Understanding the Higgs Boson Particle and Its Impact
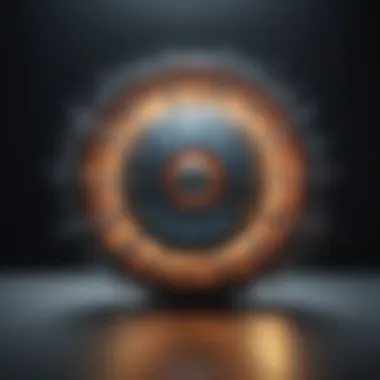
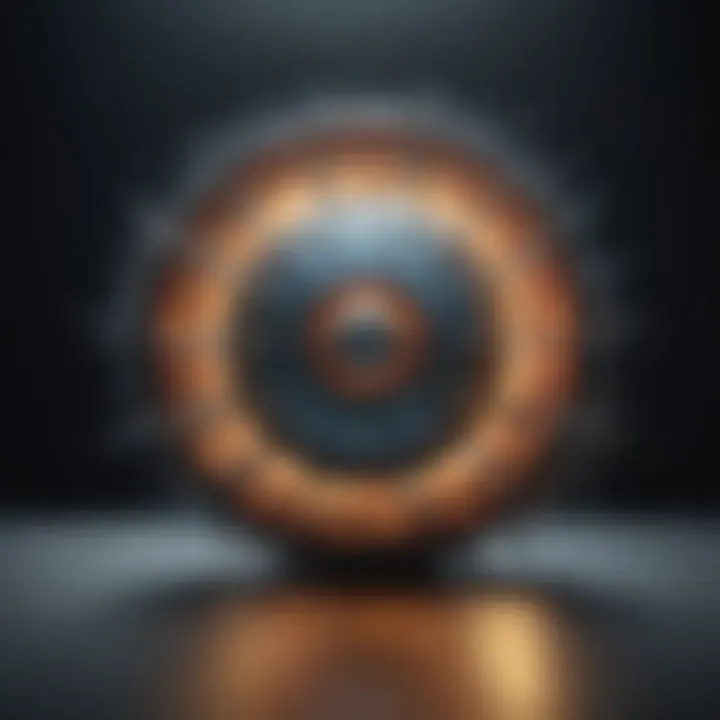
Intro
The Higgs boson is a cornerstone of contemporary physics, playing a key role in our understanding of mass and the fundamental building blocks of the universe. Its discovery was a major milestone in the field of particle physics, providing crucial insights into the Standard Model. This article will unfold the intricate nature of the Higgs boson, focusing on its properties, discovery, and significance in scientific theory.
In this journey, we will explore the methodologies employed to detect this elusive particle, particularly at the Large Hadron Collider. We will also discuss ongoing research that extends beyond the Standard Model, investigating implications for the cosmos. By addressing the complexities surrounding the Higgs boson, this piece aims to deepen the readerβs comprehension of its relevance in modern science.
Key Research Findings
Overview of Recent Discoveries
Since its discovery in 2012 by the ATLAS and CMS experiments at CERN, the Higgs boson has been a subject of intense research. Scientists confirm that the particle has a mass around 125 giga-electron volts. Recent experiments continue to probe its properties, leading to both confirmatory and novel insights. For example, research indicates that the coupling strength between the Higgs boson and other particles aligns closely with theoretical predictions, affirming the robustness of the Standard Model.
Another exciting area of research involves the investigation of the Higgs bosonβs self-interaction. This aspect is fundamental to understanding how the universe evolved shortly after the Big Bang.
Significance of Findings in the Field
The findings related to the Higgs boson are pivotal for several reasons. They validate the theoretical framework that underpins particle physics. Not only does this ease our understanding of mass generation, but it also poses questions that propel further inquiry into physics beyond the Standard Model.
"The discovery of the Higgs boson is not just an end, but a beginning of a new chapter in our understanding of the universe."
Simplifying Complex Concepts
Breakdown of Advanced Theories
One of the challenges in grasping the significance of the Higgs boson is its complex theoretical background. The Higgs field, for instance, is crucial for explaining why particles have mass. When particles move through this field, they acquire mass through interactions, akin to moving through a medium that impedes motion. This analogy simplifies a deep theoretical framework, albeit only to a certain extent.
Visual Aids and Infographics
Infographics can substantially aid in the understanding of the Higgs boson and its field. Visually representing interactions can elucidate how the Higgs boson influences other particles. Some useful resources include interactive visualizations available on en.wikipedia.org, which effectively showcase its discovery process and implications.
Prologue to the Higgs Boson
The Higgs boson plays a critical role in particle physics. Its discovery was not just an achievement in its own right but also a milestone for our understanding of the fundamental forces and particles that constitute the universe. This article will explore the journey of the Higgs boson, its implications, and its ongoing research, paving the way to new insights in physics.
The Genesis of the Higgs Concept
The concept of the Higgs boson originated in the 1960s, developed by several physicists, including Peter Higgs. The notion emerged from the need to explain how particles acquire mass. The work of Higgs and others led to a theoretical framework that integrated the idea of a field permeating all of space. This field can also be described as the Higgs field.
Crucial to modern physics, the Higgs concept resolved various inconsistencies in the then-current theoretical scaffolding. It provided a mechanism known as spontaneous symmetry breaking, allowing different particles to gain mass through interactions with the Higgs field. Over the years, this idea evolved, leading to the establishment of the Standard Model of particle physics.
Defining the Higgs Boson
The Higgs boson is a fundamental particle associated with the Higgs field. Discovering its properties enhances our understanding of particle physics. The Higgs boson itself is unique due to its linkage to mass. Understanding mass is central to both theoretical and experimental physics, as it influences everything from the behavior of elementary particles to the formation of galaxies.
The particle and the field
The Higgs boson serves as a crucial component of the Higgs field. When particles move through this field, they acquire mass. The interaction is not direct, but rather in how particles interact with the field itself determines their mass. This concept is key for a coherent explanation of the physical universe as we know it.
One significant characteristic of this relationship is that it goes beyond mere theory. The detection of the Higgs boson at the Large Hadron Collider (LHC) provided empirical evidence for the existence of the Higgs field. Its detection helped cement the link between the particle and its corresponding field. It's a favorable choice to discuss in this article because it brings theoretical concepts into the realm of empirical validation, which is a fundamental aspect of scientific inquiry.
Mass and energy relationship
The relationship between mass and energy is encapsulated in Einstein's famous equation, E=mcΒ². The Higgs boson is pivotal in translating this concept to the realm of particle physics. Particles that acquire mass through the Higgs mechanism possess energy as a result of that mass. It constructs a bridge between mass, energy, and the broader laws governing particles.
One key feature of this relationship is its applicability to various areas of physics. Understanding how mass arises from energy opens avenues for deeper comprehension of the cosmological models that attempt to explain the universe's evolution. It is beneficial to explore this aspect, as it grounds abstract physics into concepts that have universal implications. In this article, we will highlight how mass influences not only particles but also the structure of the universe as we know it.
The Higgs boson and its field are not merely theoretical constructs. Their implications reverberate through the very fabric of our universe, affecting how we understand both the micro and macro scales of physics.
Theoretical Framework
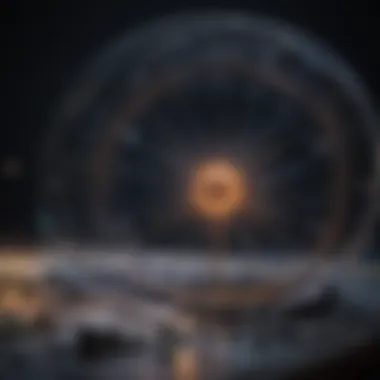
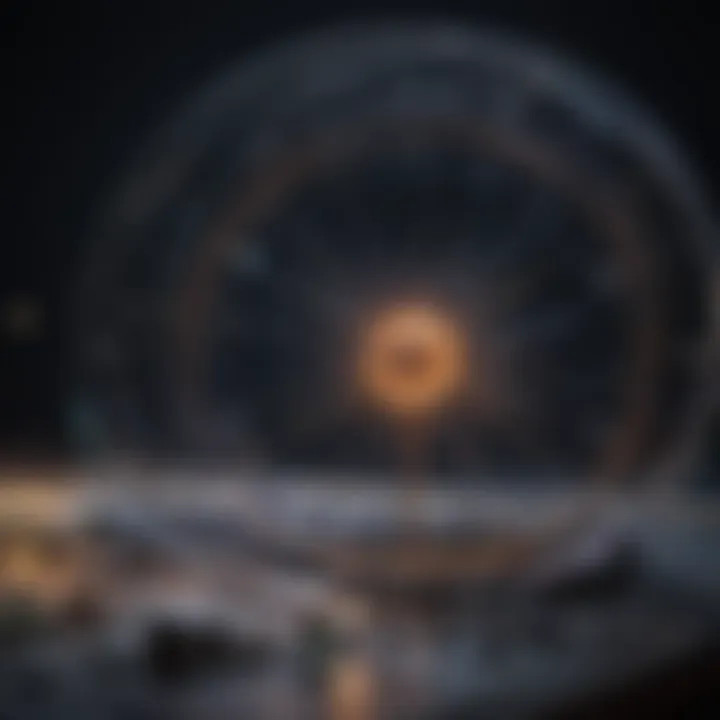
The theoretical framework serves as a cornerstone for understanding the Higgs boson and its role within the broader context of particle physics. This framework is not merely descriptive; it outlines the foundational principles that govern particle interactions. By exploring the theoretical underpinnings, readers can appreciate the significance of the Higgs boson within the Standard Model, the prevailing theory of particle physics. This provides insights into how fundamental particles gain mass and interact through distinct forces. The theoretical framework also highlights the implications of the Higgs mechanism for physics, setting the stage for deeper inquiries into both known and unknown aspects of the universe.
Standard Model of Particle Physics
Fundamental particles
Fundamental particles are the building blocks of matter in the universe. They are categorized mainly into fermions and bosons. Fermions include quarks and leptons, which make up visible matter, while bosons are force carriers such as photons and gluons. Understanding these fundamental particles is crucial because they inform scientists about the underlying symmetries of nature.
Key traits of fundamental particles include their inability to be broken down into smaller components. This characteristic makes them a focal point in particle physics, providing a clear delineation of matter. Exploring these particles not only clarifies the structure of atoms but also shapes our understanding of the forces that interact among them.
A unique aspect is how the identification of these particles allows researchers to predict new particles, guided by theories such as supersymmetry and string theory. This predictive power is advantageous for ongoing research, even if it has its limitations.
Interactions and forces
Interactions and forces dictate how these fundamental particles engage with each other. The weak force, strong force, electromagnetism, and gravity are the four fundamental forces that govern these interactions. The Standard Model successfully describes three of these forces and leaves gravity for future exploration.
The key characteristic of these interactions is the exchange of force-carrying particles, known as gauge bosons. For instance, photons mediate electromagnetic interactions, while W and Z bosons facilitate weak interactions. This conceptual framework is beneficial because it gives a coherent understanding of diverse physical phenomena van everyday life to high-energy environments.
However, a unique feature to note is that these interactions can lead to particle decay and transformations, opening pathways for complex processes like fusion in stars. While the interconnected web of interactions is insightful, some limitations persist, particularly in explaining gravity fully.
Importance of the Higgs Mechanism
How particles acquire mass
The Higgs mechanism is pivotal for understanding how fundamental particles acquire mass. It proposes that particles interact with the Higgs field, which permeates the universe. When particles interact with this field, they experience resistance, perceived as mass. This relationship between mass and the Higgs field is crucial for explaining the disparity between the mass of the W and Z bosons and that of other particles.
The key characteristic of this mechanism is that it unites various particles under a single framework, which elegantly solves the question of how particles gain mass. Its simplicity and explanatory power make it a vital focus for studies in particle physics.
One remarkable aspect is the manner in which this mechanism reinforces the validity of the Standard Model. By accounting for mass, the Higgs mechanism shines a light on the compatibility of theoretical predictions with experimental observations.
The place of the Higgs field
The Higgs field is an omnipresent field that is integral to the structure of the universe. Its importance lies in its role as the medium through which particles gain mass. Without the Higgs field, fundamental particles would remain massless and unable to form atoms, which are essential for the existence of matter.
The key characteristic of the Higgs field is its uniform presence throughout space. Its unique feature is the non-zero value it retains even in a vacuum, which is counterintuitive but crucial for particle physics. This feature illustrates why particles can possess mass in certain conditions while remaining massless in others, such as at relativistic speeds.
While the Higgs field has enriched our theoretical understanding, challenges remain in fully reconciling its existence with phenomena such as dark matter.
The role of the Higgs boson is not simply about particle mass; it questions fundamental aspects of the universe itself.
Discovery of the Higgs Boson
The discovery of the Higgs boson serves as a cornerstone in modern particle physics. It validated essential aspects of the Standard Model and deepened our understanding of mass. This section explores different phases of the search for the Higgs particle, detailing the efforts and methodologies that culminated in its discovery.
Large Hadron Collider: The Search Begins
Construction and purpose
The Large Hadron Collider (LHC) was built at CERN to explore fundamental questions in particle physics. It is the worldβs largest and most powerful particle accelerator. Its main purpose was to collide protons at near-light speeds. This facility aimed to recreate the conditions similar to those right after the Big Bang, allowing scientists to probe fundamental particles, including the elusive Higgs boson.
A key characteristic of the LHC is its circular design, stretching about 27 kilometers underground. This layout enables multiple particle collisions, increasing the likelihood of discovering rare events, such as Higgs production. Its high-energy collisions have made it a notable choice for experiments in particle physics. However, the scale and complexity of the LHC also present challenges, such as significant technical and operational demands.
Experimental methodology
The experimental methodology at the LHC is highly sophisticated. It revolves around two main experiments, ATLAS and CMS, designed to detect various particles created during collisions. These experiments employ advanced tracking and detection systems to observe decay paths of particles, including the Higgs boson itself.
An important aspect of the methodology is the use of powerful data analysis techniques. The digital data produced during collisions is monumental, necessitating cutting-edge computing systems for analysis. This high-tech approach is not only beneficial but essential for extracting meaningful results from the experiments. Yet, the complexity of the system may lead to potential data misinterpretations.
Confirmation of the Higgs Boson
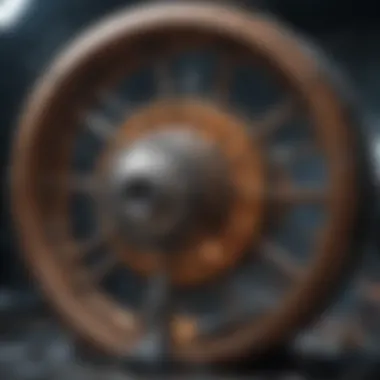
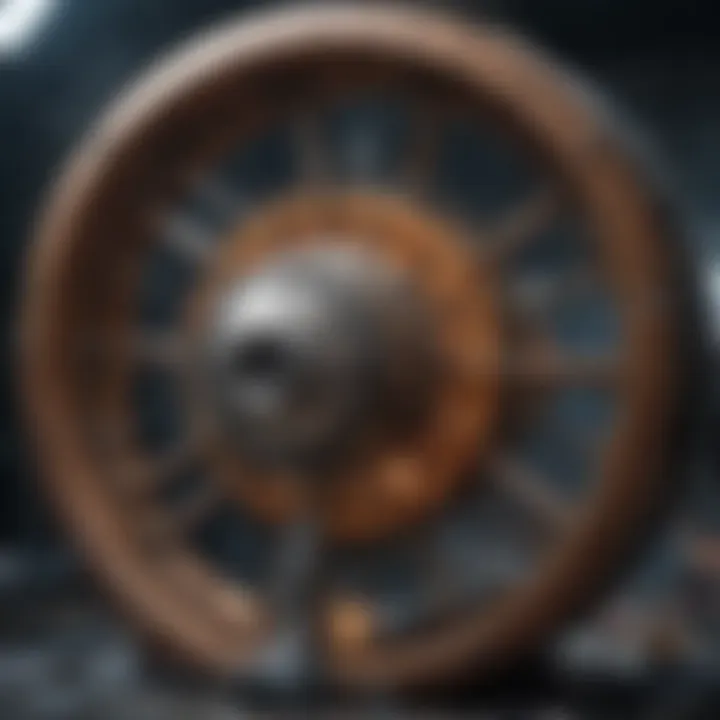
Data analysis
The data analysis process is critical for confirming any particle's existence. In the context of the Higgs boson, physicists utilized a plethora of statistical methods to filter through massive datasets. This process involved identifying signals indicative of the Higgs particle, focusing on specific decay channels that are theoretically predicted.
A key characteristic of the data analysis was the rigorous statistical criteria established to rule out background noise. This attention to detail ensured that any detected signals were credible and significant. This analytical process is vital for drawing robust conclusions in particle physics. Nevertheless, the inherent complexity of the data analysis necessitated extensive collaboration among scientists.
Announcement in
The announcement of the Higgs boson discovery in July 2012 marked a monumental moment in physics. Following thorough analysis, CERN presented evidence of a new particle consistent with the properties of the Higgs boson. This was a significant breakthrough for the scientific community, as it confirmed long-standing theories regarding mass acquisition in fundamental particles.
A key characteristic of the announcement was the collaboration effort across diverse research teams, which showcased the global nature of modern scientific research. The discovery was not only a triumph for the LHC but also emphasized the importance of international collaboration. However, while celebrating the discovery, researchers recognized that further investigations were needed to understand the particle's properties comprehensively.
Properties of the Higgs Boson
Understanding the properties of the Higgs boson is crucial for both theoretical and experimental physics. The Higgs boson, which is a fundamental particle in the Standard Model of particle physics, plays a significant role in explaining how other particles acquire mass. Its propertiesβranging from mass to interaction with other particlesβreveal a lot about the underlying mechanics of the universe. Each characteristic of the Higgs boson contributes to our comprehension of cosmic structure, particle stability, and the dynamics within particle collisions.
Mass and Stability
Measured mass
The measured mass of the Higgs boson is about 125 giga-electronvolts (GeV). This value is significant because it fits within the predictions made by the Standard Model. This particle was discovered in 2012, and its mass has implications for various theoretical constructs. A Higgs boson with a higher mass could have altered the dynamics of the universe shortly after the Big Bang, affecting formation patterns of matter and energy. Moreover, this mass allows us to explore electroweak symmetry breaking alongside other essential processes in particle physics. The unique feature of the measured mass lies in its precision; any significant deviation could challenge existing models and lead to new physics.
Lifetime and decay
The lifetime of the Higgs boson is incredibly short, approximately 10^-22 seconds. This rapid decay is crucial for understanding how it transmits mass to other elementary particles. When it decays, the Higgs boson produces various other particles, which can be detected and measured in experiments, mainly at the Large Hadron Collider. The decay channels of the Higgs boson are numerous, and they vary based on its interaction with other particles. A unique aspect of this process is that it enables physicists to infer the existence of the Higgs boson indirectly through its decay products. However, the short lifespan presents challenges for experimental verification, making it a double-edged sword in studies of particle interactions.
Interaction with Other Particles
Couplings to fermions and bosons
Couplings of the Higgs boson to fermions and bosons establish how it interacts with matter fields. This is pivotal, as these couplings dictate the mass of elementary particles through the Higgs mechanism. The distinctive characteristic of Higgs couplings is the proportionality to particle masses; heavier particles, like top quarks, couple more strongly. Therefore, observing these couplings provides insights into the mass hierarchy of particles. Understanding these interactions is beneficial for further examining the relationships established in the Standard Model and potential extensions.
The role in particle collisions
In particle collisions, the Higgs boson plays a central part by mediating interactions between various types of particles. When high-energy particles collide, conditions can sometimes lead to the creation of Higgs bosons. These collisions act as a testing ground to study expected behaviors of the Higgs field. A notable characteristic is that the creation of Higgs bosons is maximized at certain energy levels. This specific role helps validate the predictions made by the Standard Model and allows scientists to probe deeper into the fabric of matter. While studying these collisions provides extensive data, the complexity of the interactions can also hinder straightforward interpretations, suggesting a need for advanced methodologies in analysis.
These properties open a window into the fundamental workings of our universe. Understanding the Higgs boson could pave the way for breakthroughs in existing theories.
Implications of the Higgs Boson
The discovery of the Higgs boson opened pathways to many implications in the field of physics. Understanding these implications is vital for comprehending the fundamental principles of our universe. Higgs boson reassures the standard model, yet challenges popular theories, particularly in cosmology and our understanding of dark matter. This section will elaborate on significant aspects, highlighting how this particle advances knowledge in these areas while presenting new challenges to accepted theories.
Significance in Cosmology
Contribution to universe formation
The Higgs boson plays a critical role in the formation of the universe. In the early moments after the Big Bang, the Higgs field influenced particle interactions, which ultimately shaped the structure of matter in our universe. One key characteristic of this contribution is its ability to give mass to fundamental particles through the Higgs mechanism. As a result, it became possible for atoms to form, leading to stars and galaxies. The understanding of this process is not merely historical; it is fundamental in appreciating how the universe we observe came to be.
The unique feature of this contribution lies in the intrinsic link between mass acquisition and the Higgs field. Without it, particles would not have mass, and complex structures necessary for life would not exist. This relevance underscores the importance of research surrounding the Higgs boson in cosmology, as it has implications for understanding the early Universe.
Relation to dark matter
The relationship between the Higgs boson and dark matter is also an area of substantial interest. While the Higgs particle does not directly account for dark matter, its discovery may illuminate new theories regarding dark matter particles. The search for answers involves exploring the possibility that dark matter particles could interact with the Higgs field. A key characteristic of these interactions is that the mass of dark matter could potentially be linked to mechanisms involving the Higgs boson.
Understanding this relationship is important for the larger goal of elucidating one of the universe's greatest mysteries: the nature of dark matter itself. The unique feature of these explorations lies in their potential to create a cohesive model linking visible and invisible matter. This model could bridge gaps current theories face, although many uncertainties remain in defining these interactions. This interplay keeps the study of the Higgs boson at the forefront of physics.
Challenges to the Standard Model
The significance of the Higgs boson extends beyond its implications for cosmology. It brings forth challenges to the standard model of particle physics, leading to rigorous discussions within the scientific community. Exploring these challenges aids in understanding the limitations of existing theories and opens avenues for new ideas.
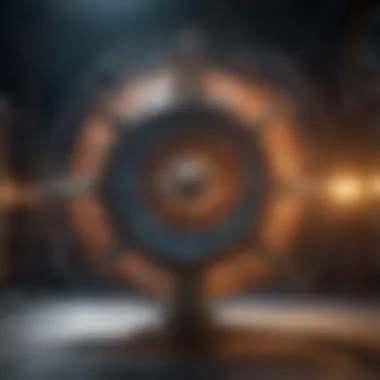
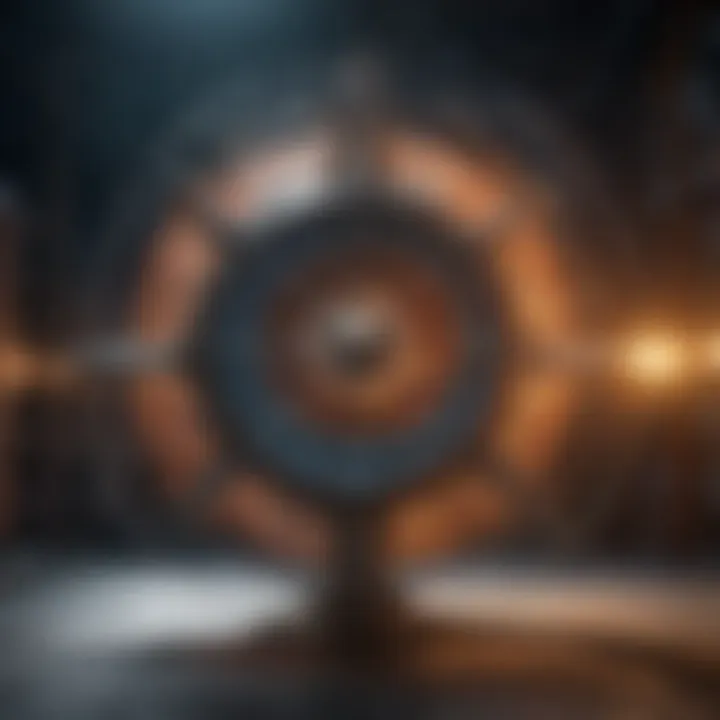
Open questions in physics
Several open questions in physics arise from the role of the Higgs boson in the standard model. One pressing question concerns why the Higgs boson is lighter than what some theoretical frameworks predict. This anomaly prompts investigation into what stabilizes its mass despite quantum fluctuations that could drive it higher.
Such questions highlight a key characteristic of this research area: an intersection of existing theories and new observations. The continual pursuit of answers keeps the discussion dynamic and relevant. In examining the open questions, researchers can inspire deeper inquiry into fundamental physics principles.
Beyond the standard model theories
Lastly, discussions on the Higgs boson bring focus to theories beyond the standard model. Some physicists propose additional particles or fields that could further explain phenomena not fully captured by established theories. Beyond the standard model theories offer compelling alternatives that can challenge and expand our understanding.
These theories often introduce new concepts like supersymmetry or extra dimensions. They provide a framework that might account for phenomena such as dark matter and the hierarchies in particle mass. Exploring these alternatives involves a unique feature of this field: the willingness to adapt and reevaluate based on new evidence.
Such explorations are significant as they push boundaries and may lead to a more complete understanding of macro and microcosmic interactions. This ongoing dialogue in the field molds the understanding of the universe, continuing to align with the quest for fundamental truths in physics.
Future Research Directions
Future research directions regarding the Higgs boson are crucial for advancing our understanding of particle physics. The ongoing efforts focus on not just what the Higgs boson is, but also what it can teach us about the universe. New technologies, increased collaboration, and innovative theories offer a pathway to uncover deeper insights about fundamental forces and particles.
Ongoing Experiments at CERN
Proposed upgrades to the LHC
the Large Hadron Collider (LHC) is undergoing some proposed upgrades that are intended to enhance its capabilities. These upgrades aim to increase the luminosity of the collider, which translates to a higher collision rate. More data from collisions means improved statistical analysis, giving researchers better chances of discovering rare phenomena involving the Higgs boson. The key characteristic of these upgrades is the improved detection accuracy. This helps in pinpointing interactions of the Higgs with other particles more effectively.
The upgrades are a beneficial choice for researchers because they will bolster the LHC's ability to explore beyond standard model physics. A unique feature of these upgrades includes advanced detector technologies that allow for better resolution and sensitivity to various particle interactions. However, these enhancements also come with significant financial implications and technical challenges.
Exploration of new physics
The exploration of new physics is another important aspect of ongoing research. It aims to go beyond what is currently understood through the standard model. By investigating phenomena that were not predicted, such as supersymmetry or extra dimensions, physicists hope to find more fundamental explanations of how particles interact, including the Higgs boson itself.
One key characteristic of exploring new physics is the potential to uncover unexpected results. This could lead to groundbreaking theories about the universe and its fundamental forces. This aspect is beneficial as it encourages innovative thinking and broadens the scope of particle physics. However, venturing into new territory can often lead to uncertainties, as theoretical foundations may not yet be fully established or experimentally confirmed.
Global Research Efforts
Collaborative projects
Global research efforts on the Higgs boson often stem from collaborative projects that unite scientists across different countries and institutions. This unity fosters the sharing of knowledge and resources, thereby accelerating the pace of research. The key characteristic of these collaborative endeavors is the pooling of expertise. By drawing on diverse areas of specialization, researchers can approach complex questions from multiple angles.
Collaborative projects are a beneficial choice for the study of the Higgs boson as they enhance the scientific community's ability to tackle intricate problems. A unique feature of these projects is their international scope, which can lead to more substantial funding opportunities and shared technologies. Nonetheless, these collaborations also require careful coordination and management, which can sometimes lead to disagreements and delays.
Advancements in particle physics
Advancements in particle physics are continually shaping the understanding of the Higgs boson and its implications. These advancements often come in the form of new theoretical models or refinements to existing ones. The key characteristic of these advancements is their impact on experimental design. A deeper understanding of the Higgs boson influences how experiments are structured.
These developments are incredibly beneficial, as they can lead to more precise measurements and predictions about particle behaviors. An important aspect of these advancements is the integration of computational methods that enhance data analysis. However, with rapid advancements comes the challenge of ensuring that new theories are aligned with empirical data, which is an ongoing requirement in the scientific process.
"The continuous evolution of our understanding of the Higgs boson inspires new methodologies that are critical for uncovering the secrets of the universe."
Culmination
The conclusion of this article encapsulates the significance of the Higgs boson within the field of particle physics. It serves as a crucial endpoint that synthesizes the vast information presented in the earlier sections, enhancing the reader's understanding of both the particle itself and its broader implications.
The Higgs Boson in Scientific Literature
The Higgs boson has been a subject of rigorous discussion in scientific literature since its theoretical prediction. Researchers have published numerous papers detailing its properties, discovery methods, and implications. Journals like Nature and Physical Review Letters have featured studies that explore the subtleties of the Higgs mechanism and its confirmation at the Large Hadron Collider. These writings often address challenges faced in particle physics, including the necessity of the Higgs field for mass generation and the ongoing puzzles related to dark matter and energy in cosmology.
Research has highlighted the key questions still remaining, such as why the mass of the Higgs boson itself is so low compared to the energy scales of other forces in the universe.
The Broader Impact of Higgs Research
The research surrounding the Higgs boson extends beyond mere particle physics. Its implications touch upon various domains, from cosmology to our understanding of the fundamental forces of nature. The Higgs boson's discoveries are continuously influencing theoretical models, pushing researchers to consider new frameworks that might unite different aspects of physics. Moreover, elements of Higgs research have cultural impact, sparking interest in STEM fields. By capturing the public's imagination through discussions of the universe's nature, the studies on the Higgs boson establish a link between advanced scientific inquiry and broader societal understanding. As physicists persist in their search for phenomena beyond the Standard Model, the legacy of Higgs research will undeniably shape future explorations in the realm of theoretical physics.
The ongoing pursuit of knowledge associated with the Higgs boson embodies the essence of scientific inquiry, as we continually seek deeper understanding of the universe.
The Higgs boson remains an integral component of modern physics, driving ongoing research efforts and theoretical advancements. From its inception in theoretical discourse to its confirmed existence, the particle offers a robust foundation for a multitude of scientific pursuits.