Exploring the Higgs Boson Field and Its Impact
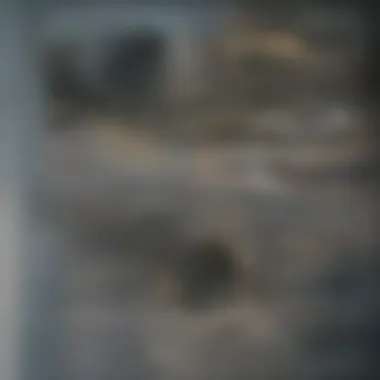
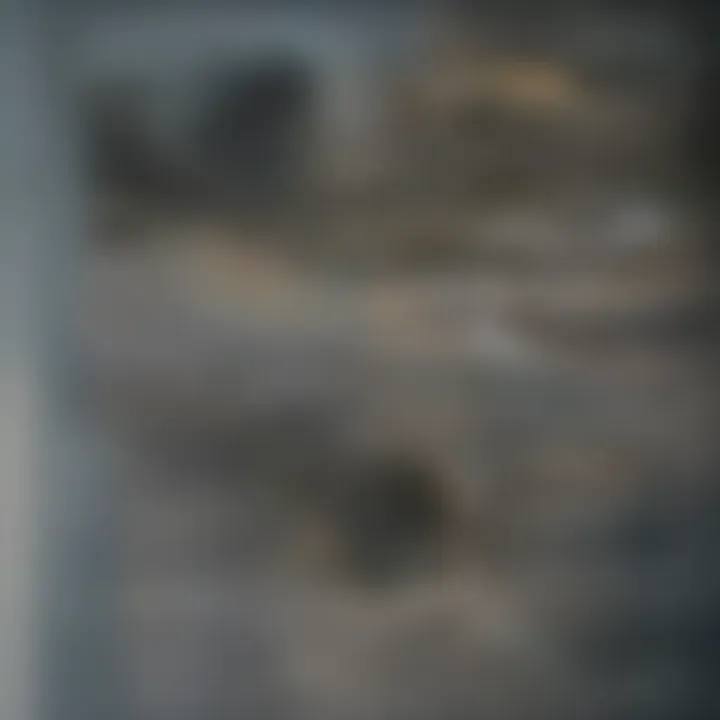
Intro
The Higgs boson field has emerged as a crucial component of modern particle physics. Discovered in 2012 at CERN's Large Hadron Collider, this field is integral to understanding how particles acquire mass. This phenomenon is essential for the consistency of the Standard Model of particle physics. The importance of the Higgs boson extends beyond mere mass acquisition; it invites questions about the very fabric of the universe, the forces that govern it, and the potential for new physics beyond current theories.
This article aims to unravel the complexities surrounding the Higgs boson field, covering theoretical insights, detection strategies, and broader implications for physics. By providing detailed information, readers will gain a comprehensive perspective on this pivotal topic, which underpins not only theoretical pursuits but also experimental investigations in the field of high-energy physics.
Key Research Findings
Overview of Recent Discoveries
Recent advancements have shed light on the properties of the Higgs boson and the field associated with it. Notable findings include:
- Couplings with Other Particles: Studies have confirmed the coupling of the Higgs boson with various quarks and leptons, reinforcing its role in the mass generation mechanism.
- Higgs Self-Coupling: Observations from the LHC point to the possibility of self-coupling of the Higgs boson, indicating the existence of a complex potential landscape and its implications for scalar fields.
- Search for New Physics: Ongoing experiments are probing beyond the standard model, looking for potential exotic particles or phenomena that may emerge from the Higgs field.
Significance of Findings in the Field
These discoveries are significant because they bolster our understanding of particle interactions and contribute to the validation of the Standard Model.
"The Higgs boson serves as a cornerstone in theoretical frameworks, providing insights into both mass and the unification of forces."
The exploration of the Higgs field raises many unresolved questions. For example, its relation to dark matter and energy remains an intriguing topic for further research. As researchers continue to analyze data from high-energy collisions, they focus on potential deviations from the predictions made by existing theories.
Breakdown of Complex Concepts
Simplification of Advanced Theories
Understanding the Higgs boson field requires an appreciation of several advanced concepts in physics. Here, we break down some of these ideas:
- Spontaneous Symmetry Breaking: This key idea explains how particles can attain mass through the Higgs mechanism. When the Higgs field acquires a non-zero value, symmetry is 'broken,' leading to the mass properties of particles.
- Vacuum Expectation Value: The vacuum expectation value of the Higgs field is responsible for the mass of fundamental particles. This value is critical in describing the stability of the field.
Visual Aids and Infographics
Visual aids are often helpful for conceptualizing complex theories. Infographics explaining the interaction between the Higgs boson and other particles can elucidate the ideas without overwhelming detail.
For more detailed visual representations, readers may refer to resources such as Wikipedia on Higgs boson and Britannica articles.
Ending
The Higgs boson field stands at the forefront of theoretical and experimental physics, bridging gaps between abstract concepts and empirical validation. As research progresses, it remains an area ripe for exploration. Each discovery not only enhances our understanding of fundamental particles but also sets the stage for future theoretical innovations.
Foreword to the Higgs Boson Field
The Higgs boson field is a crucial element in modern physics, particularly in understanding the Standard Model. It represents a fundamental breakthrough in how we relate mass to particle physics. The discovery of this field brings many insights into the fundamental structure of matter.
Definition and Overview
The Higgs boson field, proposed by Peter Higgs and others in the 1960s, is a quantum field that permeates the universe. It is associated with the Higgs boson, a particle that was confirmed experimentally in 2012. Essentially, the field is responsible for giving mass to elementary particles through their interactions with it. When particles move through this field, they acquire mass as they interact with its energy. This mechanism provides a key part of our understanding of how particles obtain mass and, thus, form the building blocks of the universe.
The Higgs boson itself is often referred to as the "God particle," a nickname that emphasizes its vital role in the mass generation mechanism. However, this term can be misleading. The boson reflects a deeper underlying symmetry of nature that researchers continue to explore.
Importance in the Standard Model
The Higgs boson field holds substantial importance in the Standard Model of particle physics. This model describes the fundamental forces and particles that compose our universe,
"The existence of the Higgs field solidifies the Standard Model, bridging gaps between theoretical predictions and experimental evidence."
Without it, the model cannot adequately explain how particles acquire mass. In essence, particles would behave as if they were massless, fundamentally altering our understanding of interactions between them.
The confirmation of the Higgs boson also validates the principles of gauge theories and spontaneous symmetry breaking in the framework of the Standard Model. Such breakthroughs have profound implications, not only for particle physics but also for cosmology, providing insights into the early universe's conditions.
In summary, the Higgs boson field is indispensable for grasping the intricate dance of particles and forces that govern existence as we know it. Understanding this field and its implications sets the foundation for further exploration in physics, promising to unveil even more secrets of the cosmos.
Historical Context
The historical context surrounding the Higgs boson field is crucial for understanding its significance in particle physics. The journey to the Higgs mechanism reflects a series of developments that reshaped our conception of elementary particles and their interactions. Recognizing these events helps to frame the scientific breakthroughs we see today.
Development of the Higgs Mechanism
The Higgs mechanism, proposed in the early 1960s, originated from the need to understand how particles acquire mass. Prior to this advancement, physicists struggled with inconsistencies in the Standard Model of particle physics. The ideas were developed by various scientists, among them Peter Higgs, FranΓ§ois Englert, and Robert Brout. Their collective work provided a unified explanation integrating the weak force and electromagnetism. The key breakthrough was the introduction of a scalar field, now known as the Higgs field, which permeates all of space.
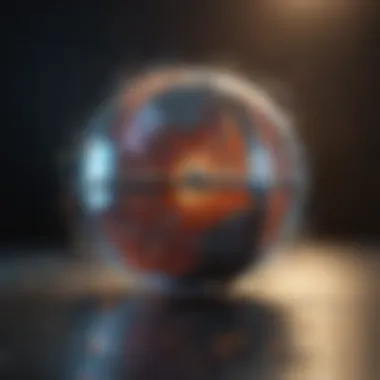
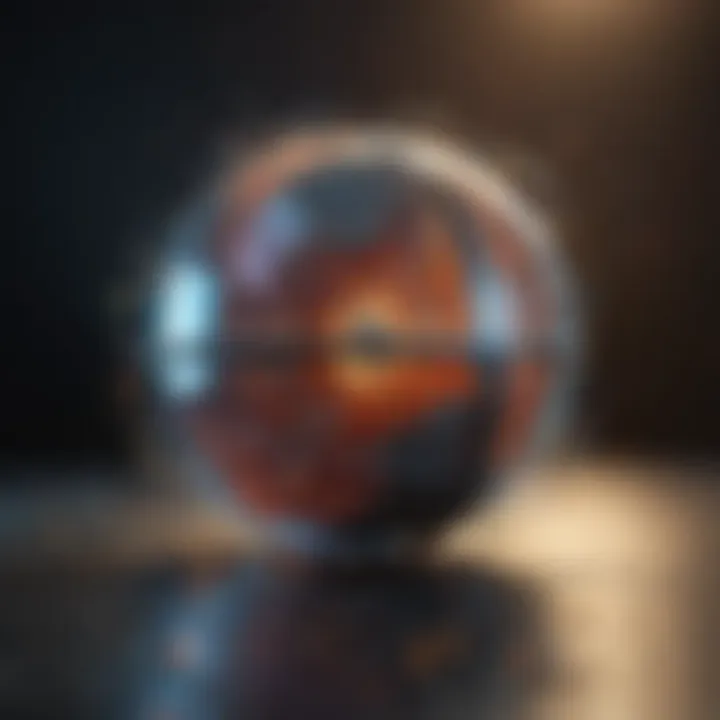
This mechanism showed that particles can gain mass through their interactions with this field. When certain particles traverse the Higgs field, they interact in such a way that they experience resistance resembling friction, which manifests as mass. This concept not only resolved theoretical issues but also laid a path for experimental verification. Understanding its historical development reinforces the importance of scientific collaboration and exploration in fundamental physics.
Key Figures and Milestones
Numerous scientists contributed to the development of the Higgs boson concept. Some key figures include:
- Peter Higgs: Introduced the theoretical framework in 1964, which included a mechanism for mass generation.
- FranΓ§ois Englert and Robert Brout: Independently developed similar ideas in the same year, laying foundational work essential for understanding particle mass.
- Gerry Guralnik and C.R. Hagen: Helped refine the mechanisms that solidified the theoretical basis of the Higgs boson.
Milestones along this journey include:
- 1973: The completion of the electroweak theory, incorporating the Higgs mechanism.
- 2012: The discovery of the Higgs boson at CERN's Large Hadron Collider confirmed the theoretical predictions.
Each of these milestones underscores the collaborative efforts across decades that were foundational to our current understanding of particle physics.
After a period of speculation and research, the confirmation of the Higgs boson fundamentally altered our understanding of mass and the universe.
This exploration into the historical context underscores that the Higgs boson field is not merely a footnote in physics; it is central to the fabric of modern theoretical frameworks. Understanding its origin provides insights into ongoing research and future questions in the realm of high-energy physics.
Theoretical Foundations
The theoretical foundations of the Higgs boson field serve as the backbone in understanding its role in particle physics. These principles are pivotal for grasping why the Higgs boson is not just a curious particle but a fundamental necessity in the Standard Model of particle physics. The inquiry into quantum fields and mass generation unravels complexities that explain how particles acquire mass and interact with fundamental forces. This section will illuminate these concepts, providing insight into both the existing frameworks of particle physics and the various implications that arise from them.
Quantum Field Theory and the Higgs Field
Quantum Field Theory (QFT) is a crucial framework in modern physics. It allows the description of particles not as isolated entities but as excitations in underlying fields. Within this context, the Higgs field is a specific quantum field permeating the entire universe. Its importance lies in the way it interacts with other particles, providing them with mass through a process known as spontaneous symmetry breaking.
In QFT, every particle can be seen as a quantum of its respective field. The Higgs boson, as an excitation of the Higgs field, emerges when the field is disturbed. This means that the properties of the Higgs field have profound implications on how we understand particle interactions. For example, when a particle moves through the Higgs field, it interacts with this field, leading to an effective mass. Heavier particles interact more strongly with the Higgs field, while lighter ones interact weakly.
Furthermore, the significance of the Higgs field lies beyond just particle mass. It plays a crucial role in explaining the structure of the universe itself, including how particles acquire mass differentially. This explains the diversity of observed particles and interactions in the universe. The theoretical constructs of QFT, therefore, not only provide a foundation for defining the Higgs boson but also frame our understanding of the universe's interactions at the most fundamental level.
Mass Generation Mechanism
The process of how mass is generated via the Higgs field is fundamental to the Standard Model. This mechanism, known as the Higgs mechanism, allows particles to gain mass without violating gauge invariance, a principle crucial for maintaining the symmetries in particle physics. The elegance of the Higgs mechanism lies in its simplicity. When the Higgs field obtains a non-zero value, it breaks the electroweak symmetry, which in turn allows W and Z bosons to acquire mass.
An important aspect of this mechanism is the relationship between mass and energy. According to Einsteinβs famous equation, energy and mass are interchangeable. The energy invested in interacting with the Higgs field manifests as mass for various particles. Thus, without the Higgs field, electroweak particles would remain massless, rendering the universe radically different than we observe today.
- The contributions of the Higgs mechanism include:
- Explanation of differences in mass among particles.
- Maintenance of the underlying symmetries observed in particle interactions.
- Allowance for the formation of atoms and molecules, hence the existence of matter as we know it.
The Higgs field effectively serves as a cosmic molasses, providing resistance to otherwise massless particles as they move through it. This process has been key to not only shaping particle physics but also to influencing cosmological models that seek to explain the formation of the universe.
"The discovery of the Higgs boson validated the theoretical framework that had been under question for decades; it opened new avenues in fundamental physics beyond the Standard Model."
In summary, the theoretical foundations concerning the Higgs boson field and its associated mechanisms are not merely academic exercises. They have profound implications on our understanding of the physical universe, leading to ongoing explorations of what lies beyond the accepted frameworks of particle physics.
Detection of the Higgs Boson
The detection of the Higgs boson represents a crucial aspect of modern particle physics. Understanding how this fundamental particle was discovered allows researchers to assess the ramifications of its existence. Moreover, the techniques employed to detect the Higgs boson reflect the ingenuity and technical advancements in experimental physics. The process not only offers insight into the Higgs field but also solidifies our understanding of the Standard Model, which is the leading theory describing the fundamental forces and particles.
Experimental Techniques
To successfully detect the Higgs boson, various experimental techniques are utilized. These include high-energy particle collisions that help to create conditions necessary for the boson to emerge briefly before decaying into other particles. One key technique is called collider experiments. In these experiments, proton beams are accelerated to nearly the speed of light and collided at extraordinarily high energies.
Another important method involves particle identification. When the Higgs boson decays, it results in various particles like photons, Z bosons, and W bosons. Detectors need advanced capabilities to distinguish these specific decay signatures from background noise. The ATLAS and CMS collaborations at the Large Hadron Collider (LHC) have played a vital role in implementing these techniques. They utilize sophisticated tracking systems and calorimeters to accurately measure the energies and momenta of the resulting particles.
Key Techniques to Note:
- Collider Experiments: High-energy collisions producing transient Higgs bosons.
- Trackers: Devices that identify particle trajectories post-collision.
- Calorimeters: Instruments that measure the energy of particles emitted in decays.
- Data Analysis Algorithms: Sophisticated software that extracts meaningful information from massive datasets.
The Role of the Large Hadron Collider
The Large Hadron Collider is pivotal in the journey of Higgs boson detection. Launched in 2008, it is the worldβs largest and most powerful particle accelerator, designed specifically to explore the deep mysteries of particle physics. The LHC facilitates collisions between protons at unprecedented energies, significantly increasing the chances of Higgs boson production.
The collider operates by accelerating two beams of protons in opposite directions within a 27-kilometer ring underground. These high-energy collisions, occurring billions of times per second, provide the experimental conditions needed for the Higgs boson to be produced. While the Higgs boson itself decays rapidly, its decay products can be measured and analyzed in detail, confirming its presence indirectly.
"The discovery of the Higgs boson was a milestone that confirmed a fundamental aspect of particle physics and the mass generation mechanism."
Discoveries and Observations
The exploration of the Higgs boson field has led to significant discoveries and observations that enhance our understanding of fundamental physics. These findings not only confirm predictions made by the Standard Model but also pave the way for deeper inquiries into the nature of the universe. Each experimental result acts as a building block, contributing to a larger framework that researchers are eager to explore.
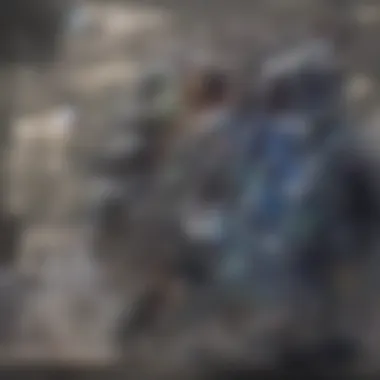
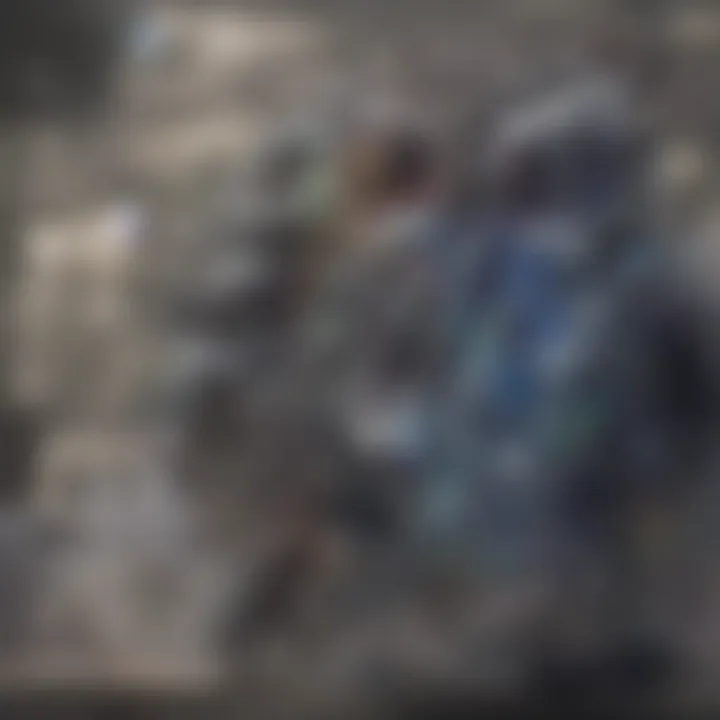
Key Experimental Results
Among the notable experimental results, the 2012 announcement of the Higgs boson discovery at CERN marked a critical milestone. Scientists observed a particle consistent with the Higgs boson through data collected at the Large Hadron Collider. The particle's mass was measured to be approximately 125 giga-electronvolts, fitting predicted ranges outlined in theoretical models.
Additional significant findings involve variations in decay channels of the Higgs boson. Observations show that the Higgs decays into various particles like photons, W bosons, and Z bosons. Each decay channel provides unique insights into the properties of the Higgs field.
Moreover, ongoing studies continue to refine the understanding of the Higgs boson's coupling with other particles. This leads to implications regarding the broader implications for particle interactions in the universe.
Confirming the Higgs Boson Existence
Confirming the existence of the Higgs boson involves multiple aspects, such as precision measurements of its properties. The consistency between the observed data and theoretical predictions strengthens the case for the particle's existence. As physicists analyze heavy Higgs production and its subsequent decay processes, they leverage statistical methods to ensure reliability of the findings.
The agreement of experimental data with theoretical models supports beyond doubt the role of the Higgs boson in endowing mass to elementary particles. In addition, experiments have scrutinized the Higgs boson in various contexts, allowing a deeper investigation of phenomena like electroweak symmetry breaking. These confirmations extend not only to the Higgs boson but also validate the coherence of the Standard Model.
The discoveries surrounding the Higgs boson are not merely academic; they hold profound significance for understanding fundamental questions in physics, such as the origins of mass and the evolution of the universe. Continuing research seeks to uncover anomalies or discrepancies that could indicate new physics, possibly guiding future theories beyond the Standard Model.
"The Higgs boson has fundamentally reshaped our grasp of the universe."
In summary, the discoveries and observations of the Higgs boson play a pivotal role in confirming established theories while opening avenues for future exploration. As research progresses, it ensures that our understanding of the universe remains dynamic and evolving.
Properties of the Higgs Boson
The properties of the Higgs boson are an essential aspect of understanding its role in physics. This section explores the mass and spin characteristics of the Higgs boson as well as its interactions with other particles. Both aspects play a crucial role in how we comprehend the Higgs mechanism and its significance within the Standard Model of particle physics.
Mass and Spin Characteristics
The Higgs boson has a mass of about 125 giga-electronvolts (GeV). This value is significant; it determines how particles acquire mass through interactions with the Higgs field. According to the Higgs mechanism, particles gain mass by interacting with this field, the strength of which correlates directly with the mass of the particle.
Massiveness is what distinguishes the Higgs from other scalar particles in theoretical physics. Its spin is zero, which means it is a scalar boson. Unlike fermions, which follow the Pauli exclusion principle, bosons can occupy the same quantum state. This property is important for the behavior of fields and for the particles resulting from interactions in particle physics.
The Higgs boson's mass and spin characteristics are central to our understanding of mass generation in the universe.
Interactions with Other Particles
The interactions of the Higgs boson with other particles provide an essential understanding of its role in the universe. It couples particularly strongly with massive particles, such as the W and Z bosons, and top quarks. This means that the more massive the particle, the stronger its interaction with the Higgs field. Conversely, light particles like electrons interact weakly. This differential interaction leads to a complex landscape of mass relationships and particle behavior.
There are several aspects to consider about interactions:
- Role in mass generation: Without the Higgs field, fundamental particles such as W and Z bosons would be massless, which would alter the fabric of particle interactions.
- Decays and production: The Higgs boson can decay into various particles, including photons and other bosons, which is crucial in experimental detection.
- Implications for new physics: Understanding these interactions can reveal potential physics beyond the Standard Model, such as the behavior of dark matter or extended theories in particle physics.
The analysis of these interactions remains a significant aspect of current research and future explorations in particle physics.
Implications for Physics
The implications of the Higgs boson field extend far beyond its role in the Standard Model of particle physics. Understanding these implications is essential for grasping the broader context of modern physics and its potentially revolutionary consequences.
Effects on the Standard Model
The Higgs boson is a cornerstone of the Standard Model, which describes the fundamental particles and forces that constitute the universe. It provides the mechanism for mass generation through the Higgs mechanism. When particles interact with the Higgs field, they acquire mass. This integration explains why some particles, like the W and Z bosons, are heavy, while others, like photons, remain massless.
The discovery of the Higgs boson at CERN in 2012 confirmed a key prediction of the Standard Model. This event validated the theoretical framework that has been the foundation of particle physics for decades.
The implications for the Standard Model are profound. Without the Higgs boson, the mathematical consistency of the model would collapse. Furthermore, its properties may provide insight into unresolved issues, such as the unification of forces. As physicists analyze the Higgs boson further, they hope to uncover nuances that may indicate the presence of new physics.
Beyond the Standard Model Theories
While the Higgs boson consolidates the principles of the Standard Model, it also opens the door to theories that seek to extend our understanding beyond this framework. Notably, concepts like supersymmetry, string theory, and extra dimensions have arisen partly from the quest to reconcile the Higgs boson with other unanswered questions in physics.
Research into the Higgs boson may reveal new interactions or particles that do not fit neatly within the existing model. For instance, theorists speculate about the existence of dark matter candidates and how the Higgs field could interact with them. There is also discussion about the possible implications that the Higgs boson could have on cosmic inflation and the overall evolution of the universe.
"The discovery of the Higgs boson is only the beginning. It raises new questions and challenges our understanding of the universe at its most fundamental level."
As experiments continue at facilities like the Large Hadron Collider, new data may test the limits of the Standard Model. Results that deviate from predictions could indicate new physics and expand our understanding of the universe. Each finding regarding the Higgs boson could potentially shift paradigms and lead physicists towards groundbreaking theoretical advancements.
In summary, the implications of the Higgs boson field for physics are considerable. They touch upon the foundations of our theoretical constructs, the search for knowledge beyond established models, and the quest to unify disparate forces and particles in our understanding of the cosmos.
Ongoing Research and Future Directions
The realm of high-energy particle physics is continuously evolving. The study of the Higgs boson and its field remains a central theme in modern physics, making ongoing research particularly vital. Investigating the Higgs boson provides insights not only into particle interactions but also into the fundamental structure of the universe. Scientists are probing deeper into its properties, interactions, and implications to uncover hidden layers of physical reality beyond the established theories.
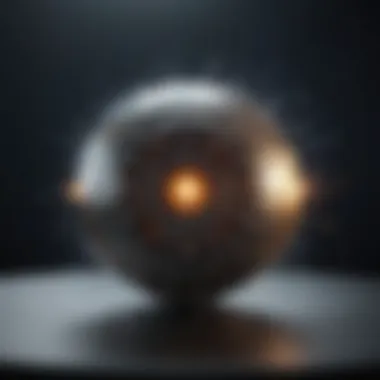
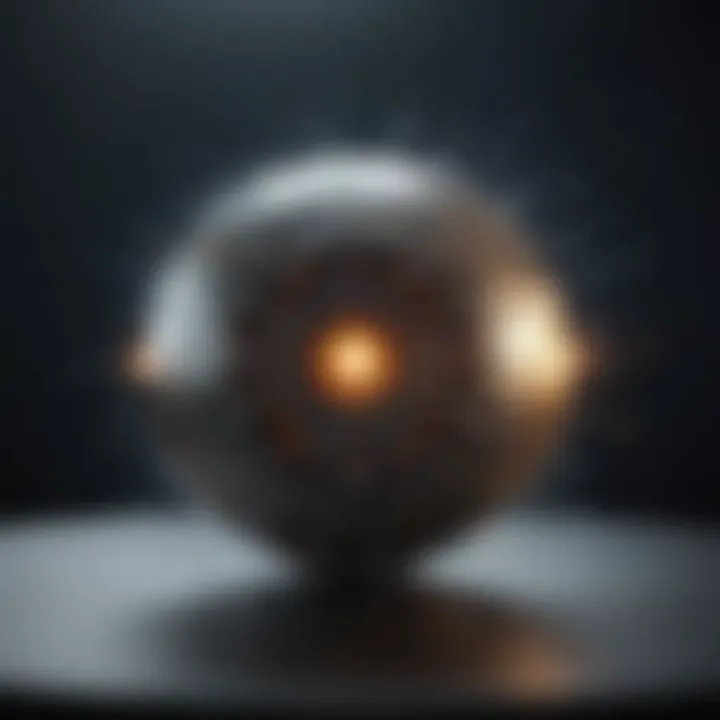
Current Experiments and Findings
Current experiments focused on the Higgs boson are crucial for validating theoretical predictions and extending understanding of the Standard Model. Institutions like CERN are at the forefront of these experiments. Researchers utilize the Large Hadron Collider to produce collisions at unprecedented energies, allowing for detailed studies of the Higgs field's interactions.
Recent observations from experiments have provided new insights:
- Higgs Decay Channels: Scientists analyze various decay channels of the Higgs boson, such as decay into photons or bottom quarks. This helps them understand the Higgs boson's properties better.
- Search for Anomalies: Finding unexpected results could indicate physics beyond the Standard Model. Researchers are constantly evaluating data for signs of new phenomena.
- Precision Measurements: Ongoing experiments aim to measure the Higgs boson's mass and coupling constants with high precision. Such measurements can either solidify the current theories or suggest new ones.
These efforts have revealed valuable information, helping refine the theoretical framework surrounding the Higgs boson and enhancing our understanding of particle physics.
Potential Discoveries on the Horizon
The future holds significant promise for breakthroughs in Higgs research. As technology advances and methodologies improve, several potential discoveries could reshape our understanding of physics:
- Beyond Standard Model Physics: New particles and interactions may emerge from experimental results, challenging existing paradigms. Exploring supersymmetry or additional dimensions could unveil hidden aspects of the universe.
- Dark Matter Interactions: Investigations into the Higgs boson might provide clues about dark matter. The nature of dark matter remains one of the most pressing questions in cosmology, and the Higgs field could play a role.
- Cosmological Implications: Understanding the Higgs field's behavior in the early universe may shed light on cosmic inflation and the formation of structures in the universe. Addressing this phenomenon could transform current cosmological models.
Research into the Higgs boson is not just a quest for knowledge; it is a pathway to uncovering the deeper truths about the universe. The work being done today sets the stage for discoveries that may redefine the physical laws governing our reality.
The Higgs Boson in Cosmology
The relationship between the Higgs boson and cosmology is a vital area of study that links particle physics with the broader understanding of the universe. This connection helps illuminate the formation and evolution of structures in the cosmos. The Higgs boson plays a pivotal role in explaining why particles have mass, which in turn influences how matter interacts with energy throughout the universe.
Connections to the Early Universe
In the early universe, shortly after the Big Bang, conditions were extreme. The energy levels were so high that all fundamental forces were thought to be unified. As the universe expanded and cooled, symmetries began to break, leading to the formation of distinct particles and forces. The Higgs field is fundamental in this respect. It provides the mechanism that gives mass to particles through the Higgs mechanism.
This time period is critical because if particles did not acquire mass, they would not be able to form atoms, stars, galaxies, and ultimately the complex structures we observe today. Research into the Higgs boson helps to answer questions about mass acquisition during the universe's infancy and informs theories about how the early universe developed.
Role in Cosmic Evolution
The Higgs boson continues to influence the evolution of the universe on a cosmic scale. Its interactions contribute to the mass of elementary particles, which affects how these particles clump together under gravity. When particles gain mass, they can form larger structures, transitioning from simple quarks and electrons to protons, neutrons, and eventually atoms.
Here are some notable implications of the Higgs boson on cosmic evolution:
- Formation of Atoms: Without the mass that the Higgs mechanism provides, matter would behave differently, preventing the stable formation of atoms.
- Star Formation: The mass of particles influences temperature and pressure mechanics in stellar formation. Stars, being the building blocks of galaxies, heavily depend on these processes.
- Galactic Structures: The way galaxies form and evolve is directly influenced by the properties of mass-generating particles.
The interactions governed by the Higgs field underpin the fabric of the universe, influencing everything from the smallest particles to the largest cosmic structures.
In closing, exploring the Higgs boson's role in cosmology offers a profound understanding of how the universe was shaped. It links the microcosmic behavior of particles to the macrocosmic structures we observe, solidifying its place as a cornerstone in the relationship between particle physics and cosmology.
Philosophical Considerations
The exploration of the Higgs boson field extends beyond the realm of particle physics, inviting deep philosophical inquiries. This component is crucial as it examines the broader implications of our scientific understanding. The philosophical considerations surrounding the Higgs boson challenge us to rethink not just what we understand about the universe, but also how we define reality itself.
The Nature of Reality in Physics
Philosophy and physics intersect notably when exploring the nature of reality. The existence of the Higgs boson reshapes what it means to be a particle. Reality, in traditional terms, is defined by observable phenomena and physical entities. Yet, the Higgs field challenges that perception by introducing a field that is omnipresent but not directly observable. While we can measure the boson itself, the fundamental field affecting mass remains abstract.
This leads to questions like: What constitutes reality in the absence of direct observation? Are theoretical entities, like the Higgs field, real if their effects can be observed, despite being inherently elusive? The implications of the Higgs boson thus extend into discussions about realism versus anti-realism in scientific theory, with some philosophers suggesting that if a theory is useful in explaining observations, it holds a kind of truth.
Implications on Fundamental Questions
The introduction of the Higgs boson influences multiple fundamental questions in science and philosophy. It speaks to the larger questions of existence and the cosmos. For instance, why do elementary particles have mass? The Higgs mechanism provides a framework to answer this question, indicating that mass is a property arising from interactions with a pervasive field.
Here are key considerations that arise:
- Nature of Mass: The Higgs field illustrates that mass is not an intrinsic property but a manifestation of interaction.
- Existence of Fields: The necessity of fields beyond the tangible encourages a reevaluation of what we perceive as components of the universe.
- The Limitations of Human Perception: The Higgs boson, while confirmed indirectly, highlights the constraints of human perception in science. What else could be true, yet undiscovered?
Ultimately, engaging with the philosophical dimensions of the Higgs boson may inspire more profound inquiries about the fabric of reality. In doing so, it challenges us to keep pushing the boundaries of understanding in both the physical and philosophical realms. This discourse remains vital for students, researchers, and thinkers who aim to explore not only the mechanics of particles but also the questions that lie at the very heart of our understanding of existence.
Closure
The conclusion of this article serves as a crucial summation of the multifaceted aspects surrounding the Higgs boson field, reflecting on why it remains a pivotal focus in modern physics. The synthesis of knowledge across various sectionsβranging from the theoretical foundations to experimental discoveriesβprovides readers with a coherent understanding of the complexities of the Higgs mechanism. It is not merely a retrospective examination; it encourages continuous inquiry into deeper implications and future applications.
The importance of the Higgs boson in the Standard Model cannot be understated. Its existence lends credence to the mechanisms by which particles acquire mass. As we delve deeper into topics such as quantum field theory and ongoing experimental endeavors, it becomes evident that comprehending the Higgs field is essential for unraveling further mysteries in particle physics.
Overall, the conclusion encapsulates the essence of what has been discussed throughout the article, reinforcing the understanding that ongoing research is crucial. As findings evolve, our comprehension of the universe and its fundamental principles also extends.
Summary of Key Points
- The Higgs boson field is fundamental to our understanding of mass in the universe.
- Its discovery at the Large Hadron Collider marked a significant milestone in particle physics.
- Theoretical frameworks like quantum field theory underpin its relevance in the Standard Model.
- Research continues to explore implications and applications beyond the Standard Model framework.
Outlook on the Future of Higgs Research
Looking forward, the study of the Higgs boson field promises to reveal new insights into both theoretical and experimental physics. Several key areas may present significant discoveries:
- Advanced Experiments: New technologies and methodologies are being developed to probe the properties of the Higgs boson more accurately.
- Beyond the Standard Model: Researchers are examining extensions and alternatives to the Standard Model, searching for phenomena that the current model does not explain.
- Cosmological Links: Understanding the connection between the Higgs field and cosmic evolution offers possibilities for insights into the early universe and dark matter.