Exploring Quantum Computing: Principles and Impact
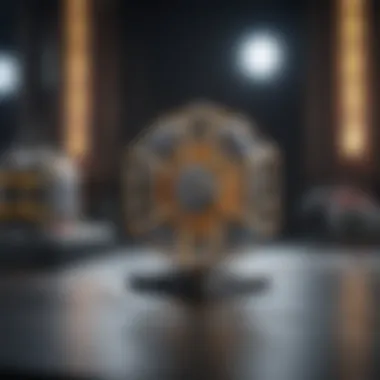
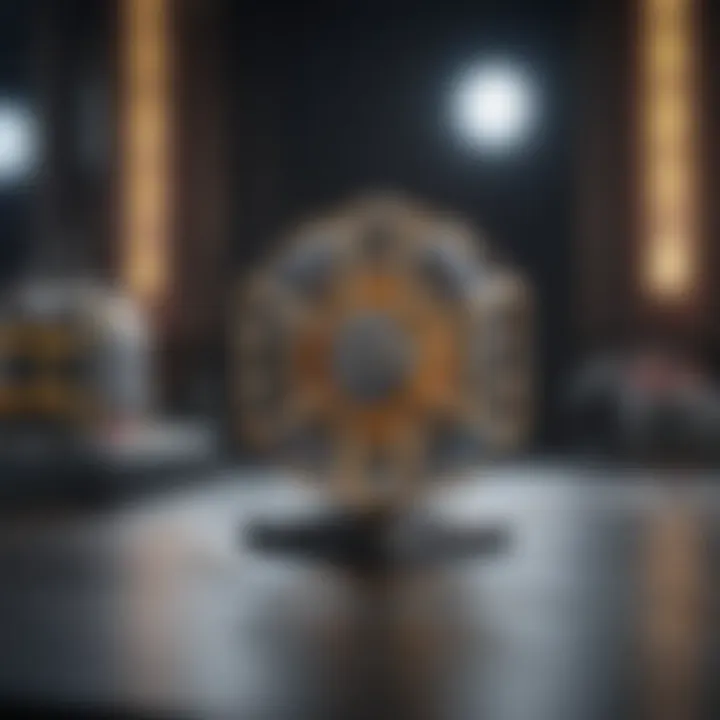
Intro
Quantum computing, a term that pops up more frequently in discussions about the future of technology, isn't just a trendy catchphrase. Instead, it's a complex field that seeks to revolutionize how we process information. At its core, quantum computing challenges traditional notions of computation by leveraging principles from the quantum realm. With concepts like qubits, superposition, and entanglement, the differences between classical and quantum systems become a fascinating area of exploration.
The excitement surrounding quantum computing lies in its potential. Industries from finance to healthcare are watching closely, eager to tap into its capabilities. However, as we dive into the mechanics and implications of quantum systems, it becomes crucial to understand the foundational concepts and the progress made in this burgeoning field.
In this exploration, we will navigate through the essential principles of quantum computing, highlight recent research findings, and break down some complex theories. The aim is to paint a clearer picture for students, researchers, educators, and professionals who are keen to grasp what this technological frontier may mean for our future.
Preamble to Quantum Computing
Quantum computing stands at the crossroads of science and technology, embodying a paradigm shift that transcends the foundational principles of classical computing. As we navigate through this intricate landscape, understanding quantum computing is not merely an academic pursuit; it holds significant implications for various sectors, from cryptography to artificial intelligence. This article serves as a detailed guide to the underpinnings and repercussions of quantum computing, shedding light on its potential to revolutionize our current computational models.
Defining Quantum Computing
At its core, quantum computing leverages the principles of quantum mechanics to process information. Traditional computers encode data as bits, which are either 0 or 1. In contrast, quantum computers utilize quantum bits, or qubits. These qubits can exist in a state of superposition, allowing them to represent both 0 and 1 simultaneously. This unique characteristic enables quantum computers to perform complex calculations at an unprecedented speed.
Beyond qubits, quantum computing introduces concepts like entanglement, where qubits become interconnected in ways that traditional bits cannot. This means the state of one qubit can instantly influence another, no matter the distance separating them. This interconnected behavior presents new avenues for computing power that were previously unfathomable.
In practical terms, this means that quantum computers have the potential to solve problems that are currently intractable for classical machines, such as factoring large numbers or simulating quantum systems. The implications are vast, impacting fields ranging from cryptography to materials science.
Historical Context
The road to quantum computing is paved with substantial theoretical groundwork that dates back to the early 20th century. It begins with the advent of quantum mechanics, a field that challenged classical physics and revealed the peculiar nature of atomic and subatomic particles. Notably, the work of physicists such as Albert Einstein, Niels Bohr, and Max Planck laid the bedrock for what would eventually evolve into quantum theory.
As we progressed into the 1980s, figures like Richard Feynman proposed that classical computers might struggle to simulate quantum systems, urging researchers to explore alternative computing models. In 1994, Peter Shor introduced his groundbreaking algorithm, which demonstrated how a quantum computer could factor large integers exponentially faster than the best-known classical algorithms. This watershed moment intensified interest in quantum computing, ultimately leading to the first experimental demonstrations by research institutions and tech companies, sparking a global race to harness its potential.
Today, major players, including IBM, Google, and various startups, invest heavily in research and development, cooking up quantum processors while tackling the challenges of stability and error rates. As quantum computing marches forward, understanding its mechanisms and implications is crucial for anyone vested in the future of technology.
Basic Principles of Quantum Mechanics
When delving into quantum computing, there's no getting around the importance of quantum mechanics. This foundational science serves as the bedrock upon which quantum computing stands. Understanding its principles is not just a bonus; it's essential for grasping how quantum bits, or qubits, operate and why they differ fundamentally from the classical bits we're accustomed to.
What makes quantum mechanics vital is its ability to challenge traditional ways of thinking about information and computation. Unlike classical mechanics, which follows strict, predictable laws, quantum mechanics introduces a level of unpredictability and complexity. This complexity is not merely a hurdle to jump overโit is the very essence that unlocks the astonishing potential of quantum computing.
Quantum States and Qubits
At the heart of quantum computing lies the concept of quantum states and, specifically, qubits. A qubit is the basic unit of quantum information, much like a bit in classical computing; however, its capabilities extend far beyond.
A qubit can exist in a state of 0, 1, or both simultaneously, thanks to a principle called superposition. This is what distinguishes it from a classical bit that can only represent one state at a time. To understand this better, consider a spinning coin: while it's in the air, it's not just heads or tailsโit's in a state that could be defined as both until it lands. This unique property enables quantum computers to process vast amounts of information more efficiently than traditional computers.
Here are some points that illustrate the significance of qubits:
- Parallelism: Because qubits can be in multiple states, they allow quantum computers to perform many calculations at once.
- Information Density: A single qubit can hold more information than a classical bit, enhancing data processing capabilities.
Superposition Explained
Superposition can often be a head-scratcher, but it plays a key role in quantum computing. In essence, a qโubit isnโt confined to the binary states of 0 or 1; instead, it can inhabit a state that is a combination of both. This is not a mere theoretical idea; it has real implications for how quantum computing operates.
Visualize this process through quantum algorithms. In a classical scenario, if you wanted to search for a specific item in a database, you would check each entry one after the other. In contrast, a quantum computer, thanks to superposition, could evaluate multiple entries simultaneously. Thus, when deployed correctly, quantum algorithms have the potential to transform computational tasks that would otherwise take ages into processes that complete in a fraction of the time.
"The magic of superposition is that, while classical bits can only work on one choice at a time, qubits can tackle several possibilities at once."
Quantum Entanglement
If superposition captures the imagination, quantum entanglement will leave you fascinated. Entangled qubits have a connection that transcends distance; when one qubit is changed, its entangled partner responds instantaneously, no matter how far apart they are. This peculiar phenomenon defies classical intuitions about information transfer, as it suggests a deep interconnectedness, kind of like a cosmic web.
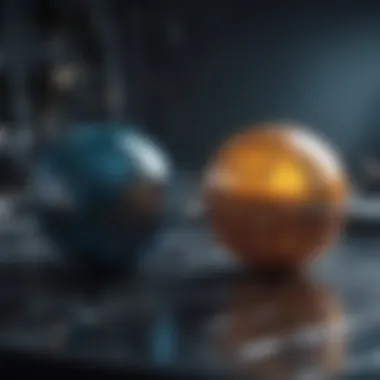
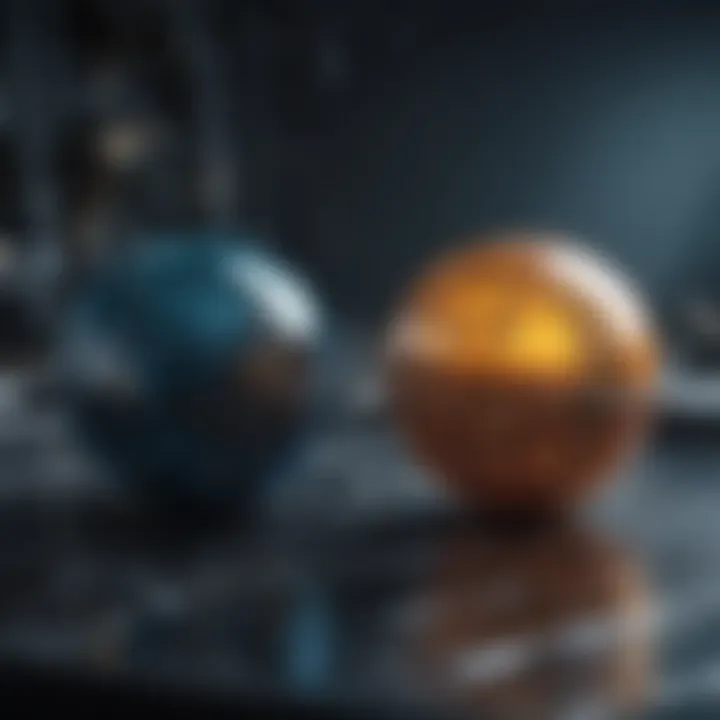
This characteristic has profound implications for quantum computing. It can enhance the speed of calculations and ensure that errors are minimized, allowing for more robust operations in quantum algorithms. Additionally, entanglement plays a critical role in quantum communication protocols, paving the way for unprecedented levels of data security.
To sum up, the principles of quantum mechanics aren't just academic exercises; they directly impact the capacity of quantum computers to outperform traditional systems. By grasping the nuances of quantum states, qubits, superposition, and entanglement, the pathway to understanding the broader implications of quantum computing begins to unfold in a compelling way.
Quantum Computing Architecture
In the realm of quantum computing, architecture plays a crucial role in determining the functionality and efficiency of quantum computations. Think of it like a blueprint that lays out how qubits work together to form quantum computers. As we step deeper into the topic, it becomes clear how various architectures can either make or break the effectiveness of quantum systems. The rich tapestry of mechanisms within quantum computing allows for diverse implementation strategies, each with its unique strengths and weaknesses, making it an engaging subject for researchers and technologists alike.
Qubit Implementation
When discussing quantum computing architecture, qubit implementation is at the forefront of the conversation. Qubits are the building blocks of quantum information, and their method of implementation significantly influences the performance of quantum computers. There are several approaches to implementing qubits, each showcasing its benefits and considerations. Letโs take a closer look at three primary methods:
Superconducting Qubits
Superconducting qubits utilize superconducting materials to achieve quantum states, capitalizing on the phenomenon of superconductivity. One key characteristic of superconducting qubits is their relatively high scalability; they can be integrated into larger arrays, allowing for complex computations.
Their unique feature lies in their operation at extremely low temperatures, which keeps them stable and minimizes noise, a significant factor for maintaining coherence in quantum states. This makes superconducting qubits a popular choice among many research groups, including IBM and Google. However, the cooling requirement can be a drawback, making it costly and complex to set up.
Advantages:
- High scalability
- Established technology and support
- Strong compatibility with existing fabrication techniques
Disadvantages:
- Requires elaborate cooling systems
- Susceptible to noise at higher temperatures
Ion-Trap Qubits
Ion-trap qubits, as the name suggests, utilize ions trapped in electromagnetic fields. This method allows individual quantum states to be manipulated using lasers. One important characteristic of ion-trap qubits is their exceptional degree of control. The ability to precisely manipulate qubits leads to lower error rates, which is pivotal in quantum computations.
The distinctive feature of ion-trap qubits is their inherent natural isolation from the environment, which helps reduce decoherence significantly. Institutions like the University of California, Berkeley, and others have been active in this field, pushing the boundaries of what's possible with ion traps. However, the complexity of the technology can limit scalability compared to other methods.
Advantages:
- Low error rates due to isolation
- Highly controlled operations
- Reliable entanglement over long distances
Disadvantages:
- Complexity in scaling to large qubit numbers
- Requires intricate laser setups
Topological Qubits
Topological qubits are built on the principles of topology and quantum field theory. They are crafted to be more resilient to errors, making them a fascinating area of research. The key characteristic of topological qubits lies in their ability to retain quantum information for longer periods due to their topologically protected nature. This feature theoretically allows for computation without the severe error corrections that other qubit types require.
The most noteworthy element of topological qubits is their reliance on anyons โ exotic particles that do not exist as traditional particles do. This uniqueness presents both promising potential and considerable hurdles in practical implementation. Microsoft is a notable player in this space, exploring its capabilities. However, the nascent technology and limited understanding pose challenges in realizing its promise.
Advantages:
- High resilience to environmental noise
- Potential for fault-tolerant quantum computing
Disadvantages:
- Still largely theoretical with many open questions
- High demands for material and experimental setups
Quantum Gates and Circuits
Quantum gates and circuits form the basis of quantum logic, analogous to classical logic gates used in traditional computing. They enable manipulation of qubits through a series of operations, allowing computations to be performed within quantum circuits. This setup is essential in translating quantum algorithms into executable tasks that lead to valuable outputs.
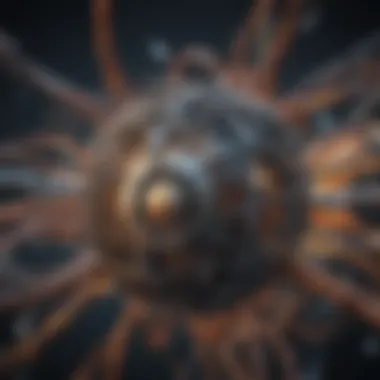
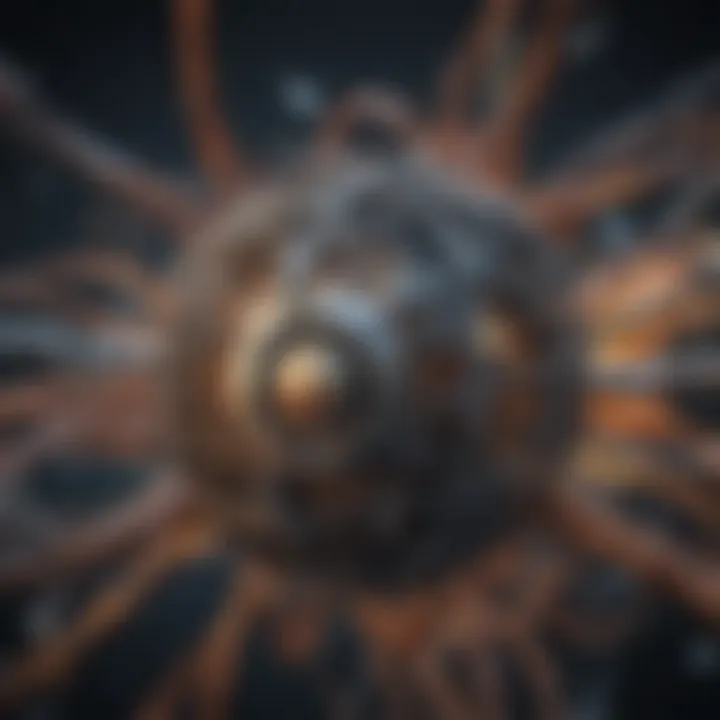
As researchers continue to explore the intricacies of quantum gates, the architecture of quantum circuits becomes vital in advancing our understanding of computation on this fascinating frontier.
How Quantum Computing Works
The mechanics behind quantum computing reveal a fascinating intersection of computation and physics. Understanding how quantum computing operates is crucial, especially considering its promise to solve problems traditional computers struggle with. At its core, quantum computing transcends classical algorithms through the unique processes involving quantum bits, or qubits, and algorithms meticulously crafted for their extraordinary capabilities.
Quantum Algorithms
The algorithms that power quantum computing essentially serve as the instruction sets that leverage the principles of quantum mechanics. These algorithms allow quantum computers to perform calculations at remarkable speeds, opening doors to advancements in various domains. Two noteworthy quantum algorithms often discussed are Shor's Algorithm and Grover's Algorithm, each playing pivotal roles in demonstrating quantum computing's potential.
Shorโs Algorithm
Shor's Algorithm represents a significant breakthrough in factoring large integers efficientlyโsomething that classical computers would require an impractically long time to achieve. The key characteristic of Shorโs Algorithm lies in its ability to factor numbers exponentially faster than the best-known classical methods. This makes it an essential algorithm in the realm of quantum computing, especially in the context of cryptography.
What's unique about Shorโs Algorithm is its polynomial time complexity, contrasting starkly with classical approaches. This efficiency raises concerns about current encryption protocols relying on factoring large numbers, such as RSA. While Shorโs Algorithm showcases quantum computing's capability, it also highlights the critical advantage of speed and effectiveness in solving specific problems. However, it is worth noting that its actual implementation on large scales remains a technical challenge, suggesting that while promising, it is not yet a widespread solution.
Groverโs Algorithm
On the other hand, Groverโs Algorithm shines in the search domain, providing a robust method to search unstructured databases. A fundamentally beneficial aspect of Groverโs Algorithm is its ability to reduce search timesโsolving problems quadratically faster than classical algorithms. This algorithm is particularly beneficial for optimizing search functions, be it in database management or optimization scenarios.
One unique feature of Groverโs Algorithm is that it can be applied to various tasks beyond simple database searches, rendering it versatile. Nevertheless, classical algorithms often suffice for smaller datasets, bringing into question the practical necessity of implementing Groverโs Algorithm until larger datasets are commonplace in various fields.
Error Correction in Quantum Computing
Error correction is of utmost importance in quantum computing, where the delicate state of qubits can be easily disturbed by their environment. Unlike classical bits, which can handle noise without losing integrity, qubits are highly susceptible to errors during calculations. To address this, quantum error correction codes have been devised to protect information, maintaining the fidelity of operations. This area of quantum computing examines strategies that can correct errors without the need to measure and thus collapse the quantum stateโan innovative leap crucial for practical applications.
In summary, understanding the workings of quantum computing hinges on delving into its algorithms and error correction methods. By grasping how algorithms like Shorโs and Groverโs enhance the capabilities of quantum computers, one gains insight into their potential impact across various sectors, from security to search optimizations. With ongoing advancements, the future holds promises that stretch beyond current capabilities.
Applications of Quantum Computing
The leap from classical computing to quantum computing isn't just a step; it's more like a leap into a new dimension. This fresh approach introduces a realm of possibilities, bringing along unique applications that stand to reshape various sectors. In this section, we will explore how quantum computing holds the key to breakthroughs, especially in nuanced fields like cryptography, medicine, and machine learning. Understanding these applications clarifies not only what quantum computing can do but also the profound implications it has for the future.
Cryptography
One of the most talked-about applications is in the field of cryptography. Traditional encryption methods rely heavily on mathematical complexity. For instance, modern encryption hinges on the difficulty of problems like factoring large integers, which is a task that classical computers find arduous, if not impossible, for immense numbers. Enter quantum computing. Using algorithms like Shor's Algorithm, quantum computers can achieve this feat significantly faster than classical counterparts, effectively breaking many of todayโs encryption protocols in the process.
Imagine a future where transactions, communications, and even personal data are at risk because of a technology that can decode your secrets almost instantly. This draws us toward the significance of quantum-resistant encryption methods that are currently under development. Thereโs ongoing research aimed at devising techniques that will safeguard sensitive information even in a quantum world. The idea is to adapt and evolve, creating new cryptographic methods that leverage the principles of quantum mechanics, thus ensuring data remains secure.
Medicine
Next up on our radar is medicine, a field where quantum computing could lead to groundbreaking advancements. Consider drug discovery, for example. The process of understanding molecular structures and interactions can take years and plenty of resources. Quantum algorithms hold the potential to model molecular interactions at a level of detail that is simply unattainable with classical computations. This means quicker identification of viable drug candidates and a faster route to market.
Furthermore, quantum computing could streamline problems related to personalized medicine. By analyzing complex biological data more efficiently, it can help tailor treatment plans based on individual genetic makeups. On a broader scale, the implications for predictive healthcare are colossal. The ability to simulate biological processes and environmental factors allows for more informed decisions, ultimately increasing the effectiveness of treatments.
Machine Learning
Finally, let's dive into machine learning, another domain ripe for quantum enhancement. Classical machine learning techniques can struggle with heavy data loads and complex models. Quantum computing flips this narrative on its head. For instance, quantum algorithms can process large datasets and optimize model training much faster than their classical counterparts.
Notably, quantum computing facilitates a paradigm shift in clustering methods and classification tasks. Consider applications in predictive analytics, where companies could anticipate market trends or customer behaviors with unprecedented accuracy. Moreover, the blending of quantum mechanics and neural networks is an exciting frontier, promising to yield models that might outperform classical approaches in numerous applications.
"Quantum computing promises a world where data analysis is not just fast, but fundamentally different in what it can achieve."
Current Challenges in Quantum Computing
Navigating the intricate landscape of quantum computing is far from a walk in the park. Despite the promises this revolutionary technology holds, several challenges need to be tackled. Understanding these roadblocks is not only imperative for researchers and developers, but also for anyone interested in the future of computation. This section explores two key areas: technical limitations and scalability issues. These elements are critical in understanding the broader implications of quantum computing.
Technical Limitations
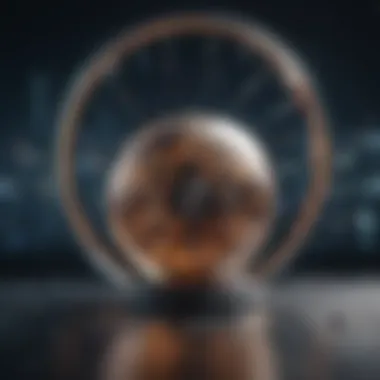
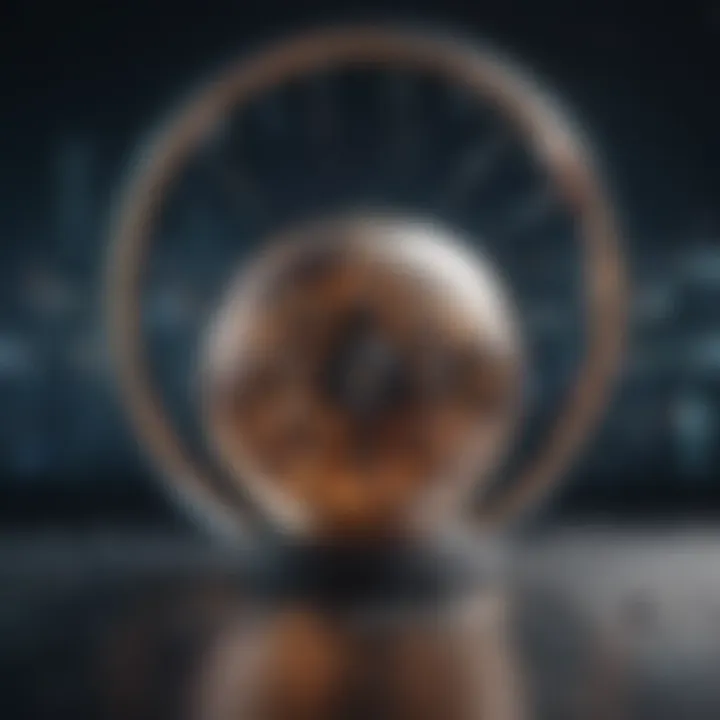
Technical limitations encompass a variety of hurdles that impede the progress of quantum computing technology. One primary issue lies in the fragility of qubits. Unlike classical bits, which can easily maintain their state of either 0 or 1, qubits exist in a superposition of both states. However, they are easily disturbed by their environment, leading to what is known as decoherence. This phenomenon results in loss of information and, ultimately, computational accuracy.
Moreover, developing quantum hardware that is both stable and scalable poses another hurdle. For instance, superconducting qubits require extremely low temperatures to function correctly. The complexity of maintaining such conditions can limit practical applications. Similarly, ion trap technologies, albeit promising, also struggle with limitations in qubit connectivity and error rates.
Some other critical technical repercussions include: Error rates: Current quantum computers exhibit higher error rates than classical computers. High error rates can result in unreliable outcomes, which makes practical deployment a significant concern. Limited qubit coherence times: Qubits decay rapidly, limiting the time available for computations.
"The path to quantum supremacy is dotted with pitfalls; overcoming them requires not just innovative thinking but also a collaborative effort across disciplines."
Scalability Issues
The conversation around scalability is crucial when considering the future possibilities of quantum computing. Current quantum computers have only a handful of qubits, which is far from sufficient for practical applications. Scaling up the number of qubits while maintaining performance integrity is a daunting task. Transitioning from a few qubits to a large number of operational qubits involves various complexities.
A few notable challenges in scalability include:
- Interconnectivity: As the number of qubits increases, the requirement for each qubit to interact with others grows exponentially. Designing systems that allow for effective communication between a large number of qubits is particularly tricky.
- Error correction: With many qubits in use, the impact of error correction becomes vital. Each additional qubit can significantly add to the complexity of the error-correcting codes needing to be implemented.
- Physical space and infrastructure: Building quantum computers with substantial numbers of qubits may also demand extensive physical space and specialized infrastructure. This can lead to increased costs and resource requirements.
In summary, while the flickering light of quantum computing holds the potential to illuminate numerous fields, it is crucial to shed light on the challenges that lay along the pathway to its widespread adoption. As researchers and engineers continue to strategize around these limitations and scalability hurdles, the dialogue remains open on the future implications of quantum computing. The road may be rugged, but every challenge presents an opportunity for innovation.
The Future of Quantum Computing
The future of quantum computing is a promising and multifaceted arena that could reshape the technological landscape as we know it. Understanding this trajectory is critical for anyone engaged in science and technology. As we venture deeper into the realm of quantum mechanics, several key elements emerge that hold substantial implications for various industries.
One of the paramount aspects to consider is the pace of innovation in quantum hardware. Companies are racing to develop more reliable and scalable qubits, essential for creating powerful quantum computers. Firms such as IBM and Google are democratizing access, offering cloud-based quantum computing services. This accessibility allows researchers and developers to experiment without the need for expensive hardware, spurring innovation across a broad spectrum of applications.
Moreover, the development of quantum algorithms tailored for specific tasks is also set to accelerate. Developers are not just working on existing algorithms but are also exploring creative solutions that could exploit quantum properties in novel ways. This evolution will likely enhance the efficiency of various computational tasks, enabling breakthroughs in areas like cryptography, optimization, and drug discovery.
Predicted Trends
The trajectory of quantum computing is expected to exhibit several notable trends in the coming years:
- Increased Integration with Classical Systems: Rather than replacing classical computing, quantum systems will likely work alongside them, handling specialized tasks that require quantum proficiency.
- Enhanced Error Correction: Advances in quantum error correction will allow for more stable qubit performance, mitigating one of the significant challenges currently faced.
- Broader Industry Adoption: As understanding and interest grow, industries ranging from finance to agriculture will start to adopt quantum solutions for complex problem solving.
- Collaboration between Public and Private Sectors: Ongoing partnerships between governments and tech firms will not only drive funding but also bolster research initiatives that promote quantum literacy and application.
These trends signify a shift toward a more integrated technological future where quantum computing does not just exist in isolation but interacts with broader systems in profound ways.
Potential Impact on Society
The potential societal impacts of quantum computing are vast and varied. As this technology continues to evolve, it stands to influence several critical areas:
- Revolutionizing Healthcare: Quantum computing holds the potential for significant advancements in personalized medicine, enabling tailored treatments through accelerated drug discovery processes.
- Transforming Financial Services: With enhanced computational power, quantum algorithms could revolutionize risk assessment and financial modeling, potentially leading to more stable economic systems but also raising ethical concerns around data privacy and algorithmic bias.
- Driving Sustainability: By solving complex optimization problems, quantum computing could help tackle significant environmental challenges such as climate modeling, resource allocation, and energy efficiency.
- Educational Empowerment: As accessibility and understanding of quantum principles increase, educational institutions may implement quantum curricula, fostering a new generation of quantum-savvy professionals.
"The convergence of quantum technologies with everyday life holds a transformative power that will touch various aspects of society, from how we work to our fundamental understanding of the universe."
In assessing these impacts, it is prudent to remain vigilant regarding the ethical, societal, and economic implications of quantum advancements. Thus, engaging in dialogue about responsible quantum research and application will be crucial for harnessing its full benefits.
Ending
In recognizing the significance of quantum computing, it's essential to synthesize the core elements discussed throughout this article. Quantum computing stands at the forefront of technological evolution. Acknowledging its foundationsโqubits, superposition, and entanglementโprovides deeper comprehension of its mechanisms. The rapid advancements in the field not only herald a new era of computational capability but also raise numerous questions regarding ethical implications and accessibility.
Summary of Key Points
The article covered pivotal topics that illuminate the landscape of quantum computing. Below are the crucial takeaways:
- Defining Quantum Computing: Understanding how quantum computers differ fundamentally from classical counterparts sets the stage for further exploration.
- Quantum Mechanics Basics: A solid grasp of fundamental principles of quantum mechanics is crucial, especially the concepts of quantum states, superposition, and entanglement.
- Architecture Insights: Different architectures, such as superconducting and ion-trap qubits, illustrate the diverse approaches to building quantum computers.
- Applications Explored: The potential applications across fields like cryptography, medicine, and machine learning highlight how quantum technology can address complex problems.
- Challenges Identified: Recognizing ongoing technical limitations and scalability issues is vital to fostering realistic perspectives on future developments.
- Future Outlook: Anticipated trends within quantum technology help frame discussions about its broader societal impact.
In essence, the intersection of quantum computing and real-world application is fraught with uncertainties and opportunities alike.
Final Thoughts
Reflecting on the journey into quantum computing, it's clear that this field is much more than just a technological curiosity; it has implications that stretch across numerous dimensions of society. As research progresses, so does our understanding of complex problems and solutions that traditional computing cannot feasibly handle. While we stand on the brink of a quantum revolution, it's imperative that discussions on ethical frameworks, accessibility, and sustainable practices follow suit. The future will require dialogue that transcends just the technicalโcalling for an interdisciplinary approach that encompasses science, ethics, and policy-making.
"In every great advancement, the human element remains at the heart of innovation."
As students, researchers, educators, and professionals dive deeper into this realm, maintaining a balance between enthusiasm and caution will be key. The journey into the heart of quantum computing does not just seek to understand a new technology; it aspires to build a future where such advancements are integrated thoughtfully into our daily lives.