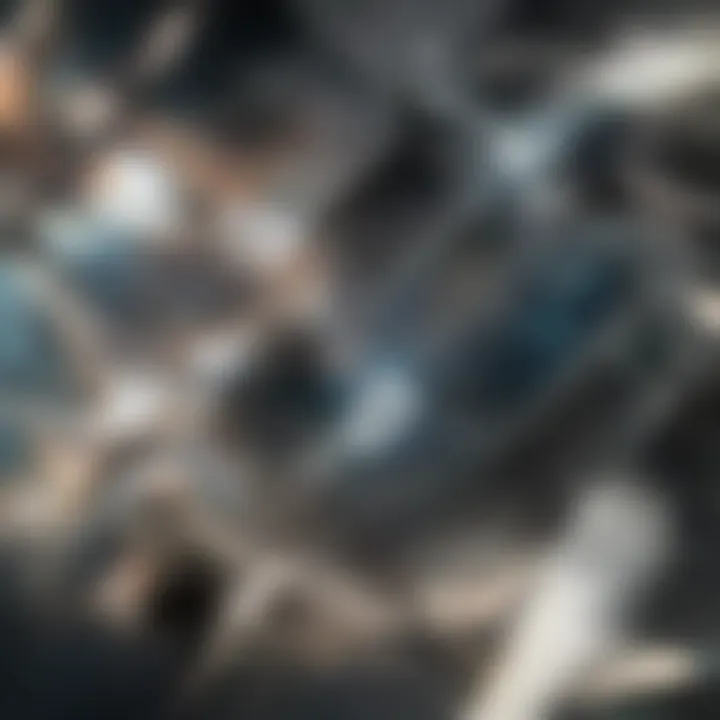
The choice of magnetic material impacts performance. For example, materials with higher permeability can enhance signal processing efficiency by lowering noise levels. Additionally, magnetic materials have temperature sensitivity that can affect their operational reliability. This interplay of properties presents both opportunities and challenges, requiring continuous research for improved performance.
Data Encoding Techniques
Data encoding is another critical aspect of magnetic computing. It dictates how information is represented, processed, and retrieved. Two prominent techniques are Binary Encoding and Quantum Bits.
Binary Encoding
Binary encoding is the foundation of digital data representation. In this system, all data is converted into a series of ones and zeros. The simplicity of binary encoding makes it a compelling choice for magnetic computers.
A key characteristic of binary encoding is its robustness. It provides clear distinctions between the two states of data. This binary nature reduces the chance of errors during data processing.
The unique feature of binary encoding lies in its universality. It is widely supported across different computing systems and languages. However, it does have limitations. The sheer scale of data might lead to excessive power consumption and slower processing speeds. Overall, binary encoding is essential for efficient data manipulation in magnetic computing.
Quantum Bits
On the cutting edge of data representation are quantum bits, or qubits. Unlike binary encoding, which uses two discrete states, qubits can exist in multiple states simultaneously. This property is called superposition, and it allows for more complex calculations.
A distinguishing feature of qubits is their potential for parallel processing. This can significantly speed up algorithms, offering advantages in fields like cryptography and data analysis. However, the technology is in its nascent stages, posing challenges in terms of stability and error correction.
Architecture of Magnetic Computers
The architecture of magnetic computers is crucial in understanding their unique role in the evolution of data processing. It involves the arrangement and interaction of various hardware components specifically designed to optimize magnetic storage and retrieval systems. This architecture not only supports the efficient operation of magnetic technologies but also paves the way for innovative advancements and applications in computing.
Basic Components
Magnetic Tapes
Magnetic tapes have been a foundational element of data storage for decades. They utilize a long, thin strip of plastic film coated with a magnetic substance to record data. One significant aspect of magnetic tapes is their ability to store large quantities of data at a relatively low cost. This cost-effectiveness makes them a popular choice for archival storage in many industries.
A key characteristic of magnetic tapes is their sequential access nature. Unlike hard disk drives, where data can be accessed randomly, magnetic tapes require a linear movement to reach desired data segments. This unique feature presents both advantages and disadvantages. On one hand, the sequential nature allows for high storage densities and lower manufacturing costs. On the other hand, accessing specific data points can be slower due to the need for tape movement.
Disk Drives
Disk drives, in contrast to magnetic tapes, offer random access capabilities, which significantly enhances data retrieval speeds. A fundamental aspect of disk drives is their use of rotating disks coated with magnetic material, commonly known as platters. This design enables quick access to data, making disk drives suitable for applications demanding rapid data retrieval and manipulation.
The key characteristic of a disk drive is its speed and efficiency. These drives can handle complex data operations with ease, making them a preferred choice for computing environments where performance is critical. However, while disk drives can be faster, they typically come at a higher cost per gigabyte compared to magnetic tapes. Additionally, their mechanical components make them more susceptible to physical damage compared to tape systems.
Circuit Design
The circuit design of magnetic computers plays a pivotal role in ensuring the seamless integration of all components. It involves creating pathways for electrical signals that control data flow and processing tasks effectively. A well-structured circuit design enhances the overall performance of magnetic computing systems, balancing speed, reliability, and energy efficiency. Careful consideration is given to the arrangement of circuits to minimize interference and optimize data throughput.
Thus, the architecture of magnetic computers, encompassing both basic components and circuit design, is integral to understanding their function in the broader context of data processing evolution. As technology advances, ongoing innovations in architecture will continue to reshape the capabilities and applications of magnetic computing systems.
Operational Mechanics
The operational mechanics of magnetic computers are crucial for understanding their functionality and efficiency in data processing. This section delves into the critical processes of data retrieval and writing mechanisms, which directly impact performance and reliability.
Data Retrieval Process
Data retrieval in magnetic computers involves accessing stored information using magnetic materials. The primary method for achieving this is through the use of read/write heads that interact with the magnetic medium. These heads analyze the magnetic fluctuations to decode data. This process is essential for ensuring that the system can access the needed information quickly and accurately.
This efficiency is particularly beneficial in environments that require high-speed data processing, such as data centers or supercomputing facilities. The retrieval operation is generally categorized into two main techniques:
- Sequential Access: The head must move to a specific location on the tape or disk. This method is slower as it requires time to navigate the medium.
- Random Access: More advanced systems allow immediate access to any particular data segment. This greatly enhances operational speed and reduces latency.
As magnetic computer technologies advance, the focus on improving data retrieval processes continues. Enhancements in magnetic materials and the precision of read/write mechanisms can lead to faster access times, thereby improving overall system performance.
Data Writing Mechanisms
The data writing mechanisms in magnetic computers are equally significant. This involves encoding data onto the magnetic medium through alterations in the magnetic field. Data is represented in binary form, which needs to be converted into magnetic signals that can be stored.
The primary techniques for data writing are:
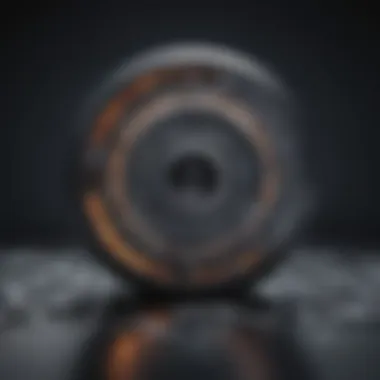
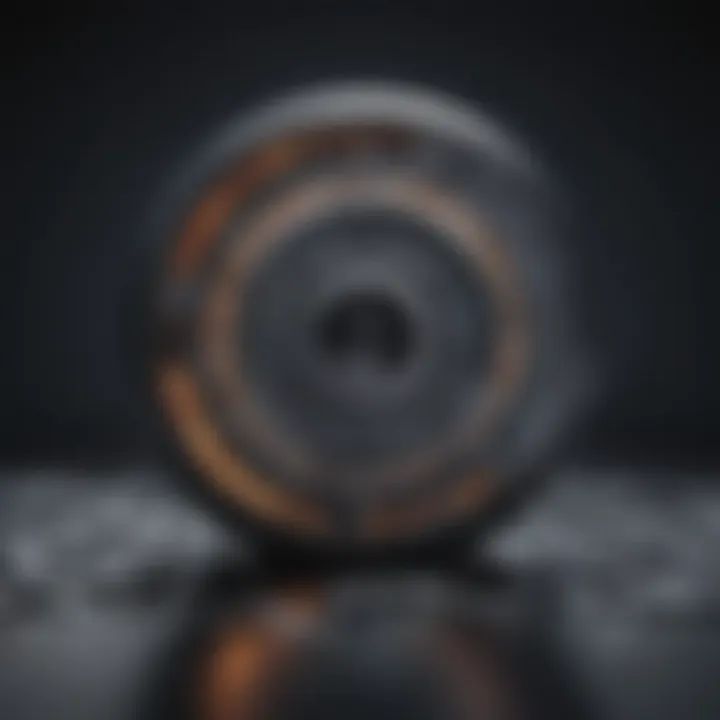
- Magnetization Patterns: The write head generates patterns that represent digital data by changing the magnetic orientation on the storage medium.
- Pulse Writing: In this method, the write head sends short pulses at specific intervals to create distinct magnetic states. This method enhances data density and efficiency.
Efficient writing mechanisms are vital. They determine how quickly data can be written and how effectively it can be stored for future retrieval.
"Speed and accuracy in data writing are paramount for the competitiveness of magnetic computing systems."
Understanding these mechanisms helps in grasping how magnetic computers can offer improved performance over traditional technologies. As we look toward the future, advancements in these areas could propel magnetic computing into more mainstream applications, addressing many of the limitations faced today.
Advantages of Magnetic Computers
The consideration of the advantages of magnetic computers is crucial in understanding their potential to revolutionize the landscape of data processing. As technology evolves, the need for efficient and cost-effective solutions becomes increasingly significant. Magnetic computers stand out for several reasons. Their inherent properties offer both economic and practical benefits that can appeal to various sectors.
Cost-Effectiveness
Magnetic computers present a compelling case for cost-effectiveness, particularly when compared to traditional semiconductor-based systems. Organizations are often under pressure to manage expenses while still maintaining high performance. Magnetic storage systems, especially those utilizing magnetic tapes, can be much less expensive in terms of data storage per gigabyte. This makes them an enticing choice for enterprises managing large amounts of data.
Considerations about energy consumption also play a role. Magnetic storage requires less energy to maintain data over time than some flash-based systems. This results in lower operational costs which can be beneficial for institutions that rely on extensive data archiving. Furthermore, the market availability of magnetic storage solutions allows for competitive pricing, making them accessible to a broad range of users.
- Cost advantages of magnetic computers include:
- Lower initial investment for data storage infrastructure.
- Reduced operational costs due to lower energy requirements.
- Competitive pricing in the market, allowing for flexible budgeting strategies.
Longevity and Durability
Longevity is another pivotal advantage of magnetic computers. These systems are designed to withstand various environmental pressures that might degrade other forms of data storage, such as solid-state drives. The physical nature of magnetic storage means it is less susceptible to wear and tear, lending to its durability.
Studies indicate that magnetic tapes can last for decades without significant data loss, provided that they are stored properly. This stands in contrast to the shorter lifespan of many solid-state disks, which can fail unpredictably.
Reasons for the durability of magnetic computers include:
- Resistance to physical shocks and magnetic fields.
- Lesser degradation over time when maintained correctly.
- Established history of archival storage capabilities in enterprise environments.
"Magnetic storage's remarkably long lifespan enhances its role in data archiving and backup processes."
Challenges in Magnetic Computing
Magnetic computing promises many advancements, but it does not come without hurdles. These challenges must be addressed for magnetic computers to fully integrate into mainstream technology. Understanding these issues helps in appreciating the obstacles faced in the evolution of magnetic computing.
Technological Limitations
The field of magnetic computing is still in its infancy, leading to a myriad of technological limitations. One immediate concern is the data transfer speed. While traditional magnetic storage solutions, like hard disk drives, have made significant progress, their read/write speeds are still slower compared to solid-state drives. This limitation can hinder the overall performance of magnetic computing systems.
In addition to speed concerns, energy efficiency is another critical challenge. Magnetic materials often require substantial power to maintain their states, especially when transitioning between data states. The drive for innovation necessitates the development of materials that can overcome these energy hurdles while enhancing speed performance.
Lastly, the reliability of magnetic systems is often questioned. As magnetic materials can degrade over time, the potential for data loss is higher. This leads to a reluctance in adopting such systems for critical data-heavy applications. The reliability issue is heightened in high-density storage where the spatial arrangement of magnetic bits influences the potential for error.
Market Acceptance
Another significant challenge is market acceptance. The technology landscape is extremely competitive, which means new entrants must demonstrate a clear value proposition to gain traction. Despite the advantages of magnetic computing, businesses often hesitate due to the maturity of existing technologies, like SSDs and cloud computing solutions.
Part of market hesitation stems from perceived risks. Organizations are typically cautious when it comes to adopting new technology that may require substantial investment in both time and resources. The potential for high costs associated with transitioning away from established technologies can lead to resistance.
Moreover, widespread adoption also depends on educating stakeholders about the benefits of magnetic computers. Many decision-makers may not yet grasp the advantages of transitioning to magnetic systems. Without an in-depth understanding, convincing industries to commit to a new technology becomes a daunting task.
"The challenge is not merely technological; it's fundamentally about building trust and demonstrating the tangible benefits of magnetic computing to potential users."
Applications of Magnetic Computers
The applications of magnetic computers extend beyond simple data storage. They represent a significant advancement in data processing. These systems are vital in fields where large amounts of data must be efficiently processed and stored. Their ability to combine speed, capacity, and reliability makes them suitable for various industries.
Data Storage Solutions
Magnetic computers excel in data storage solutions due to several factors. They offer large storage capacity and quick access speeds. This makes them suitable for both personal and enterprise-level applications. Magnetic tape and hard disk drives continue to be prominent technologies in this area. Companies utilize tape drives for offsite backups and long-term archiving. These traditional methods are still relevant because they can store vast amounts of data with relatively low cost.
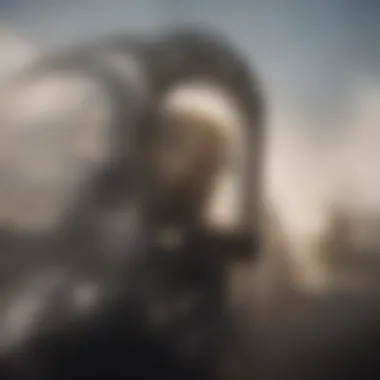
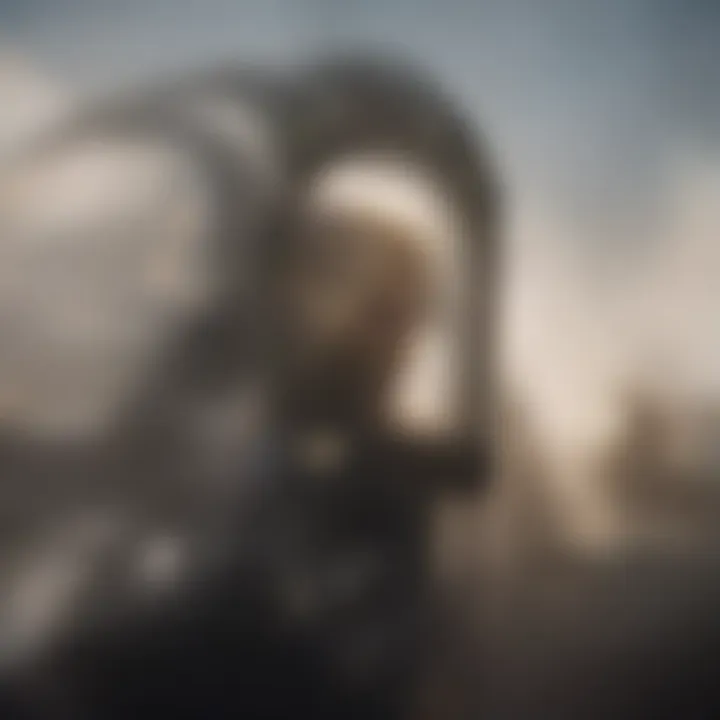
Additionally, using magnetic disks allows for efficient random access of data, critical in real-time applications. The durability of magnetic storage media also contributes to its long-standing presence in data storage. It can withstand various environmental conditions, providing peace of mind for users who handle sensitive information.
Supercomputing Facilities
In supercomputing facilities, magnetic computers play a pivotal role. They are essential for complex computations and simulations. The combination of magnetic materials with advanced processing capabilities enables higher computation speeds and process efficiencies. Supercomputers often require massive data handling capacities. Magnetic computers can meet these demands by efficiently managing large datasets.
Furthermore, the scalability of magnetic computing systems helps supercomputers adapt to growing computational needs. This adaptability is crucial in a landscape where data continuously increases. Magnetic computers contribute to high-performance computing by allowing rapid data sharing across systems within the facility.
"The future of supercomputing relies heavily on the integration of magnetic computing technologies to achieve unprecedented performance levels."
The Future of Magnetic Computing
The future of magnetic computing holds significant potential for revolutionizing data processing and storage. As traditional silicon-based computers face limitations in performance and efficiency, magnetic technologies are emerging as a feasible alternative. The unique properties of magnetic materials, such as speed and energy efficiency, make them well-suited for next-generation computing tasks. These advancements are not merely theoretical; they are increasingly being applied in real-world scenarios.
Predicted Trends
Several trends are anticipated to shape the future of magnetic computing:
- Hybrid Systems: As the demand for faster data processing grows, hybrid systems that integrate magnetic components with conventional silicon architectures are likely to become common. This approach combines the benefits of both technologies, achieving enhanced performance and efficiency.
- Increased Adoption of Quantum Technologies: Magnetic computing is predicted to play a crucial role in quantum computing. There will be intersections where magnetic materials facilitate the development of quantum bits, improving data reliability and accelerating processing speeds.
- Emergence of Advanced Storage Solutions: New storage systems that leverage magnetic technology, such as heat-assisted magnetic recording (HAMR), are set to redefine data storage capabilities. This technology promises to significantly increase storage densities, allowing more data to be kept in smaller physical spaces.
- Rise in AI Applications: The growing intersection of artificial intelligence and magnetic computing is expected to yield optimal results for machine learning algorithms, providing faster processing speeds and reduced energy consumption.
Research and Development Focus
The R&D in magnetic computing is concentrated on several key areas:
- Understanding Material Properties: Ongoing research seeks to deepen the understanding of the magnetic properties of materials. This knowledge will pave the way for the development of new materials that enhance performance and efficiency.
- Enhancing Data Encoding Techniques: Innovations in data encoding protocols, including advanced error correction techniques, are crucial for the robustness of magnetic computing. Research will focus on developing methods that improve the reliability of data storage and retrieval.
- Scalability of Technology: Another vital focus area is scalability. As market demands grow, the ability to scale magnetic computing solutions without a proportional increase in cost is critical. R&D efforts aim to create cost-effective production processes and materials.
- Sustainability Considerations: With growing concerns over environmental impact, researchers are prioritizing sustainable practices in the development of magnetic computing technologies. Investigating renewable materials and energy-efficient production methods will shape the industry.
In summary, the future of magnetic computing appears bright, supported by focused research and anticipated trends that emphasize performance, efficiency, and sustainability. The potential applications are vast, indicating that magnetic computing might play a pivotal role in shaping the computing landscape of tomorrow.
Case Studies in Magnetic Computing
Case studies in magnetic computing serve as practical illustrations of the theoretical concepts discussed throughout this article. They offer valuable insights into how magnetic computing technologies are being implemented in real-world scenarios. This section outlines the benefits and considerations of these case studies, emphasizing their significance within the broader context of magnetic computing.
By examining successful implementations, one can identify effective strategies and methodologies that can be replicated. Moreover, case studies highlight how magnetic computing solutions address specific needs in various sectors, ultimately influencing future developments in the field.
Successful Implementations
Successful implementations of magnetic computing systems illuminate the capability and versatility of the technology. Examples in various sectors include:
- Healthcare Data Management: Hospitals have successfully integrated magnetic data storage systems to manage vast amounts of patient records. These systems ensure data integrity and quick access, crucial in emergency situations.
- Scientific Research: Institutions like CERN utilize magnetic storage solutions for experiments. The need for reliable data handling and retrieval is met through advanced magnetic systems, which allow researchers to focus on their analysis rather than data limitations.
- Financial Services: Many financial institutions are adopting magnetic computing to handle large transactions. This ensures that data is consistently archived and easily retrievable, which is vital for audits and regulatory compliance.
These implementations show the adaptability of magnetic technologies across industries that demand reliability and efficiency.
Insights from Industry Leaders
Listening to insights from industry leaders in magnetic computing provides clarity on future trends and challenges. Experts often share their experiences, highlighting crucial takeaways:
- Emphasis on Research: Many leaders stress the importance of ongoing research and development. For example, advancements in quantum bits could pave the way for more sophisticated magnetic computing applications.
- Market Dynamics: Industry experts discuss how competition shapes the adoption of magnetic systems. As new technologies emerge, the need to stay ahead drives continuous improvement.
- Educational Initiatives: Leaders advocate for more educational programs focused on magnetic computing technology. Building a knowledgeable workforce is vital for driving innovation in this sphere.
"Understanding real applications and experiences in the field of magnetic computing will guide future innovators to refine their approaches and contribute to this vital technology."
This rich narrative encapsulates the potential and challenges of magnetic computing, providing essential knowledge for researchers and professionals.
The End
The significance of the conclusion in an article about magnetic computers cannot be overstated. This final section serves as a synthesis of the insights discussed throughout the piece, reinforcing the essential aspects of magnetic computing. Understanding this topic helps in appreciating not only the technical details but also the broader implications for the future of data processing. It encourages readers to consider the changing landscape of computing technology and the role magnetic computers may play moving forward.
Summary of Key Insights
In summary, this article highlights several key insights regarding magnetic computers:
- Innovative Architecture: Magnetic computers employ unique structures and components that set them apart from traditional silicon-based systems.
- Operational Efficiency: These machines promise better data retrieval and writing mechanisms, potentially leading to higher performance in various applications.
- Cost and Longevity: The cost-effective nature of magnetic technology combined with its durability positions it as a favored option for long-term data storage solutions.
- Challenges and Opportunities: While there are challenges in terms of market acceptance and technological limitations, ongoing research and development may mitigate these issues while enhancing capabilities.
By compiling these insights, a robust understanding of magnetic computers can be developed. Their future trajectory can influence various sectors, from personal computing to complex data centers.
Final Thoughts on the Impact of Magnetic Computing
In the grand schema of technological advancement, magnetic computing stands poised to redefine how data is processed and stored. As we observe a continual increase in data generation across diverse fields, the demand for efficient, scalable, and reliable data solutions becomes more critical.
As emphasized in the article, the potential impact of magnetic computers extends beyond mere performance metrics. Their success hinges not only on technical specifications but also on how well these systems can integrate into existing frameworks and systems.
"The future of magnetic computing lies in its ability to adapt and innovate amidst rapidly changing technological landscapes."