Understanding Black Hole Collisions: A Comprehensive Review
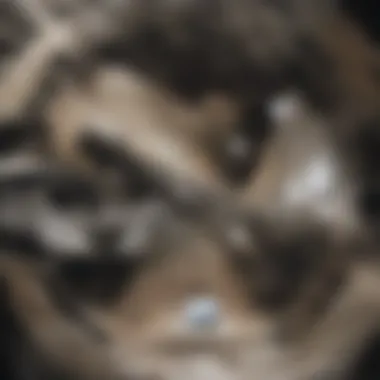
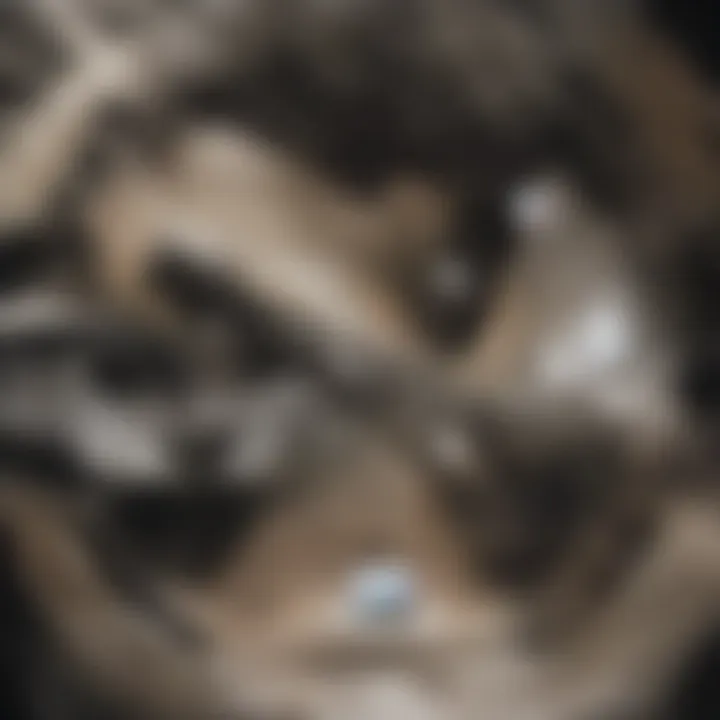
Intro
The study of black holes has captured the imagination of scientists and the public alike. Among the many intriguing aspects of black holes, their collisions present a particularly fascinating area of research. As two black holes spiral towards each other and eventually merge, they produce significant gravitational waves, which can be detected by advanced technologies such as LIGO and Virgo. Understanding these events sheds light on fundamental questions in astrophysics, cosmology, and the nature of spacetime itself.
Black hole collisions not only confirm predictions made by Einstein's theory of general relativity but also provide insights into the formation and evolution of these mysterious cosmic structures. The significance of studying these events cannot be overstated as they help answer queries about the behavior of matter under extreme gravitational conditions. The ability to observe and analyze these collisions expands our understanding of the universe, pushing the boundaries of human knowledge.
Given their importance, this article will explore the core findings from recent studies on black hole collisions, simplifying complex theories, and detailing the technologies used in these endeavors. Furthermore, it underscores how these phenomena reshape the landscape of physics and cosmology.
Prelude to Black Holes
The study of black holes is crucial within astrophysics. They represent extreme gravitational phenomena that challenge existing theories of physics. Understanding black holes can help explain universe's formation, evolution, and structure. Through this article, we will explore key aspects related to black holes, especially their collisions. Studying these collisions not only advances theoretical physics but also enables us to explore the universe on a grander scale.
Defining Black Holes
A black hole is a region in space where the gravitational pull is so strong that nothing, not even light, can escape from it. This leads to a point of singularity, where density becomes infinite and space-time curvature becomes extreme. Black holes form when a massive star exhausts its nuclear fuel and collapses under its own gravity. Their mysterious nature and behavior are intriguing for scientists and astronomers both.
The Types of Black Holes
Black holes are generally categorized into four different types. Each type has unique characteristics, formation processes, and roles in the cosmos.
Stellar Black Holes
Stellar black holes are formed from the remnants of massive stars after they explode into supernovae. The collapse leads to a core so dense that it creates a stellar black hole. This type is critical for understanding the lifecycle of stars. One key characteristic of stellar black holes is their mass, which typically ranges between 3 to tens of solar masses. They serve as excellent objects for study due to their relative abundance and proximity in the universe. The unique feature of these black holes lies in their potential to emit X-rays when they pull in matter from their surroundings. This can lead to spectacular observations that help in understanding their properties.
Supermassive Black Holes
Supermassive black holes are found at the centers of galaxies. They can have masses ranging from millions to billions of solar masses. These black holes play a significant role in galaxy formation and evolution. One key characteristic is their association with active galactic nuclei; they can significantly influence the dynamics of surrounding stars and gas. Their formation is still an area of active research, with theories suggesting they could form through the merger of smaller black holes or through direct collapse of massive gas clouds. A unique aspect of supermassive black holes is that they can affect the growth patterns of galaxies, and studying them helps us grasp the dynamics of cosmic structures better.
Intermediate Black Holes
Intermediate black holes are less understood compared to stellar and supermassive black holes. They have masses ranging between hundreds to thousands of solar masses. One key aspect is their potential formation pathways, which include the merging of stellar black holes in dense star clusters. Their unique position in the mass spectrum of black holes makes them fascinating for research. Studying them may provide insight into the early universe and bridge the gap between stellar and supermassive black holes. However, detection of these black holes remains complicated due to their elusive nature and limited observable phenomena.
Primordial Black Holes
Primordial black holes are hypothesized to have formed in the early universe, shortly after the Big Bang. They could vary greatly in size, from very small to massive. One key characteristic of primordial black holes is that they might not correlate with the formation of stars. This offers a different perspective on the nature of mass and gravitational interactions during the universe's formative years. Unique features include their potential as dark matter candidates, which makes them important in discussions about cosmic evolution and structure formation. However, they remain largely theoretical, as direct evidence supporting their existence is still lacking.
Theoretical Framework of Black Hole Collisions
The theoretical framework of black hole collisions is crucial in astrophysics. It combines principles from physics to explain how black holes interact. Understanding this framework helps researchers predict outcomes and analyze observational data. This section explores two main components: general relativity and the mechanics of collision events. Both provide context for subsequent discussions and illuminate how collisions shape our universe.
General Relativity and Black Holes
General relativity plays a key role in understanding black hole collisions. Proposed by Albert Einstein, this theory describes how massive objects warp space-time. Black holes, with their extreme mass and density, are perfect examples of this phenomenon. General relativity explains how black holes form, interact, and ultimately collide with one another.
The power of general relativity lies in its mathematical elegance. It yields predictions about gravitational waves, which are emitted during black hole collisions. These waves give insight into the nature of the universe, allowing us to test the theory itself. Observations of gravitational waves from events like the merger of two black holes validate aspects of this framework. Their detection marks a significant advancement in astrophysics and deepens our understanding of stellar evolution.
Mechanics of Collision Events
The mechanics of collision events encompass various processes that occur when black holes approach each other. This area of study helps us dissect the dynamics involved in a merger.
Orbital Dynamics
Orbital dynamics examines the motion of black holes before they collide. It looks at their orbits, relative velocities, and interactive forces acting on them. As black holes spiral toward one another, they lose energy through gravitational radiation. This phenomenon accelerates their approach, leading to a collision. The behavior of binary black holes serves as a good example, as it highlights unique orbital characteristics through different evolutionary stages.
A key characteristic of orbital dynamics is its predictive capability. Understanding how black holes interact permits astronomers and physicists to forecast potential outcomes from various configurations. This knowledge enhances our ability to interpret data from gravitational wave observations, thus bolstering our comprehension of these mysterious entities.
Energy Release
Energy release is a significant outcome of black hole collisions. During a merger, enormous amounts of energy are liberated in the form of gravitational waves and electromagnetic radiation. This release can be profoundly illuminative, shedding light on the processes governing black holes.
The key aspect of energy release is its variability in magnitude. Depending on the mass and spin of the colliding black holes, the amount of energy emitted may differ greatly. This variability ensures diverse observational signatures, crucial for scientists analyzing such events. However, capturing this energy release requires sophisticated detection and analysis methods, presenting a challenge for researchers.
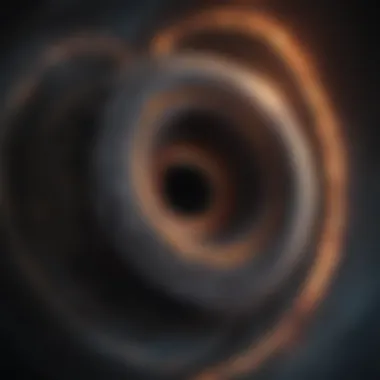
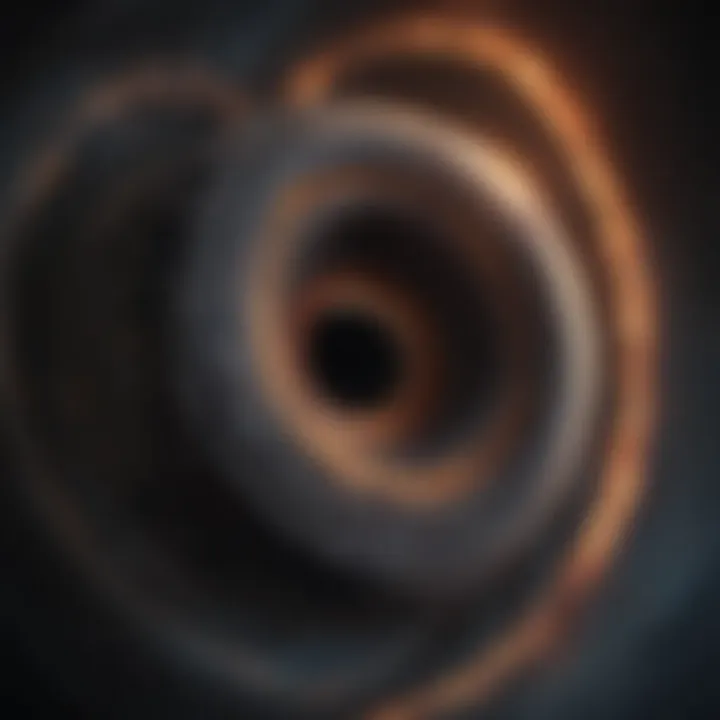
Final Merger Phase
The final merger phase is the climax of a black hole collision. It is the stage where two black holes coalesce into a single, larger black hole. This phase is marked by a dramatic release of gravitational waves, providing a unique signature that astronomers can detect.
The unique feature of the final merger phase lies in its complexities. Factors such as spin orientation and contact time significantly affect the outcome and the resulting waveforms. This intricacy poses challenges when attempting to model these events. However, understanding this phase is beneficial, as it can lead to insights about the formation of black holes and their roles in the universe.
In summary, the theoretical framework of black hole collisions interconnects general relativity and mechanics. This connection is fundamental in decoding the interactions, energy dynamics, and features of black hole mergers. Through comprehensive research in these areas, we can further our understanding of black holes and their impact on the cosmos.
Observing Black Hole Collisions
Observing black hole collisions is a pivotal area of study in astrophysics. It reshapes our understanding of fundamental laws of physics and the dynamics of cosmic events. The significance of observing these collisions extends beyond mere academic interest; it offers insights into the formation of galaxies and the evolution of cosmic structures. With advancements in technology, particularly in gravitational wave detection and electromagnetic observation, scientists can gather extensive data on these extraordinary phenomena.
Electromagnetic Signals
Light Emission
Light emission is one of the earliest signs associated with black hole collisions. When two black holes merge, they can generate intense light across various spectra. This light emission helps researchers detect and understand the collision events. The primary characteristic of light emission is its ability to travel vast distances, allowing us to observe ancient events. This makes it a popular choice for studies aiming to piece together the history of the universe.
A unique feature of light emission is the spectrum it covers. Traditional telescopes can capture optical light, but specialized instruments can measure other wavelengths like infrared and ultraviolet. One advantage of utilizing light emission in this context is that it often provides real-time data, unlike some other detection methods. However, atmospheric interference can diminish clarity, requiring advanced filtering techniques.
X-ray and Gamma-ray Bursts
X-ray and gamma-ray bursts are high-energy phenomena resulting from black hole collisions. These bursts carry immense energy and play a critical role in astrophysical observations. A notable characteristic of these bursts is their short duration; they can last from milliseconds to hours. Such potency makes them substantial for studies focusing on energetic events in the universe. X-ray and gamma-ray bursts are vital for understanding the aftermath of black hole mergers, shedding light on matter behavior in extreme conditions.
The unique feature of X-ray and gamma-ray bursts is their ability to signal the presence of black holes or neutron stars. They often serve as indicators of extreme gravitational forces at work. The advantage of high-energy detection is that it can penetrate dense cosmic materials, revealing hidden events. However, the challenge lies in the need for sensitive instrumentation, as conventional detectors might not capture these fleeting phenomena.
Gravitational Waves
Detection Methods
Detection methods for gravitational waves have revolutionized astrophysics. These waves are ripples in spacetime caused by massive acceleration, such as black hole collisions. The methods employed focus on measuring tiny changes in distance caused by passing waves. This trait makes gravitational waves a profound tool for observing events that might be invisible to electromagnetic methods. This detection method is beneficial for grasping the dynamics of colliding black holes, providing information about their masses and spins.
Unique features of the detection methods include LIGO and Virgo observatories, which utilize laser interferometry to achieve breakthrough sensitivity. One advantage of this technology is its ability to provide information from vast distances and historical depths without being hindered by cosmic dust or gas. Yet, it is essential to note the complexity and high cost associated with these observatories, which might limit accessibility for wider research.
Significant Observations
Significant observations in gravitational wave astronomy mark a milestone in understanding the universe. Events like the first detection of gravitational waves from the merger of two black holes in 2015 opened new avenues in astrophysical research. Highlighting this breakthrough illustrates how detection not only confirms theoretical predictions but also provides new data about the universe's structure.
A critical characteristic of these significant observations is their contribution to merging black hole properties, such as mass and distance. This data is essential for models predicting black hole behavior and frequency of collisions. The unique feature of significant observations lies in their potential to discover previously unseen phenomena, offering a glimpse into the universe's workings. However, limitations include the fact that only a small fraction of events may be detectable, leaving gaps in data that require further research and technology improvements.
Astrophysical Implications of Collisions
Black hole collisions hold profound implications in astrophysics. These events do not merely represent an interesting anomaly in the cosmos; they are pivotal in understanding the structure and evolution of galaxies. The study of these collisions influences our theories about cosmic formations and the behavior of matter on grand scales. As we examine the nuances of how black holes interact, we can uncover insights into fundamental questions about the universe's origins and its ultimate fate.
Influence on Galaxy Formation
The process of galaxy formation is deeply entwined with the existence of black holes. When two black holes collide, they can produce significant gravitational effects that influence the dynamics of surrounding matter. This interaction can impact star formation rates in a galaxy. For example, the energy released from such collisions can compress gas clouds, spurring new star formations, or they can disrupt existing stars.
Furthermore, when supermassive black holes at the center of galaxies merge, they can alter the gravitational landscape significantly. The repercussions may extend over vast distances, highlighting dependencies among galactic structures. These events can serve as catalysts for galaxy mergers, influencing the morphology and development of galaxies over time.
Matter Redistribution in the Universe
Black hole collisions are also instrumental in the redistribution of matter throughout the universe. During the merger process, immense amounts of energy are emitted in the form of gravitational waves, leading to the ejection of matter away from the collision site. This redistribution can alter existing mass concentrations, impacting the cosmic webโthe large-scale structure of the universe. Such events facilitate the movement of gas and dark matter, contributing to the formation of new structures.
In addition, the chaos and gravitational waves generated from these mergers may lead to the phenomenon known as relativistic jets. These jets can ejected matter at near-light speeds and can travel across intergalactic distances, potentially influencing environments far removed from their origins.
In summary, the ramifications of black hole collisions extend significantly beyond the immediate vicinity of the event. They influence the formation of galaxies, the dynamics of gas and dark matter, and the future of cosmic structures. This area of research is fertile ground for further exploration, as understanding these complex interactions can enhance our overall comprehension of the universe.
Technological Advances in Studying Black Holes
The advancements in technology to study black holes have been transformative in astrophysics. This section highlights the key elements, benefits, and considerations related to two significant areas: gravitational wave astronomy and imaging techniques.
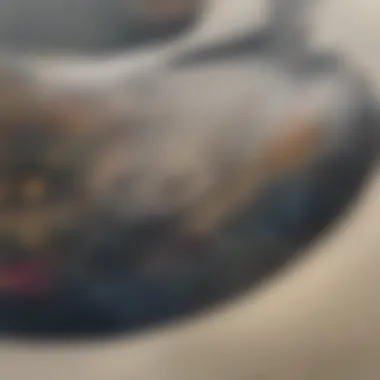
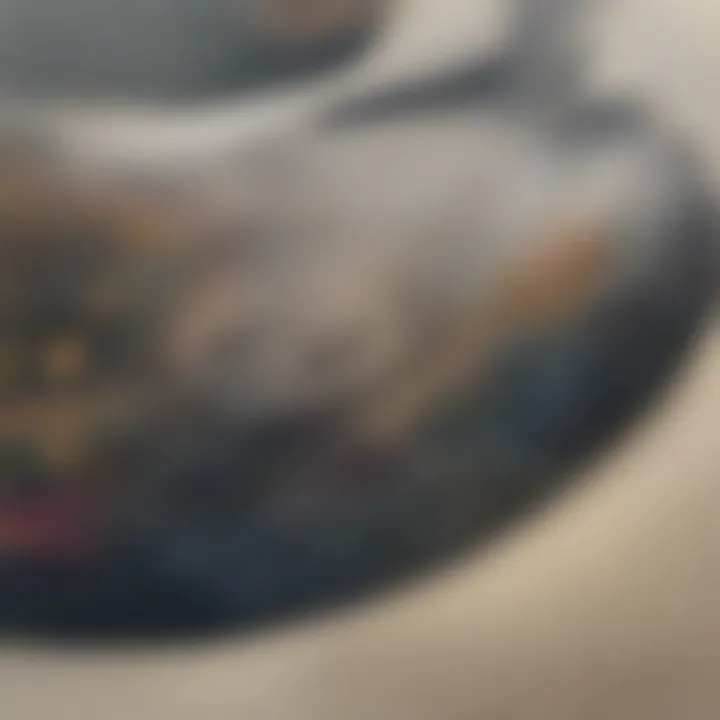
Gravitational Wave Astronomy
Gravitational wave astronomy has opened an entirely new frontier in the observation of cosmic events. Using instruments like the Laser Interferometer Gravitational-Wave Observatory (LIGO), scientists can detect tiny ripples in space-time caused by massive events, such as black hole collisions. This groundbreaking technique provides insight into the properties of black holes and their interactions.
The significance of gravitational wave astronomy lies in its ability to observe phenomena that were previously hidden from view. By detecting these waves, researchers can gather data on black hole masses and spins, leading to a deeper understanding of their formation and evolution. The ability to track these occurrences in real-time allows for a more dynamic approach to studying astrophysical events.
Additionally, gravitational waves contribute to the confirmation of general relativity in extreme conditions, providing empirical evidence supporting existing theories. As technology progresses, gravitational wave detectors will become more sensitive, enhancing our capability to identify more distant and smaller collisions.
Imaging Black Holes
Imaging black holes presents unique challenges and opportunities in astrophysics. The Event Horizon Telescope is a notable project aimed at capturing direct images of black holes, particularly the supermassive black hole at the center of the Milky Way, known as Sagittarius A*.
Event Horizon Telescope
The Event Horizon Telescope utilizes a global network of radio telescopes, effectively creating a planet-sized virtual telescope. This configuration gives it the sensitivity necessary to visualize the shadow of a black hole against the backdrop of surrounding light. One key characteristic that makes the Event Horizon Telescope advantageous is its capability to capture data in high resolution, allowing scientists to observe gravitational effects around black holes.
The benefit of this project lies in its pioneering approach to direct visualization, which complements the observations made through gravitational waves. However, the unique feature of using an array of telescopes introduces complexities in data synchronization and interpretation, leading to significant computational challenges.
Challenges of Imaging
The challenges of imaging black holes stem from their inherent nature; black holes are, by definition, regions where gravity is so strong that nothing can escape, including light. This presents fundamental difficulties in capturing clear images. One key aspect to consider is the need for extremely high resolution to discern details near the event horizon.
Moreover, atmospheric conditions and the vast distances involved complicate the capture process, presenting a limiting factor in the frequency and quality of images obtained. Despite these hurdles, the continuous improvements in algorithms and computational power are gradually overcoming these obstacles, enabling clearer images and better data handling.
"The challenge of imaging a black hole pushes the limits of our technological capabilities, revealing both our ambitions and the mysteries that still elude us."
Through the advances made in both gravitational wave astronomy and imaging black holes, we better understand these enigmatic objects. As we continue to refine our methodologies, the potential for new discoveries remains vast and promising.
Mathematical Models of Black Hole Collisions
Mathematical models of black hole collisions are vital in advancing our understanding of such complex astrophysical events. These models allow researchers to simulate and analyze the extreme environments that emerge during collisions. By employing sophisticated mathematics and computer algorithms, scientists can predict behaviors and outcomes that would otherwise remain inaccessible through direct observation. This section will delve into the significance of simulations and computations, as well as predictive models that are critical to analyzing black hole collisions.
Simulations and Computations
In astrophysics, simulations are integral for investigating the dynamics of black hole collisions. They provide a practical approach to understanding the interactions between massive objects without requiring physical experiments, which are impossible given their cosmic scale. Computational models allow scientists to create environments based on the principles of general relativity and other relevant physical laws.
These simulations are often sophisticated and utilize numerical methods to solve complex equations related to gravitational forces, matter dynamics, and energy distributions. Here are some key elements of these simulations:
- Numerical Relativity: This involves formulating Einsteinโs equations in a form suitable for computation.
- Initial Conditions: Accurate specifications of the black holesโ properties, such as mass and spin, are essential for meaningful results.
- Grid Schemes: Utilizing adaptive mesh refinement techniques helps to manage computations efficiently, focusing on areas of interest without wasting resources.
The benefits of simulations extend beyond mere visualization. They contribute to a richer coupling of theory with observational data. Researchers can refine their models based on what is observed in gravitational wave data, making simulations a two-way street between theory and observation.
Predictive Models
Predictive models serve an important role in theoretical astrophysics. They provide forecasts based on existing theories and observed phenomena. These models aim to predict outcomes of black hole collisions, which can then be tested against future observations or existing data.
Key aspects of predictive models include:
- Event Rate Estimations: Models can estimate the frequency of collisions within different regions of the universe. This helps determine how many events like these we can reasonably expect to detect, thereby guiding observational strategies.
- Waveforms Prediction: In gravitational wave astronomy, the production of waveforms corresponding to specific collision scenarios allows researchers to compare theoretical predictions with actual detections.
- Parameter Space Exploration: Predictive models allow for exploration of various parameters that influence collision dynamics, which is essential for a thorough understanding of the physical processes involved.
Ethical Considerations in Astrophysical Research
Astrophysical research, particularly in the context of phenomena such as black hole collisions, raises several ethical considerations that must be addressed. While the primary aim of this research is to deepen our understanding of the universe, the implications it holds for society and the scientific community are significant. Ethical considerations cover a spectrum of challenges ranging from the responsible use of data to the inclusivity of diverse perspectives in scientific discourse.
One central element is the impact of research on scientific communities. Scholars and researchers must consider how their findings contribute to existing knowledge and influence future investigations. It is essential to maintain integrity in the reporting of results. This upholds the credibility of science and fosters trust among peers and the public. Misrepresentation or selective reporting can lead to misconceptions not only about black holes but also about the scientific process itself.
Furthermore, researchers should recognize the societal context surrounding their work. The exploration of black holes, while seemingly remote from daily life, can lead to technological advancements with far-reaching effects. For instance, the techniques developed for gravitational wave detection could enhance other technological fields, potentially altering everyday experiences. Therefore, scientists have a responsibility to consider how their research may benefit or harm society at large.
Another aspect is the need for ethical engagement in interdisciplinary research. The study of black hole collisions often involves collaboration across different scientific domains, including physics, astronomy, and even philosophy. Ensuring that discussions are inclusive and that differing opinions are respected can lead to richer and more nuanced understandings of complex issues. This diversity can ultimately help mitigate biases that might arise when perspectives are limited.
"Ethics in research is not just about compliance; it is about fostering an environment of accountability and collaboration that enriches knowledge creation."
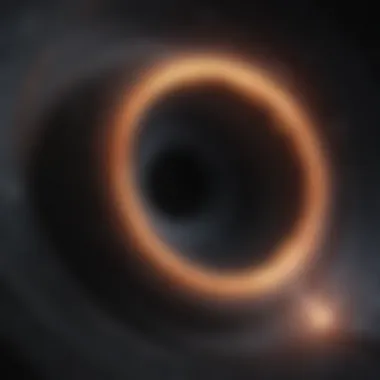
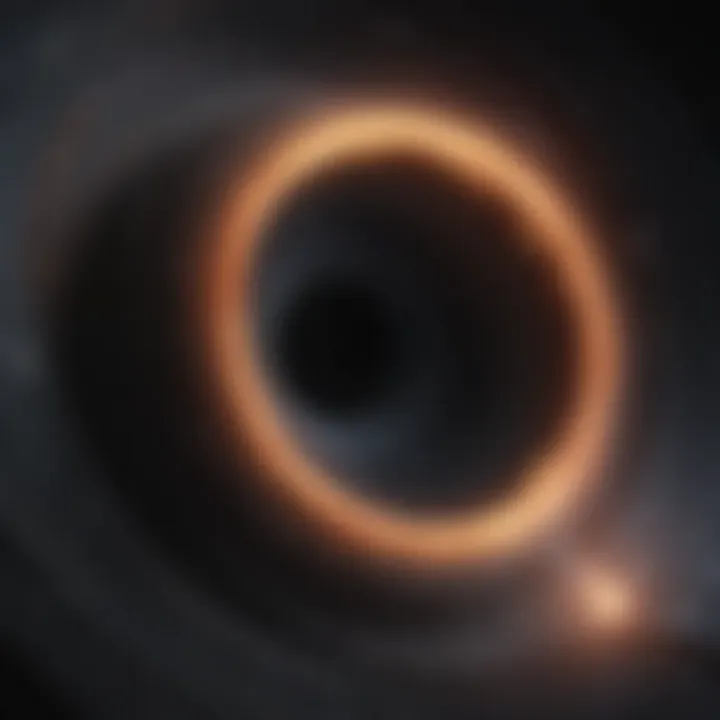
Impact on Scientific Communities
The impact of ethical considerations extends profoundly into scientific communities. In an environment thriving on collaboration, ethical frameworks guide the way researchers interact, share information, and respect each other's contributions. When scientists uphold ethical standards, they enhance the collective pursuit of knowledge.
Transparency is a notable benefit. Open communication about findings and methodologies allows for reproducibility, which is a cornerstone of scientific inquiry. This principle encourages others in the community to verify results, fostering confidence in the scientific process. On the other hand, lack of ethical standards can lead to conflicts and mistrust, making collaboration difficult.
Moreover, ethical practices promote inclusiveness in research methodologies. By valuing diverse voices, ethics encourages the integration of fresh insights that can lead to innovative solutions in astrophysical challenges. This breadth of input enhances the quality and relevance of research, making it more reflective of the complexities of the universe and society as a whole.
Philosophical Implications
Philosophically, ethical considerations in astrophysical research raise important questions about the nature of discovery and our responsibilities as custodians of knowledge. When we study enigmatic entities like black holes, we tread on vast and uncharted territories. The implications of our findings could reshape our fundamental understanding of space, time, and existence itself.
One pressing question is: what does it mean to claim knowledge of cosmic phenomena? The pursuit of knowledge in astrophysics often rests on theoretical models that may never be fully observed or verified. This uncertainty presents ethical dilemmas regarding how confidently scientists should present their findings to the public and the broader scientific community.
Additionally, the understanding of black hole collisions integrates concepts of causality, existence, and the role humans play in the cosmos. These philosophical implications compel researchers to contemplate their ethical obligations, not merely to their peers but to future generations.
In essence, the discourse surrounding ethical considerations is not just confined to the laboratory or observatory; it branches into the very way humanity perceives its place in the universe. As discoveries unfold, so too must the conversations about their implications and responsibilities.
Future Directions in Research
Research on black hole collisions continues to advance, highlighting the intricacies of the universe and our role in understanding it. Future directions in this field are critically important as they promise to unfold new avenues for inquiry and discovery.
One essential element is the development of advanced observational technologies that can enhance our capacity to detect and study gravitational waves. For instance, the next generation of observatories, such as the Einstein Telescope, will offer improved sensitivity and resolution. This will enable scientists to observe more distant events, ultimately broadening the scope of research on black hole collisions.
Benefits of exploring future research directions include:
- Enhanced detection capabilities: Improvements in technology will facilitate capturing signals from mergers that were previously too faint to observe.
- Greater understanding of astrophysical phenomena: A more extensive dataset allows for refined models of how black holes interact and evolve.
- Interdisciplinary collaboration: As scientists in different fields contribute their expertise, the collective understanding of black holes can significantly expand.
Key considerations include the ethical implications of such research and the significance of collaboration in interdisciplinary approaches.
Upcoming Missions and Projects
Research into black hole collisions is set to be revolutionized by upcoming missions and projects. Many space agencies and research institutions are assembling initiatives that aim to push the boundaries of current astrophysics. For example, projects like the Laser Interferometer Space Antenna (LISA) will be capable of detecting gravitational waves at lower frequencies than current terrestrial detectors. This advantage could allow for observations of black hole collisions in regions of the universe that have not been easily accessible.
Additionally, the integration of new satellite missions can lead to enhancing our understanding of high-energy astrophysics. Projects focused on using space observatories to monitor the electromagnetic spectrum, including X-ray and gamma-ray emissions, will complement gravitational wave observations. These dual approaches can provide a more holistic view of black hole events, making it possible to connect theoretical models with empirical data.
The Role of Interdisciplinary Approaches
Future directions in research are not solely reliant on advancements in technology but also on the integration of interdisciplinary methodologies. Astrophysics, cosmology, computer science, and engineering all converge to explore the profound questions surrounding black hole collisions.
A notable aspect of interdisciplinary research is the shared knowledge that emerges when experts from different fields collaborate. This synergy can lead to:
- Innovative methodologies: Breaking away from traditional research boundaries can yield new techniques for data analysis, simulation, and modeling.
- Enhanced theoretical frameworks: Diverse perspectives contribute to creating more robust and comprehensive theoretical models concerning black holes.
- Public engagement: Incorporating social sciences helps translate complex scientific findings into formats understandable for the broader public, fostering greater interest in space science.
As researchers continue to push the envelope of black hole studies, it becomes clear that the future of this discipline is rooted in cooperative effort and a willingness to explore novel ideas.
"Collaboration and interdisciplinary approaches will be the cornerstone of our next big leap in understanding black holes and their collisions."
In summary, the future directions in black hole collision research are multifaceted, involving innovative technologies, novel missions, and interdisciplinary strategies that hold promise for expanding our knowledge of the universe.
Closure
The conclusion of this article serves as a pivotal point in embracing the profound implications of black hole collisions within the realm of astrophysics. It synthesizes the intricate knowledge established throughout the text and highlights not only the significance of understanding these cosmic events but also the broader cosmic insights they reveal.
Summary of Key Findings
Through careful examination, it is clear that black hole collisions are not mere celestial events. They provide crucial clues about the nature of the universe, including insights into gravity, spacetime, and the extremes of physical laws. Key findings include:
- Gravitational Waves: The detection of gravitational waves has fundamentally changed our perception of astrophysical phenomena. Significant events, like the merger of two black holes, have been captured, providing evidence supporting general relativity.
- Cosmic Phenomena: Black hole collisions influence the structure of galaxies and the overall matter distribution in the universe. The energy released in these collisions can have lasting effects on their surroundings.
- Technological Innovations: Advances in technology, especially in gravitational wave astronomy and imaging techniques, continue to enhance our understanding of black holes. These tools enable more precise observations and more robust data collection.
Implications for Future Research
Looking forward, the implications of this research are extensive. Future studies could pave the way for newfound discoveries, including:
- Enhanced Detection Methods: With continuous advancements in technology, enhanced methodologies for detecting black hole collisions could emerge. This would allow for even more frequent and detailed observations.
- Interdisciplinary Approaches: Collaboration between different scientific fields may lead to a more comprehensive understanding of black holes and their interactions. The integration of insights from physics, computer science, and even philosophy may provide novel perspectives on these phenomena.
- Expanding Cosmological Theories: Ongoing research may refine or even redefine existing theories about the universeโs origin and development. Understanding black hole collisions could help solve fundamental questions surrounding dark matter and energy.
In summary, comprehending black hole collisions has far-reaching implications that contribute significantly to our understanding of the universe. Continued exploration in this field is essential for unraveling the mysteries that lie beyond our immediate observational capacity.