Exploring the Concept of Emergence in Science
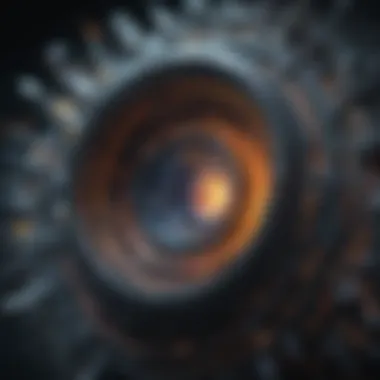
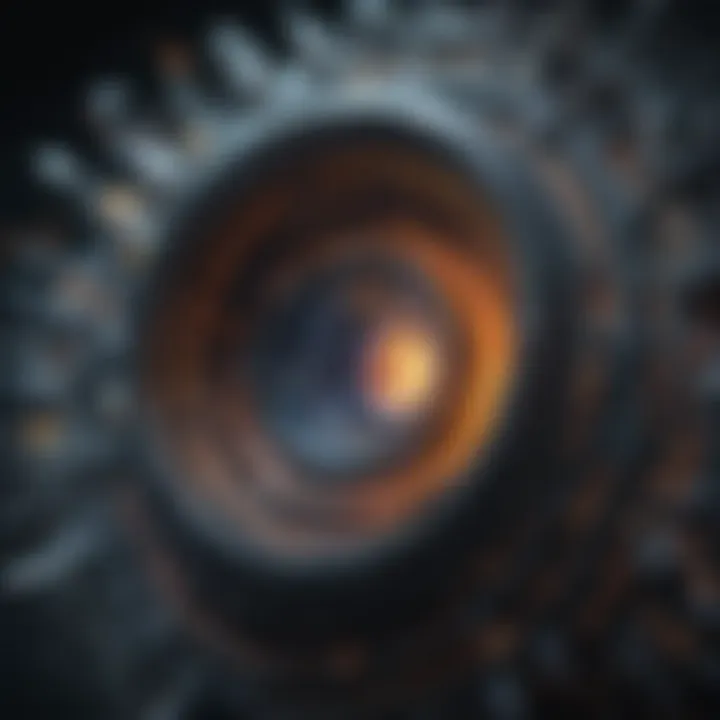
Intro
Emergence is a term that has nestled itself into various scientific conversations, gaining traction in fields such as physics, mathematics, and biology. But what exactly does it mean? Emergence can be thought of as the phenomenon where larger entities exhibit properties and behaviors not readily apparent from the individual parts that constitute them. This isn't just philosophical musing; it has real-world applications and implications in understanding complex systems.
In this discussion, we will navigate through key research findings, dissect complex ideas, and draw connections that enhance our understanding of emergence. By the end, readers from various scientific backgrounds will be better equipped to grasp the concept's significance and its influence on theories and practical applications.
Key Research Findings
Overview of Recent Discoveries
In recent years, there has been a wealth of research aimed at unpacking the intricacies of emergence. For example, in physics, studies on phase transitions—like that observed in water turning to steam—serve as a classic illustration of how emergent phenomena manifests. This is not about individual molecules but how they interact collectively under certain conditions.
In the realm of biology, research has been illuminating the concept of emergent behavior in ecosystems. A study conducted by scientists exploring ant colonies demonstrated how relatively simple rules governing the actions of individual ants lead to sophisticated foraging patterns that benefit the entire colony.
Significance of Findings in the Field
These findings are more than just interesting anecdotes. They help reshape our understanding of complex systems. For scholars and practitioners, recognizing that the whole can be greater, or different, than the sum of its parts opens up fresh avenues for inquiry and innovation. The implication is that in systems where emergence occurs—be it an ecosystem or a network of neurons—basic predictions based solely on component behaviors may lead to flawed conclusions.
"To understand emergence is to grasp that not every puzzle can be solved by merely understanding the individual pieces."
Breakdown of Complex Concepts
Simplification of Advanced Theories
The idea of emergence isn’t confined to a narrow slice of academia; it's a thread woven through many disciplines. That said, addressing its complexities can be daunting.
One effective way to break it down is to relate it to something familiar. Think of a sports team. Each player has their role, yet when they come together, they can create a synergy that leads to unexpected outcomes, such as winning a championship.
Visual Aids and Infographics
Visual representations can help make the concept of emergence clearer. Here are some ideas:
- Diagrams showing how simple rules lead to complex patterns, like flocking behavior in birds.
- Infographics that illustrate emergent properties across different fields.
- Charts depicting how individual behaviors cumulate into group phenomena, emphasizing the visual impact of these interactions.
These tools can serve as great aids, particularly for those who might struggle with purely analytical descriptions, providing a lens through which to see the broader picture of emergence.
Defining Emergence
To grapple with the concept of emergence is to tread through a necessary yet intricate dialogue about how complex systems operate in ways that often diverge from our direct understanding of their parts. In our ever-evolving scientific landscape, defining emergence becomes a pivotal task, facilitating a framework in which diverse branches of knowledge interconnect. This section lays the groundwork, shedding light on the fundamental aspects that underpin the notion of emergence while highlighting its significance in the realm of scientific inquiry.
Emergence is not merely a scientific terminology; it evokes questions about how interactions, relationships, and behaviors manifest at different scales. Various disciplines—be it physics, biology, or mathematics—grasp at this elusive concept, where the whole stands in stark contrast to the sum of its pieces. For students, educators, researchers, and professionals alike, a clear understanding of what constitutes emergence shapes not only theoretical discourse but also practical applications in technology and science.
Philosophical Foundations
The roots of emergence can be traced back to philosophical explorations. Philosophers have long wrestled with the implications of emergence. The inquiry begins with the mind-body problem, which considers whether the mind can be entirely explained through physical processes in the brain. Here, we see a practical example of the philosophical discourse informing scientific exploration. The question of emergence pushes us to ask: when does a collection of neurons transform into consciousness?
The discussion extends into considerations of reductionism and holism. Reductionism seeks to break down systems into their smallest components to understand them better. Conversely, holism posits that systems can exhibit properties that cannot be derived simply from their parts. This tension between the two philosophies forms the backdrop of debates in various scientific fields. How might emergence challenge our understanding of reality? Such questions invite philosophical rigor and depth into scientific inquiry, pushing boundaries of our existing knowledge.
"Emergence helps bridge the gap between the simple and the complex, forming a rich tapestry of explanations in both the sciences and philosophy."
Scientific Definitions
In a scientific context, defining emergence requires precision. The term captures complex phenomena through several lenses, each revealing different facets of the same coin. Weak emergence refers to situations where a phenomenon can be computed or predicted from its components, albeit this process can be computationally intensive. An example might involve fluid dynamics, where individual molecules' interactions lead to emergent properties like turbulence, which are challenging to predict without sophisticated modeling.
In contrast, strong emergence denotes systems in which new properties arise that cannot be explained by their components, regardless of the analytical depth. Consciousness, often cited in cognitive sciences, is frequently considered a case of strong emergence. Here, self-organizing behavior and unpredictable dynamics present profound implications for how we study life and intelligence.
Additionally, there is distinction between global and local emergence. Global emergence deals with properties observable at a macro level—think weather patterns arising from innumerable micro-interactions of particles. Local emergence, on the other hand, refers to properties that can emerge within a subset of the whole, often overlooked because they may occur in a confined context yet provide crucial insights into underlying mechanisms.
As we venture into other sections of this article, understanding these basic definitions sets the stage for richer, interdisciplinary discourse. Emergence is more than a term; it's an invitation to think critically about how complexity shapes the world and what that means for our continued exploration in both science and philosophy.
Historical Context
Understanding emergence necessitates a deep dive into its historical context. This exploration sheds light on how our comprehension of complex systems has evolved. It's not just about recognizing patterns but understanding the origins of those patterns in scientific thought, philosophy, and the collective inquiry that has shaped our modern conception of emergence. Historical context provides the necessary lens to view the progression of theories and ideas, allowing us to appreciate how early inquiries paved the way for today's advanced scientific explorations.
Early Theories of Emergence
The roots of emergence can be traced back to early philosophical discussions. Thinkers like Aristotle stressed the importance of the whole being greater than the sum of its parts. He used the example of a horse – merely observing a horse doesn't encompass all that is required to understand what it means to be a horse. This notion of wholeness began to take shape as various disciplines took their turn at interpreting it.
In the 19th century, the nascent field of biology began to embrace the concept of emergence through the lens of vitalism. Here, practitioners believed that life possessed intrinsic features that could not be fully explained by chemistry alone. This idea eventually lost traction with the rise of molecular biology; however, the debate about whether phenomena could emerge from complex interactions remained.
"Emergent properties in scientific contexts remind us that simplicity often lies beneath the surface, waiting to be uncovered."
20th Century Advances
As the 20th century rolled around, the scientific community began to rigorously challenge and refine theories of emergence. The advent of systems theory introduced a framework that emphasized relationships and patterns over mere components. Notably, von Bertalanffy’s General Systems Theory pushed the idea that systems, regardless of their nature—be it ecological, biological, or social—exhibited emergent properties that couldn’t be deciphered solely through component analysis.
Later, with the rise of quantum mechanics, scientists like Bohr and Heisenberg suggested that emerging properties at the subatomic level differed starkly from classical interpretations of matter. Their work compelled researchers to rethink not just how they approached matter but how holistic perspectives could yield insights into complex systems.
In addition, the evolution of computer simulations and complexity science propelled these discussions forward in the last few decades, allowing for concrete examples of emergent behavior in artificial environments. Concepts like self-organization and chaotic dynamics became central, further solidifying the importance of observing how individual elements can lead to unexpected collective behaviors.
This historical arc illustrates how notions of emergence evolved. From philosophical musings to scientific rigor, the path has been marked not merely by a change in understanding but by radical shifts in what it means to study complex systems. With this historical perspective, we gain a richer appreciation of emergence in contemporary contexts.
Types of Emergence
Understanding the different types of emergence is crucial for grasping the full scope of how complex systems function in both natural and artificial environments. Emergence can manifest in various forms, with each type playing a significant role in how we interpret phenomena in fields such as physics, mathematics, and life sciences. By diving into these categories, we can better appreciate the processes that give rise to new properties and behaviors that are not readily apparent from the individual parts.
Weak Emergence
Weak emergence generally refers to phenomena that can, in theory, be explained by the properties and interactions of their constituent parts, albeit with considerable complexity. The best way to understand this type of emergence is through examples in computer science, particularly in simulations and model development. For instance, in cellular automata, simple rules can lead to intricate patterns and behaviors. Conway's Game of Life beautifully illustrates weak emergence where individual cells follow basic rules to create surprising patterns that resemble life-like structures.
Key elements of weak emergence include:
- Simplicity in rules: The emergent behaviors stem from basic interactions under defined rules.
- Predictability: Although complex, the outcomes can be predicted if one knows the initial conditions and rules.
- Computational frame: Weak emergence often relies on computational models to demonstrate how complex behavior arises from simple rules.
"The patterns observed in weak emergence often serve as a metaphor for understanding complexity in other domains, like social networks or ecological systems."
This type of emergence is essential for researchers looking at systems where individual elements are quite simple but together create complex outputs, such as swarm robotics or decentralized decision-making processes.
Strong Emergence
Strong emergence posits that new properties and behaviors arise that fundamentally cannot be reduced to the properties of the parts that make them up. In other words, the whole is distinctly more than the sum of its parts. This idea challenges traditional reductionist perspectives, suggesting that certain systems possess qualities that cannot be predicted, even with full knowledge of their individual components.
A classic example is consciousness. The behavior of neurons doesn’t fully account for the subjective experience of thought, emotion, or awareness. This gap in understanding illustrates the crux of strong emergence, where phenomena exist that are irreducible to simpler elements.
Some characteristics of strong emergence include:
- Irreducibility: Complex properties cannot be derived from the interaction of simpler components.
- Novelty: New behaviors manifest that offer insights beyond reductionist explanations.
- Qualitative leap: The transitions from one state to another often involve unpredictable shifts, akin to transformations found in phase changes of matter.
Strong emergence calls for interdisciplinary approaches, hinting at deeper connections between science, philosophy, and the human experience itself.
Global vs. Local Emergence
The distinction between global and local emergence helps clarify the scale at which emergent properties manifest. Global emergence relates to phenomena that arise from vast interactions across a system, while local emergence pertains to changes that occur within a limited scope or subsection of the system.
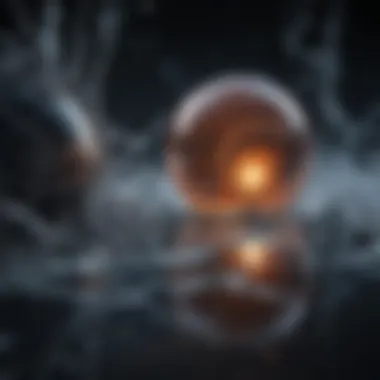
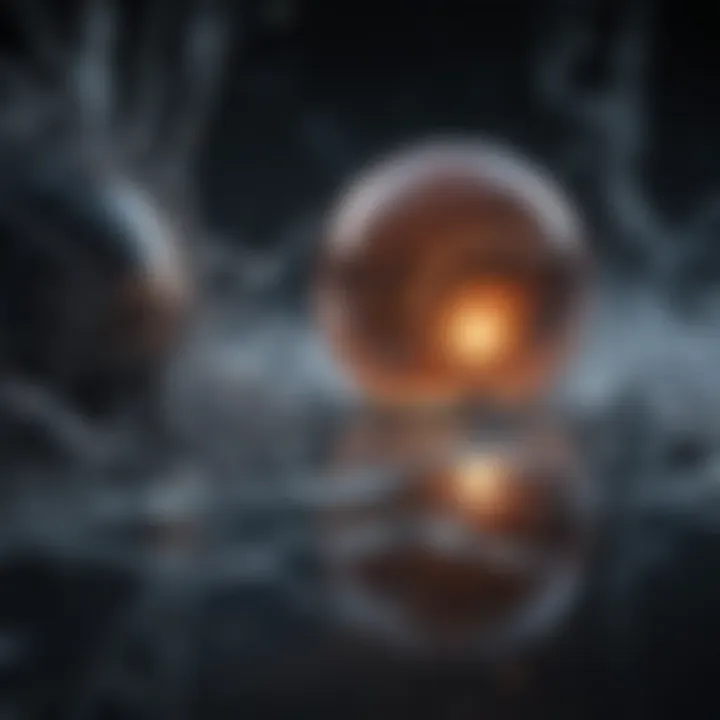
For example, in urban dynamics, global emergence could be seen in the patterns of traffic flow across an entire city, addressing how interactions among numerous drivers lead to traffic jams or smooth transit. Conversely, local emergence could analyze the behavior of a small group of cars navigating a particular intersection during peak hours.
Understanding these distinctions aids researchers and practitioners in determining how and where emergent properties can be predicted or manipulated. Consider these aspects:
- Scale of Interaction: Global emergence often requires a broad view, while local emergence can be analyzed within confined parameters.
- Scope of Influence: The impact of individual components is felt differently at various levels, influencing how we apply solutions in fields such as ecology or sociology.
- Interdisciplinary Insight: Problems in areas like climate change could benefit from recognizing both local adaptations (specific communities) and global shifts (planetary impacts).
Culmination
Recognizing and analyzing the different types of emergence provides a framework for interpreting complex systems. By distinguishing between weak and strong forms, as well as understanding global versus local perspectives, researchers in various fields can equip themselves to tackle the myriad challenges posed by emergent phenomena. This nuanced understanding fosters a deeper appreciation for how the interplay of components results in the fascinating complexities we observe in the world around us.
Mechanisms of Emergence
Understanding the mechanisms of emergence is like peeling an onion; each layer reveals deeper insights into how complex systems function. This part of the article delves into the nuts and bolts of what makes emergence possible—specifically focusing on the dynamics of interactions and relationships among components, as well as the inherent complexity and non-linearity that characterize many systems. By examining these mechanisms, we can appreciate how individual parts come together to produce unexpected properties and behaviors, pushing the boundaries of our comprehension in scientific contexts.
Interactions and Relationships
At the heart of emergence lies the idea that interactions among components are crucial. Think of a bustling city. Each person, vehicle, and building doesn’t just sit idly; instead, they interact, creating a living tapestry of behaviors—from traffic patterns to social dynamics.
In scientific study, these interactions can vary widely:
- Biological systems: For instance, in an ecosystem, the relationship between predator and prey drives population dynamics, leading to stabilizing oscillations that can’t be predicted by examining species in isolation.
- Social networks: Social media illustrates how information spreads; one tweet can cascade through networks, resulting in viral phenomena, even when each interaction seems trivial on its own.
“It’s not the parts, but the relationships that breathe life into systems.”
These examples. show how interactions within intricate systems shape emergent outcomes. By studying these dynamics, researchers gain insights into not only how systems function, but how they adapt, evolve, and sometimes, fail.
Complexity and Non-Linearity
Moving onto the next puzzle piece: complexity and non-linearity. In many systems, small changes can have disproportionately large consequences—this is the essence of non-linearity. Consider how a single decision can lead to unforeseen outcomes in a person's life or how a minor shift in temperature can shift an entire climate system.
Complexity comes into play when diverse elements come together, each contributing to a richer web of interactions. Here’s what to keep in mind:
- Emergent properties: These are often seen in weather patterns, where numerous atmospheric conditions lead to phenomena like storms—something that can’t be explained simply by looking at air pressure by itself.
- Feedback loops: Certain systems exhibit feedback mechanisms, such as in economics where rising prices can spur speculation, driving prices even higher.
This non-linear and complex interplay makes predicting outcomes challenging, if not sometimes impossible. Understanding this complexity thus provides deeper insights into how systems not only maintain but also transform their identities over time.
In summary, grasping the mechanisms of emergence lays the groundwork for appreciating how various systems—be they biological, physical, or social—function and evolve. It's this multilayered approach that allows for a more nuanced understanding of the world around us.
Emergence in Physics
Emergence in physics stands as a significant pillar in comprehending how abstract theories translate into tangible phenomena. This section digs into two core areas where emergence manifests profoundly: quantum mechanics and relativity, weaving a narrative that illustrates how fundamental laws lead to complex, emergent behaviors.
Quantum Mechanics and Emergence
Quantum mechanics, often dubbed the bedrock of modern physics, exhibits emergence in a bewildering way. At the micro level, particles behave in ways that challenge the intuitive notions of classical physics. Take entanglement, for instance. When two particles become entangled, the state of one directly influences the state of the other, regardless of the distance separating them. This intricate relationship does not conform to the simple sum of its parts. Researchers grapple with the implications of such behavior, pondering whether this entanglement hints at a deeper reality—a level of complexity that eludes our current understanding.
Emergence here suggests that properties like coherence and superposition arise from interactions at the quantum level. While certain behaviors can be derived from quantum laws, the rich tapestry of phenomena observed in systems composed of multiple particles, such as superconductivity, goes beyond mere particle interactions. This invites a broader examination of how hybrid systems manifest unique characteristics only observable at larger scales, a vital aspect for experts delving into quantum field theory.
Relativity and Spacetime
Turning our gaze towards relativity reveals yet another layer of emergence in physics. Einstein's theory fundamentally reshaped our comprehension of spacetime and gravity. Instead of viewing space and time as static backdrops, relativity presents them as dynamic entities interwoven by matter and energy. Here emerges the concept of curvature; mass tells spacetime how to curve, and curved spacetime tells mass how to move. This is not just a neat relationship; it signifies that various gravitational effects arise from the collective interactions between mass and the fabric of spacetime itself.
Emergent properties in relativity highlight how gravitational fields manifest from the collective behavior of masses rather than merely the influence of individual entities. The phenomenon of black holes serves as a stark example; they arise from the gravitational collapse of massive stars, showcasing how localized interactions can yield profound, emergent consequences in the cosmic landscape. The implications of this emergence stretch beyond just physics, leading to vital inquiries in cosmology and the study of the universe's structure.
"Emergence shows us that the whole can indeed be greater than the sum of its parts, especially at the intersections where classical laws meet quantum realities."
In these explorations, physicists confront the essence of reality itself, wrestling with concepts that blur the lines between order and chaos, simplicity, and complexity. Understanding emergence in these contexts not only enriches our grasp of physical laws but fosters a curiosity that drives further inquiry in the realms of science and mathematics.
Emergence in Mathematics
Mathematics often serves as the backbone for understanding emergence. Through abstraction and modeling, it provides a framework within which complex phenomena can be analyzed rigorously. In this section, we will delve into two crucial areas where emergence plays a significant role: topology and game theory. Both of these areas showcase how mathematical theories can reveal unexpected properties and behaviors in systems that seem simple at first glance.
Topology and Complex Systems
Topology, often regarded as the study of shapes and spaces, offers a unique lens through which we can understand the emergent properties of complex systems. Unlike traditional geometry, which concerns itself with specific measurements, topology focuses on the qualitative properties that remain unchanged under continuous deformations.
For instance, think about a coffee cup and a doughnut. Topologically, these two shapes can be considered equivalent since one can be transformed into the other without cutting or gluing. This concept plays a vital role in various fields like robotics and material science, where understanding the underlying structure of a system is key to predicting its behavior.
Emergent phenomena in topology tell us about how systems can undergo phase transitions or collective behaviors that cannot be straightforwardly predicted from their individual components. For instance:
- Shape Memory Alloys: In materials science, certain alloys can change shape in response to different temperatures. This behavior emerges from the underlying molecular structure and has applications in robotics and actuation systems.
- Network Theory: In social networks, the topology determines how information flows. The relationships between individuals can lead to emergent properties such as trends or fads that seem to arise spontaneously from the group dynamics.
"Topology provides the vocabulary to describe how complex systems interact and form behaviors that are greater than the sum of their parts."
Game Theory and Strategic Interactions
Game theory presents another fascinating vantage point for understanding emergence, particularly in social sciences and economics. It concerns itself with the strategic interactions between rational decision-makers. Here, emergence manifests through the dynamics of cooperation and competition, where the choices made by individuals lead to unexpectedly complex outcomes.
For instance, consider the famous Prisoner's Dilemma. Two individuals can either cooperate or betray each other. The emergent behavior of this game shows that although the rational choice for each player is to betray, mutual cooperation leads to a better outcome for both. This paradox illustrates how individual decisions in interactive contexts can lead to broader social phenomena—an emergent property.
Moreover, concepts in game theory extend to various fields:
- Market Competition: Firms competing over market share often create unexpected market dynamics, including price wars or monopolistic behaviors that are emergent from their interactions.
- Evolutionary Biology: Cooperative strategies in populations can drive the evolution of traits that benefit the group, again showcasing the emergence of complex behaviors from simple rules of interaction.
The beauty of emergent behavior in game theory lies in how groundwork strategies can form complex layers of social interaction and give rise to collective behaviors that are not attributable to any single actor—or strategy in play.
Ultimately, the study of emergence in mathematics highlights the importance of interconnectedness. Whether through the shapes we examine in topology or the strategic decisions analyzed in game theory, mathematicians have tools to explore phenomena that arise from perceived simplicity. Such explorations push the boundaries of our understanding, drawing connections that resonate across various fields and applications.
Emergence in Life Sciences
Emergence in life sciences offers crucial insights into how complex organisms and ecosystems shape themselves beyond the sum of their parts. This branch of study reveals not just individual biological entities but also their interwoven networks that respond dynamically to different stimuli. The emphasis here is on understanding how unique traits and behaviors arise when biological elements interact in varied conditions. Recognizing emergence provides us with the tools to explore adaptations, ecological relationships, and the intricate dance of life itself.
Biological Organization and Adaptation
At the heart of biological emergence lies the organization of life forms. Living organisms, from single-celled bacteria to complex mammals, showcase properties that don't merely sprout from their individual anatomical or genetic components. Biological organization refers to the patterns and hierarchies that characterize living systems. For instance, think about how ants work together. A single ant follows basic instincts, yet when they function as a colony, they display sophisticated behaviors like foraging, building complex nests, and forming defense strategies against threats—none of which can be attributed to any lone ant's capabilities.
This leads us into adaptation—the ability of organisms to modify their behaviors and physiological processes in response to their environments. A classic example would be the Darwin's finches found in the Galápagos Islands, which exhibit beak variations that enable them to exploit different food sources depending on environmental challenges. These adaptations emerge not merely through genetic evolution but through population interactions, ecological pressures, and resource availability. The emergent traits in these species are essentially a testament to life's intricate organization, revealing how structures and systems collaborate to navigate challenges.
Ecosystem Dynamics
When we elevate our focus from individual organisms to entire ecosystems, the concept of emergence becomes even more pivotal. Ecosystem dynamics refer to the interactions between living organisms and their physical environment. This interconnected web reflects how entities contribute to stability, resilience, and progression within their habitats.
Consider a wetland ecosystem. It is not just a collection of plants, animals, and microorganisms, but represents a dynamic system where water, soil quality, nutrient availability, and biotic interactions all come into play. Through the process of nutrient cycling and energy flow, the behaviors and roles of individual species create emergent properties like biodiversity and ecosystem resilience. For example, the presence of predators can regulate herbivore populations, which in turn impacts vegetation growth and health, demonstrating a cascading effect where the actions of one group influence the broader community.
"Emergence in life sciences offers a lens through which practitioners can perceive biological complexities beyond reductionist approaches."
Ultimately, the study of emergence within life sciences not only expands our knowledge but also shapes our approach to conservation, biodiversity, and healthcare. As we delve into these extensive networks, we begin to appreciate the significance of holistic observation and integrated management of biological systems—ushering us into a realm where understanding emergence can have profound implications for our interactions with nature.
Applications of Emergence
Emergence is not just a theoretical construct; it finds its way into practical applications that resonate across various fields. A comprehensive understanding of emergence can enhance innovation and problem-solving in disciplines ranging from technology to biology. By appreciating how collective behaviors and properties arise from simple rules and interactions, researchers and professionals alike can harness the power of systems thinking.
The significance of applications of emergence cannot be overstated. It plays a crucial role in developing frameworks that drive advancements in numerous sectors. Considerations around the potential and limitations of emergence demand a multi-faceted approach, which can often lead to unexpected benefits. This section will explore two significant domains: artificial intelligence and social dynamics.
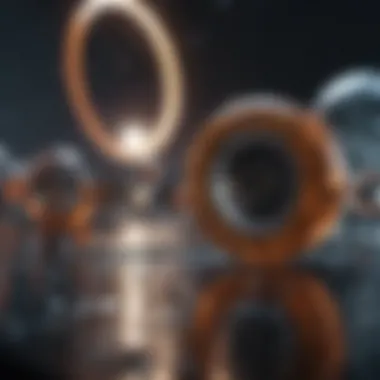
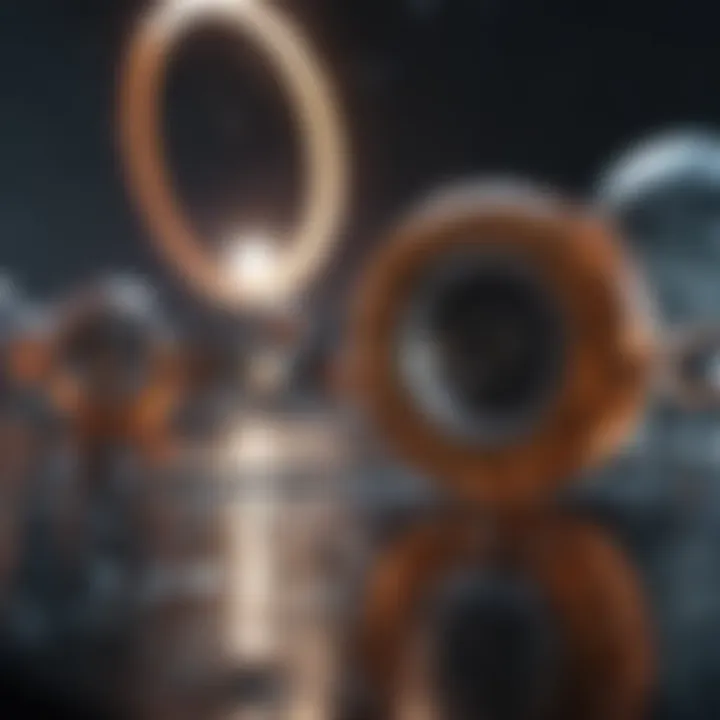
Artificial Intelligence and Machine Learning
In the realm of artificial intelligence (AI) and machine learning, emergence manifests in the patterns that arise from complex algorithms and vast data sets. One cannot ignore how simple individual algorithms can lead to sophisticated behaviors, much like the way ant colonies function seamlessly through decentralized communication. For instance, neural networks can model human cognition, showcasing how interconnected nodes contribute to emergent decision-making processes.
- Predictive Analytics: AI applications utilize emergence to analyze historical data, drawing patterns that were previously unclear. This is seen in predictive modeling, where emergent data trends help businesses anticipate consumer behavior.
- Adaptive Learning Systems: Machine learning systems, particularly those grounded in reinforcement learning, grow in effectiveness over time through interactions with their environments. They illustrate how emergent strategies can evolve based on feedback, optimizing performance without pre-defined rules.
Machine learning's inherent nature to adjust and learn from emergent phenomena enables it to solve complex problems in ways traditional programming cannot. The implications are broad, from developing personalized recommendations to medical diagnostics.
"In AI, emergent behavior is the key to unlocking autonomous systems that can adapt and respond to real-world problems in real time."
Social Dynamics and Collective Behavior
Emergence profoundly impacts social sciences, particularly in understanding collective behavior. Studying how individuals interact within societal frameworks reveals insights into phenomena such as crowd dynamics, social movements, and the diffusion of innovations.
- Crowds and Movements: The behavior of crowds can be seen as emergent in nature. When individuals congregate, spontaneous order often forms, leading to unique dynamics that cannot be predicted merely by examining individual behavior. This notion of 'the whole being greater than the sum of its parts' feeds into how researchers analyze social phenomena.
- Policy Development: Understanding emergence in social dynamics is crucial for effective policy-making. Policymakers can benefit from recognizing how community actions can lead to unpredicted outcomes, helping frame strategies that cater to collective needs.
The study of social dynamics through the lens of emergence not only provides clarity but sparks innovation in addressing societal challenges. By using systemic approaches, social scientists strive to understand and influence collective behavior, emphasizing the intricate interplay of individual actions and communal results.
In summary, the applications of emergence in both AI and social dynamics underline the potential impact of interconnected behaviors and systems on real-world issues. Recognizing these emergent properties can lead to significant strides in understanding and shaping the future.
Challenges in Understanding Emergence
Navigating the conundrum of emergence requires us to grapple with a myriad of intellectual hurdles. It's certainly no walk in the park - the complexities span various disciplines, each leaving its own imprint on our understanding. From scientific to philosophical paradigms, the journey to grasp what emergence truly is often seems like trying to catch smoke with bare hands. Laying out these challenges can guide us toward clarity and uncover the potential benefits that arise from addressing them.
Definitional Ambiguities
One of the first speed bumps in comprehending emergence is the lack of a universally accepted definition. Here’s the kicker: what one scholar views as emergent behavior, another might classify simply as a result of interaction or complexity. It’s a bit like calling a cat a dog. To some, these definitions can morph depending on context, leading to classifying the same phenomena as emergent in one study and not in another. This ambiguity can create unnecessary confusion, sidelining productive discussions in both research and education.
Measuring Emergence
The second substantial challenge lies in the quantification of emergence. Think about it: how does one accurately measure something that, by its very nature, transcends individual components? Establishing metrics is like trying to pin down a shadow in a dimly lit room.
- Establishing Parameters: For example, when dealing with emergence in physics, how do physicists agree on what constitutes an emergent property within quantum mechanics? Similarly, in social sciences, measuring collective behavior can lead to conflicting interpretations unless researchers come up with a solid set of parameters for comparison.
- Tools at Our Disposal: Efforts have been made to develop tools and methodologies that can objectively capture emergent behaviors, often involving statistical modeling, network analysis, or even simulations. However, the inconsistency in approaches can render comparisons across studies complex and even frustrating.
Moreover, researchers must remain vigilant about the limitations and biases inherent in their chosen measurement tools. If researchers aren't cautious, they might overlook crucial emergent phenomena simply because they used the wrong lens to examine their systems.
"The difficulty in defining and measuring emergence points to our need for clear, consistent frameworks to facilitate thoughtful exploration and foster collaboration between disciplines."
Future Directions in Emergence Research
Emergence research stands at a fascinating crossroads. As more scholars poke into the intricacies of complex systems, understanding emergence becomes critical. The significance of this topic cannot be overstated; it has implications not just for theoretical exploration but also for practical applications that span across various fields.
Interdisciplinary Approaches
Emergence is inherently complex. Tackling it requires insights from diverse areas like physics, biology, sociology, and computer science. One way forward lies in fostering interdisciplinary approaches. By blending methodologies and viewpoints, researchers can distill more nuanced understandings of patterns in complexity. For instance, a physicist may work with a biologist to unravel how particle interactions lead to cellular organization. This cross-pollination of ideas can unveil concepts that more traditional singular domains might overlook.
Moreover, interdisciplinary collaboration can level up scientific literacy among students. This educational aspect should not be brushed off. Whether in a classroom or a research setting, engaging multiple disciplines cultivates creativity, questioning, and innovation. Learners gain a holistic view, not boxed into the confines of their field, but liberated to explore the connections in their inquiry into emergence.
Technological Innovations
On another front, advancements in technology are opening up new avenues for emergence studies. Tools like machine learning and advanced simulations have the potential to revolutionize how researchers analyze complex systems. For instance, algorithms designed for data analysis can uncover patterns that humans might miss. An example of this is using neural networks to simulate how traffic patterns emerge in urban settings. By feeding vast amounts of real-time data into these systems, researchers can start to predict congestion and perhaps even design smarter cities in the future.
Additionally, enhanced computational power allows for increased modeling and experimentation. Simulations can run scenarios that might be impractical or impossible to observe in real life. These technological advancements enable researchers to engage deeply with the theories of emergence, rigorously testing hypotheses and refining understandings in a way that was not previously feasible.
"To grasp the full extent of emergence, the blend of diverse minds and groundbreaking tools is indispensable."
In summary, future directions in emergence research highlight the necessity of interdisciplinary collaboration and leverage technological innovations. Together, these facets are set to unravel further complexities hidden within the fabric of our understanding. The interplay between various fields and the evolution of technological capabilities will significantly shape what the future might hold for the study of emergence, encouraging expansive thinking and innovative solutions.
Case Studies of Emergence
Case studies provide a concrete way to observe the concept of emergence in action. They allow researchers to study real-world examples where complex systems exhibit behaviors and properties that cannot simply be understood by examining their individual parts. These case studies often illustrate the dynamic interactions that lead to emergent phenomena, making the concept of emergence more relatable and tangible. They can also highlight the importance of context, offering insights into how emergent properties can vary based on different conditions and backgrounds. By exploring case studies, readers can appreciate the multifaceted nature of emergence and how it manifests in various scientific contexts.
The Internet and Information Flow
The Internet stands as a prime illustration of emergence, showcasing how individual actions can lead to collective behavior with wide-reaching implications. At its core, the Internet is a network. It connects countless devices, creating a web of information flow. The behaviors we observe, such as viral trends or the way information spreads during crises, emerge from the interactions of millions of users with their own motivations and goals.
Key aspects of this case include:
- Decentralization: Unlike traditional media, where information flows in a top-down manner, the Internet allows for a more decentralized structure, leading to diverse perspectives and outcomes.
- Network Effects: As more users connect, the value and accessibility of information grow, creating a richer environment for emergent behavior.
- Feedback Loops: Changes in user behavior can lead to changes in information availability, which can then influence further behavior, creating a cycle that enhances emergence.
For instance, during a natural disaster, information can spread much faster through social media than through traditional news outlets. Individuals tap into their networks to relay crucial updates, forming a collective response that is greater than the sum of its parts. This phenomenon can be observed during events like the Fukushima disaster when real-time updates on safety and radiation levels spread through platforms like Twitter, demonstrating how the flow of information can lead to emergent decision-making and behavior.
Biological Networks and Systems Biology
In the realm of biological sciences, emergence plays a pivotal role in understanding how complex life forms function. Biological networks, comprised of genes, proteins, and cellular components, can exhibit properties that go beyond mere sum of their interactions.
Consider the concept of systems biology. This interdisciplinary field focuses on the study of complex interactions within biological systems. It looks into how individual elements work together to result in behaviors and properties that are not present in isolated units.
- Cellular Interactions: Within a multicellular organism, different cells communicate and collaborate. This interaction can lead to tissue formation, organ function, and ultimately, the behaviors of the entire organism.
- Ecosystem Dynamics: At a higher level, ecosystems display emergent behavior through predator-prey relationships, nutrient cycling, and symbiotic partnerships. Species relationships often lead to stability or instability in ecological communities, demonstrating how interconnectedness contributes to overall system health.
- Disease Network Models: Emergent phenomena also arise in understanding diseases. The spread of an infectious disease can be modeled through networks of interactions, leading to insights on how to counteract outbreaks effectively.
Understanding emergence in biological networks is crucial for advancements in medicine, environmental science, and biotechnology. Studies like the Human Connectome Project illustrate the importance of recognizing how the brain's interconnected neurons create conscious experience—a property not found in any single neuron. By analyzing these intricate networks, researchers uncover the foundations of life itself, emphasizing that emergent properties lie at the heart of biological understanding.
The Role of Observation in Emergence
In the study of emergence, observation plays a crucial role in elucidating how complex systems come together to form properties and behaviors that are more than the sum of their parts. Understanding emergence cannot be fully realized without acknowledging the observer's influence on what is being perceived. Observational practices are not passive; they shape the narratives and theories through which we interpret emergent phenomena.
Phenomenological Perspectives
From a phenomenological standpoint, observation is not merely about physical sight but involves the entire experience of perceiving. Each individual’s background, biases, and emotional state can color their interpretation of what they observe. For example, consider two biologists studying an ecosystem. One may focus on the interactions within a food web, while the other may concentrate on the adaptive behaviors of individual species. Their distinct perspectives could lead to different conclusions about the system as a whole.
An interesting illustration is the examination of ant colonies. While one observer might see a random chaos of movement, another might perceive a coordinated organization guided by pheromone trails. This divergence is pivotal; it emphasizes that the observer's context and approach can reveal or obscure aspects of emergent behavior. Thus, phenomenological perspectives remind us that our observations can frame the way we understand complexity.
Observational Limitations
However, it’s also essential to confront the limitations inherent in observational methodologies. One of the main challenges is that some aspects of emergence cannot be directly observed, leading to gaps in our understanding. For instance, in quantum mechanics, phenomena such as entanglement present challenges that resist standard observation due to their very nature.
Observational tools and methods often dictate what can be seen or measured. In complex systems, many interactions occur at scales, both temporal and spatial, that escape our current technological capabilities. These limitations pose risks of incomplete analyses and conclusions drawn from insufficient data.
Moreover, the act of observation itself can influence the systems being studied. This connection is famously illustrated in Heisenberg's Uncertainty Principle, which states that the act of measuring certain pairs of physical properties—like position and momentum—affects the outcome.
"The observer is involved in the process of observation itself, and this involvement has profound implications for the study of emergence."
Consequently, researchers must remain cautious and critical about the interpretations arising from their observations. Taking these limitations into account, scholars have proposed a more integrated approach that combines both qualitative and quantitative methodologies, ensuring a holistic view of emergent phenomena.
Through reflective observation and acknowledgment of its limitations, researchers stand a better chance of capturing the true essence of emergent systems, developing theories that resonate more deeply with the complexities of the world around us. In summation, the interplay between observation and emergence reflects a nuanced understanding vital to advancing this field of study.
Conceptual Challenges with Emergence
Emergence, while a fascinating topic within science, faces several conceptual hurdles that must be navigated for a clearer understanding. Exploring these challenges enhances our grasp of complex systems and the intricate behaviors they exhibit. Understanding the implications of emergence requires a careful examination of not only the phenomena themselves but also the underlying philosophical frameworks. This section focuses on two primary challenges: reductionism versus holism and the interconnected nature of systems.
Reductionism vs. Holism
At the heart of many discussions about emergence lies the ongoing debate between reductionism and holism. Reductionism posits that complex systems can be understood by breaking them down into their individual parts. This foundational assumption serves as a long-standing method in both natural and social sciences. For example, in biology, understanding a single gene can lead to insights about larger biological processes. However, this doesn't always paint a full picture.
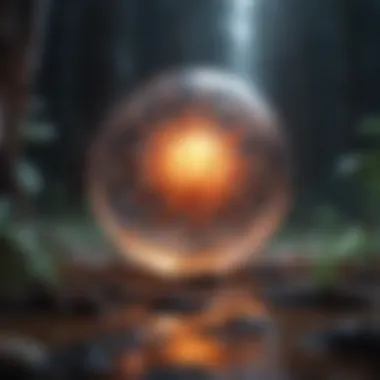
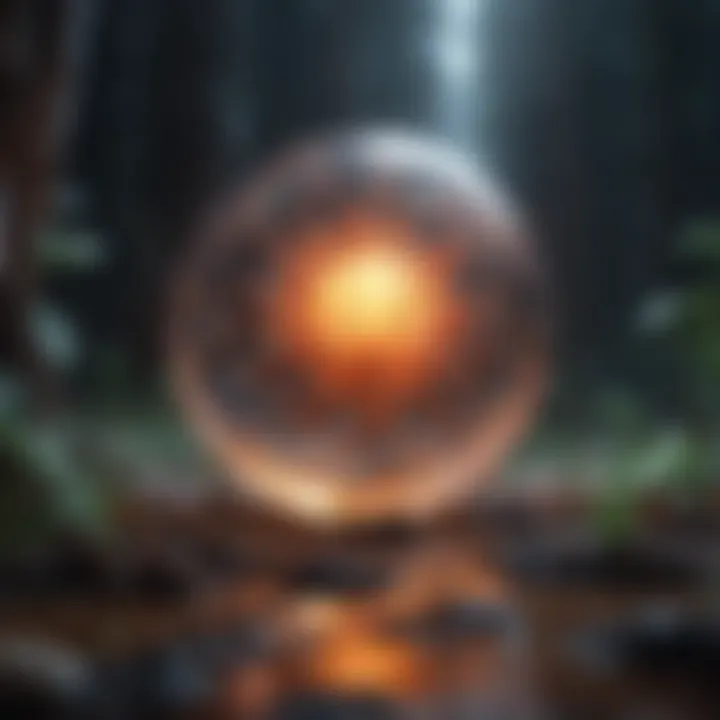
On the flip side, holism argues that the properties of a whole system cannot simply be deduced from its parts. Consider a football team: analyzing individual players' statistics won’t necessarily predict the outcome of a game. The team’s synergy, strategies, emotional dynamics, and external factors collectively contribute to its performance.
This tension underscores the difficulty in defining emergent properties. How can we fully appreciate phenomena like consciousness or ecological dynamics without acknowledging that their attributes arise not merely from individual components but from their interplay? The challenge deepens when we observe complex behaviors that only manifest when components unite within a system. Observing phenomena like flocking behaviors in birds or the market fluctuations in economics highlights this intricate dance between reductionism and holism.
"Emergence invites us to reconsider how we define understanding itself. Is it only a matter of knowing the parts, or do we need to behold the whole?"
Interconnectedness of Systems
The interconnectedness of systems presents another layer of complexity in discussions about emergence. In nature, entities don’t operate in isolation; rather, they are part of larger networks of relationships. To recognize emergence is to acknowledge these relationships and how they foster behaviors that cannot be attributed simply to individual actions.
This interconnectedness manifests across various disciplines. In ecology, for instance, the dynamics of a population can be heavily influenced by both biotic factors, like predators and competition, and abiotic factors, such as climate and habitat availability. These interrelations create patterns of behavior and responses that are emergent properties of the ecosystem as a whole.
Moreover, in social sciences, the behavior of groups can lead to emergent phenomena like collective consciousness or social movements. Individual choices lead to patterns that cannot be understood solely by looking at one person's actions. In a sense, understanding emergence here means viewing systems as holistic, interdependent constructs rather than simple summations of their parts.
As researchers and practitioners explore these complexities, they must grapple with the implications of interconnectedness in their work. Concepts of feedback loops, system boundaries, and external influences must be considered. This notion pushes the boundaries of traditional scientific disciplines and calls for more integrative methodologies.
In dealing with these conceptual challenges, one can appreciate the richer tapestry of reality that emergence unveils. Understanding emergence not merely as a phenomenon but as a holistic perspective fosters cross-disciplinary collaboration and innovation, pointing toward new areas of inquiry and application.
Ethical Implications of Emergence
The concept of emergence opens a Pandora’s box when it comes to ethical considerations. As we seek to unravel the workings of complex systems in various fields, we must not overlook the moral responsibilities tied to our discoveries. Understanding emergence is not merely an academic pursuit; it intersects with the ethical principles that govern research, policy-making, and ultimately, societal impacts. Recognizing these ethical implications can help scientists and policymakers navigate the murky waters where knowledge and responsibility meet.
Emergence, at its core, relates to how new properties or behaviors arise from the interactions within a system, often leading to phenomena that cannot be easily predicted or managed. This unpredictability introduces several ethical challenges that demand careful thought and analysis. Here, we will delve into two crucial facets of these implications: social responsibility in research and the role of emergence in policymaking.
Social Responsibility in Research
When we probe into emergent behaviors, especially in fields like artificial intelligence or biotechnology, it becomes imperative to reflect on the societal consequences of our findings. Researchers often find themselves holding the keys to powerful technologies, which, if misused, could lead to unforeseen harm.
The responsibilities of researchers can be summarized through the following points:
- Informed Consent: Many emergent systems, especially in medical research, must prioritize informed consent. Stakeholders need clarity on how their data or biological materials will be used.
- Safety and Security: Developers of emergent technologies must consider the potential misuse of their inventions. For instance, advancements in AI may aid societal progress but could also facilitate malicious activities.
- Public Awareness: There is a moral obligation to educate the public about the implications of emergent technologies, fostering a more informed community prepared for decision-making.
To illustrate, the development of AI-driven systems has sparked debates about accountability and bias. Studies show that biases can inadvertently emerge from training data, leading to discriminatory algorithms. Thus, ethical research in these fields demands procedures that mitigate risk while maximizing benefit.
Emergence and Policy Making
The intersection of emergence and policy-making highlights how new insights can drive legislative changes, but these changes must be guided by ethical frameworks that reflect societal values. As emergent phenomena demand adaptive systems thinking, policymakers must consider both the short and long-term implications of their decisions.
The following aspects are crucial:
- Adaptive Legislation: Policies need to evolve alongside scientific development. This means being prepared to revise regulations as new emergent behaviors are understood.
- Stakeholder Engagement: Inclusive dialogue with experts and the community helps capture varying perspectives, ensuring that decisions reflect the collective interest.
- Balancing Innovation and Caution: Policymakers face the challenge of nurturing innovation while protecting public interests. This requires a nuanced understanding of the emergent properties at stake and the potential risks involved.
Emergence has stirred discussions on climate change and public health, where complex interdependencies call for strategic policies that can adapt as new data emerges. For example, emergent patterns in epidemiology, as observed in the COVID-19 pandemic, necessitated quick adaptations in health policy, underscoring the need for ethical frameworks that prioritize public welfare over scientific pursuits.
"To err is human; to forgive, divine. But when it comes to emergent behavior in systems, understanding the implications is essential to avoid missteps that can affect countless lives."
Interplay between Order and Chaos
The concept of emergent phenomena can often be visualized as a dance between order and chaos. To understand emergence in scientific contexts, one must explore how these two forces interact. This interplay can help elucidate the dynamics of complex systems, as well as shed light on the properties that arise when simpler elements come together.
In various fields, the balance between order and chaos manifests in distinct ways. For instance, in sociology, social structures can emerge from chaotic interactions of individuals, yet exhibit patterns reminiscent of purposeful organization. In nature, chaotic systems under certain conditions can lead to remarkably organized outcomes, demonstrating resilience and adaptability. Recognizing this balance is key to grasping how systems develop their unique characteristics.
"Emergence often resides at the junction where chaos and order exist together. It's a delicate balance that drives complexity and innovation in systems."
Chaos Theory and Emergence
Chaos theory offers valuable insights into how small changes in initial conditions can drastically alter the outcome of a system. This concept can be illustrated through the butterfly effect, where the flapping of a butterfly’s wings might eventually lead to a hurricane elsewhere. In terms of emergence, chaos theory emphasizes how unpredictable interactions can lead to new, emergent properties.
Consider weather systems as an example. While individual atmospheric events may seem chaotic, they often coalesce into recognizable patterns such as storms or calm spells. These patterns cannot be deduced merely from their components—temperature, humidity, wind speed—but emerge through the interactions of countless factors. Thus, chaos as a facilitator of emergence highlights how systems can evolve, adapt, and exhibit surprising behavior through nonlinear interactions.
Self-Organization in Complex Systems
Self-organization refers to the process by which a structure or pattern emerges in a system without a central or external guiding influence. This phenomenon is particularly important in understanding how complexity arises naturally.
One of the most prominent examples of self-organization can be seen in ant colonies. Despite individual ants following simple behavioral rules, complex colony structures, communication networks, and foraging patterns emerge. Such behaviors aren’t dictated by a leader ant or any formal structure, but arise from local interactions between the ants themselves. This shows how order can emerge from chaos without centralized control.
Similarly, in physical systems, like the formation of patterns in lipid molecules in water, self-organization occurs as molecules interact based on local rules, leading to structured arrangements. This process illuminates how self-organization can serve as a mechanism for emergence across disciplines, from biology to physics.
In summary, grasping the interplay between order and chaos is crucial to understanding emergence. The dynamics of complex systems reveal a profound relationship where chaos can foster new order, and self-organization can lead to surprising new structures. These elements together highlight the beauty and complexity of emergent phenomena.
Systemic Thinking and Emergence
Systemic thinking plays a crucial role in comprehending emergence, as it enables the examination of complex systems as wholes rather than mere collections of parts. This approach emphasizes the interdependence of elements within a system, fostering an understanding that behaviors and patterns emerge from relationships and interactions. By adopting a systemic perspective, researchers can navigate the intricate dynamics that govern various scientific disciplines.
Holistic Approaches to Emergence
When we talk about holistic approaches to emergence, we stress the importance of seeing the bigger picture. Emergence is not merely a linear result of individual components; it often arises from intricate feedback loops and nonlinear relationships. In biology, for instance, the emergence of consciousness or complex behavior in organisms reflects how higher-level properties arise from the interactions of simpler components.
- Interconnectedness of Elements: In any system, the components are far from isolated. Think of an ecosystem—it’s a rich tapestry where each organism plays a role, affecting and being affected by others. This interconnectedness leads to dynamic behaviors that are often unpredictable.
- Multi-Disciplinary Insights: Holistic approaches encourage collaboration across fields—be it biology, social sciences, or engineering. By uniting different perspectives, researchers can uncover emergent properties that would’ve otherwise been overlooked. For example, studying traffic flow not just from a physics perspective, but also considering psychology and urban planning can yield deeper insights into city dynamics.
This approach often challenges the traditional reductionist view, which seeks to explain systems solely through their parts. As people begin to understand the limitations of reductionism, they increasingly appreciate the richness of holistic thinking.
Frameworks for Understanding Emergence
In grasping emergent phenomena, establishing effective frameworks is vital. These frameworks provide tools that aid in analyzing and predicting behaviors in complex systems. Various models and theoretical constructs can be employed to achieve this understanding:
- Network Theory: By framing systems as networks of interconnected nodes, researchers can visualize and quantify interactions. For example, social networks reveal how individual connections can drive collective behaviors, such as trends or movements.
- Agent-Based Modeling: This method simulates interactions of autonomous agents (individual entities) to observe how larger patterns emerge. These simulations often illustrate how simple rules governing agent behavior can lead to complex phenomena.
- System Dynamics: Developed for understanding the behavior of complex systems over time, this framework emphasizes stocks, flows, and feedback loops. It has applications ranging from ecology to economics, showing how changes in one part of a system can ripple through and affect the whole.
"Emergence isn’t about the individual parts; it’s about how they talk to each other. Well, that's where the magic happens."
By integrating these frameworks into systemic thinking, researchers can enhance their ability to dissect and interpret emergent phenomena in various scientific contexts. This interplay of holistic approaches and structured frameworks lays the groundwork for a deeper understanding of how complex systems behave and evolve.
Finale and Implications
The culmination of our exploration of emergence presents a rich tapestry of insights and implications that span diverse scientific domains. The understanding of emergence is not merely an academic exercise; it affects how we approach complex problems in various fields, from biology to artificial intelligence. The phenomenon serves as a reminder that the whole often exhibits properties that the individual parts cannot predict or replicate. This has profound impacts on the methodologies employed across disciplines.
In examining emergent properties, we understand that systems are inherently more than the sum of their individual elements. For instance, consider the behaviors of ant colonies. While each ant acts based on simple rules, their collective behavior results in sophisticated and adaptive solutions to environmental challenges. This principle of emergence tells us that in different scientific contexts, holistic approaches are frequently necessary for meaningful interpretations.
Key Implications of Understanding Emergence:
- Interdisciplinary Collaboration: Understanding emergence requires bridging gaps between disciplines. Researchers across fields must share knowledge to address common challenges more effectively.
- Innovative Solutions: Emergent properties often lead to novel strategies. For instance, biological systems exhibit mechanisms fine-tuned by evolution, inspiring innovations in technology and engineering.
- Ethical Considerations: As we acknowledge the complexity of systems, we also must confront the ethical implications. How do the emergent behaviors of humans in social contexts shape our interactions and governance?
Ultimately, awareness of emergence fosters a nuanced perspective on scientific research and its applications. By recognizing how interconnectedness and complexity operate in various systems, we can better navigate the challenges that arise in a world increasingly characterized by rapid change.
Synthesis of Insights
Through this article, we've drawn together various threads involving emergence and its multifaceted implications across scientific disciplines. Every section provided layers of understanding, illustrating how emergent behavior is not just an isolated concept; rather, it is a pervasive characteristic inherent in natural and artificial systems. Whether in ecology, physics, or social behavior, understanding these connections can drive new scientific inquiry and application.
- Core Insights:
- Understanding Complexity: Complexity underlies almost all systems that exhibit emergence, emphasizing that simple interactions can lead to profound outcomes.
- Behavioral Patterns: The patterns that arise from complex systems often defy simple explanation, necessitating a shift in how researchers approach problem-solving.
- Frameworks: Developing suitable frameworks to study emergence will aid in predicting how systems evolve and interact.
The synthesis highlights how interconnectedness plays a crucial role in the emergence of behaviors and properties that cannot be deduced simply from the components involved. This revelation not only enhances our understanding of existent systems but also enriches our capacity for anticipating future ones.
The Future of Emergence Studies
Looking forward, the study of emergence holds immense potential for transformative knowledge. As we continue to unravel the complexities innate in different systems, the directions in which emergence research evolves will likely include the following:
- Technological Integration: As technology continues to advance, particularly in AI and data analytics, we will better understand emergent phenomena through enhanced simulations and modelings, such as neural networks that mimic human learning processes.
- Increased Interdisciplinary Projects: As boundaries between scientific disciplines blur, collaborative projects aimed at tackling global challenges from multiple angles will become more frequent.
- Exploration of Socio-Ecological Systems: With the world's growing complications due to climate change and social dynamics, a focus on socio-ecological systems will uncover new insights about how these interrelated components emerge.