Exploring the Big Bang: Causes and Implications
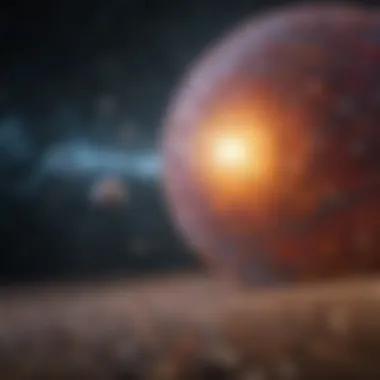
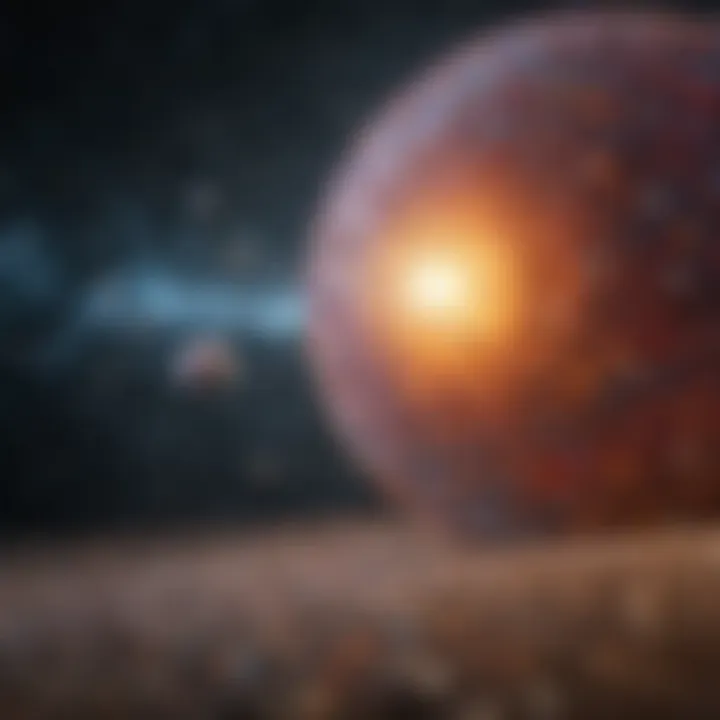
Intro
The Big Bang theory stands as a foundational concept in modern cosmology. It describes how the universe began from an extremely hot and dense state and has since expanded. This expansion continues today. While the theory is widely accepted, the exact causes of the Big Bang remain a subject of robust debate among scientists. Understanding this phenomenon is essential for grasping the complex mechanisms that govern our universe.
In this article, we will explore the causes of the Big Bang through various lenses. We will examine historical perspectives and contemporary theories, including the role of fundamental forces and quantum fluctuations. Each section aims to enhance the reader's comprehension of the rich, intricate tapestry of cosmological concepts.
The quest for knowledge about the universe's origin is not merely academic. It has profound implications for our understanding of time, space, and existence itself. By delving into these intricate matters, we hope to illuminate the various layers involved in the ongoing discourse surrounding the Big Bang.
Historical Context of the Big Bang Theory
Understanding the Historical Context of the Big Bang Theory is essential for comprehending how this idea became a central tenet in modern cosmology. Before the Big Bang concept emerged, various theories attempted to explain the structure and behavior of the universe. Each contributed to shaping the discourse around cosmological development. This context allows us to appreciate not only the strides made in scientific thought but also the socio-cultural and technological influences that guided research during these formative periods.
Early Theoretical Foundations
The origins of the Big Bang theory can be traced back to the early 20th century. Many early astronomers and physicists held a static view of the universe. The notion of a timeless and unchanging cosmos dominated. A critical shift occurred with Einstein's theory of general relativity in 1915. This theory suggested gravity's influence on the curvature of spacetime. However, Einstein himself favored a cosmological constant to maintain a static universe.
In 1922, the ideas of Russian mathematician Alexander Friedmann began changing perceptions. He proposed solutions to Einstein's equations that allowed for an expanding universe. Meanwhile, Belgian priest Georges Lemaître, in 1927, also suggested that the universe was expanding. He theorized a beginning state, which he described as the "primeval atom". These early theoretical foundations set a precedent for what would eventually become the Big Bang model.
The Role of Edwin Hubble
Edwin Hubble significantly advanced the positioning of the Big Bang theory in scientific thought. In the 1920s, he observed galaxies and discovered they appeared to be moving away from us. This implication was profound. It indicated that the universe was indeed expanding. Hubble's work helped validate the earlier predictions made by Friedmann and Lemaître. His formulation of Hubble's Law, which established a relationship between a galaxy's distance and its recessional velocity, fueled further acceptance of an expanding universe.
Hubble’s findings altered the trajectory of cosmological studies. His observations ushered in a new era of astrophysics, providing measurable evidence that contradicted previous static models of the universe. Through this lens, the expanding universe theory began to gain traction among scientists and laid the groundwork for understanding the Big Bang.
Advancements in Cosmological Models
As the 20th century progressed, advancements in technology and theory significantly refined cosmological models. The discovery of cosmic microwave background radiation in 1965 by Arno Penzias and Robert Wilson provided an unexpected yet critical piece of evidence supporting the Big Bang theory. This phenomenon was considerable proof that the universe had a hot, dense origin.
Further developments led to the formulation of the ΛCDM model, which incorporated dark energy and dark matter into the understanding of the universe's expansion. This model brought together various concepts and observations, providing a coherent framework to explain the evolution of the cosmos. Astrophysicists engaged in ongoing discussions and experiments, fine-tuning the model in light of new data.
Defining the Big Bang
Defining the Big Bang is crucial to understanding the foundational theories of cosmology. The term itself encapsulates a profound cosmic event that gave birth to our universe approximately 13.8 billion years ago. This section highlights key elements of this phenomenon and emphasizes its significance in comprehending the origins of time, space, and matter.
One major aspect of defining the Big Bang is recognizing its role as the initial singularity from which all matter and energy emerged. Without this event, the universe as we currently observe would not exist. This initial explosion set the stage for the expansion of space and the formation of galaxies, stars, and planetary systems. Understanding the Big Bang allows scientists to piece together the timeline of the universe's history and evolution.
Additionally, defining this event involves exploring various theories related to it. These theories help clarify the processes that followed the initial explosion and how our universe transitioned from hot, dense states to the cooler, more structured cosmos today. Key considerations include cosmic microwave background radiation, a left-over relic from this early phase, and the distribution of galactic structures.
"The Big Bang theory is the leading explanation about how the universe began. It describes how the universe expanded from an initial state of high density and temperature."
In this analysis, the following points will be explored:
- The essential characteristics of the Big Bang event
- Its implications for cosmic development and structure
- The critical questions it raises about the nature of existence itself
Understanding what occurred during the Big Bang is not just an academic pursuit but a quest that shapes fundamental questions about our reality. It enables researchers and educators to communicate complex ideas regarding the universe's origins effectively.
Quantum Mechanics and Cosmic Origins
The study of quantum mechanics offers profound insights into the origins of our universe, particularly when discussing the Big Bang. Quantum mechanics, a fundamental theory in physics, describes the behavior of matter and energy at the smallest scales. It provides a framework for understanding phenomena that classical physics cannot explain. In the context of cosmology, it raises intriguing questions about the state of the universe before the Big Bang and the mechanisms that may have triggered this monumental event.
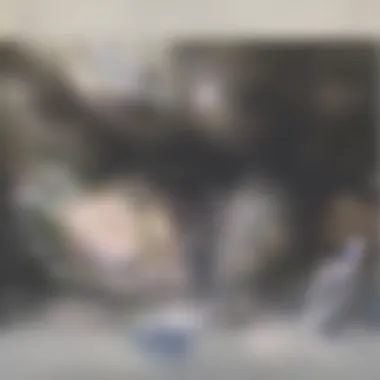
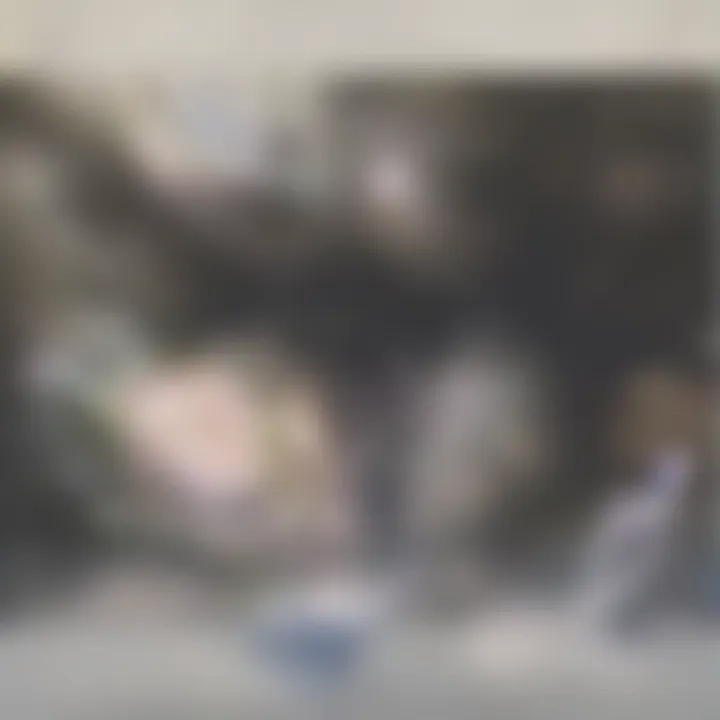
Key aspects include the understanding of quantum fluctuations and their role in cosmic evolution. By framing the discussion within quantum mechanics, researchers can explore theoretical possibilities that might have existed in the tumultuous moments leading to the Big Bang. This analysis is essential as it lays the groundwork for grasping complex cosmological theories and cosmogenesis.
Understanding Quantum Fluctuations
Quantum fluctuations refer to temporary changes in the energy levels of a point in space. This concept is significant for cosmology as it suggests that even a vacuum can possess energy due to inherent uncertainties in quantum mechanics. These fluctuations could be pivotal in the early universe, providing the seeds for the large-scale structure we observe today.
Several key points to consider include:
- Vacuüm Energy: Even empty space can contain energy, potentially leading to rapid expansions in the universe.
- Density Variations: Minute fluctuations might have caused regions of varying density in the nascent universe.
- Influence on Cosmic Microwave Background: Quantum fluctuations are thought to contribute to the anisotropies in the Cosmic Microwave Background Radiation, a snapshot of the early universe.
By analyzing quantum fluctuations, cosmologists can also address fundamental questions about the universe’s symmetry and geometry during the Big Bang.
Implications for Pre-Big Bang Conditions
The implications of quantum mechanics extend beyond mere fluctuations; they can reshape our understanding of the universe before the Big Bang. The notion that physical laws may behave differently under extreme conditions opens new avenues for theoretical exploration and debate.
Considerations include:
- Singularity Proposal: At the point of the Big Bang, classical physics suggests a singularity where conventional laws fail. Quantum mechanics may provide alternate explanations for such states.
- Theoretical Models: Various models, such as loop quantum gravity, propose that the universe may have undergone cycles of expansion and contraction, rather than emerging from an absolute void.
- Existence of Multiple Universes: Some interpretations argue for a multiverse scenario, where our universe is one of many, each arising from different quantum fluctuations.
"Quantum mechanics not only explains the smallest units of matter but also offers insights into the very fabric of the universe itself."
Cosmic Inflation Theory
Cosmic inflation theory serves as a cornerstone for understanding the early universe. It proposes a rapid expansion that occurred just after the Big Bang, stretching the fabric of space-time. This idea helps explain several large-scale structures we observe in the universe today. Understanding this theory is essential in discussing the potential mechanisms that contributed to the Big Bang. Additionally, it provides a framework for evaluating the uniformity and isotropy of the universe as we perceive it now.
The Concept of Inflation
The core idea of inflation suggests that the universe underwent an exponential growth phase. This event is believed to have taken place mere fractions of a second after the Big Bang. During this inflationary period, the universe expanded immensely, driven by high-energy fields.
The implication is powerful: if inflation occurred, it could explain the uniform temperature of the cosmic microwave background radiation. Earlier theories could not sufficiently account for this fascinating homogeneity observed today. Inflation also postulates about the creation of galaxies, as quantum fluctuations during this rapid expansion led to density differences. These variations eventually coalesced, forming the large structures we observe in contemporary astronomy.
Testing the Inflationary Model
Testing the inflationary model involves both observational and theoretical approaches. Scientists use advanced techniques in cosmology to gather data supporting this theory. Observations of the cosmic microwave background radiation yield valuable insights into the early universe's conditions. Tools like the Planck satellite have played a significant role in this regard.
Moreover, researchers look for signatures of inflation in the large-scale structure of the universe. For instance, the distribution of galaxies can provide clues that align with inflation predictions. However, challenges remain in gathering concrete evidence. While many aspects of inflation are supported by data, the model is still a work in progress involving continuous scrutiny and refinement.
Consequences of Inflation on Universe Structure
The inflationary model has significant consequences for understanding the universe's structure. First, it helps to explain the large-scale homogeneity and isotropy we observe. This uniformity can be traced back to the inflationary period, which effectively smoothed out irregularities.
Furthermore, inflation leads to the idea of eternal inflation, where different regions of space continue to inflate at various rates. This concept introduces the notion of multiple universes, or the multiverse theory. Although still largely theoretical, the idea suggests an incredibly complex structure to reality itself.
In summary, cosmic inflation theory not only enhances our comprehension of the early universe but also presents many questions that researchers continue to explore. The ramifications of this theory stretch far beyond initial expansion, influencing the way we perceive the entire cosmos.
The Role of Fundamental Forces
Understanding the role of fundamental forces is crucial for grasping the dynamics of cosmic evolution following the Big Bang. These forces uniquely shape the interactions between matter and energy, profoundly influencing the formation and structure of the universe. By examining these forces, we can better appreciate how they govern cosmic phenomena and lead to the reality we observe today.
The Four Fundamental Forces
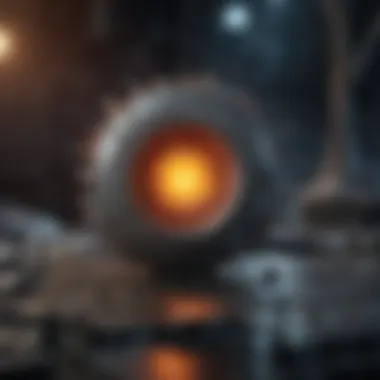
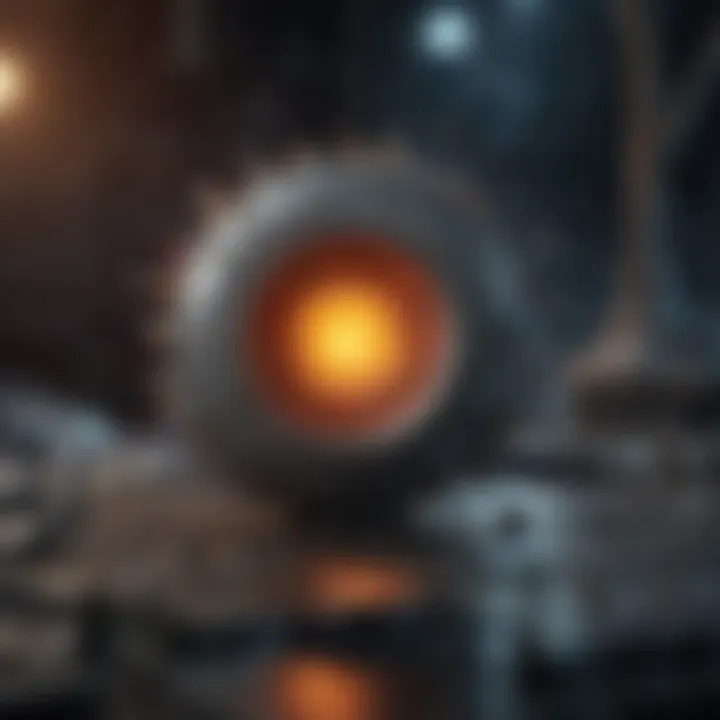
The universe operates according to four fundamental forces: gravitational, electromagnetic, weak nuclear, and strong nuclear forces. Each force plays distinct roles in the cosmic narrative.
- Gravitational Force: This is the force that attracts matter together, giving rise to the formation of galaxies, stars, and planets. It is the weakest force but operates over vast distances, shaping large-scale structures in the universe.
- Electromagnetic Force: This force governs the interactions between charged particles and photons. It's responsible for the formation of atoms and molecules, thus playing a fundamental role in chemistry and the behavior of matter.
- Weak Nuclear Force: This force is vital for processes like nuclear decay and fusion in stars. It facilitates reactions that power the sun, affecting the life cycle of stars.
- Strong Nuclear Force: This is the strongest of the four forces, binding protons and neutrons within atomic nuclei. Its existence is crucial for the stability of matter at a fundamental level.
How Forces Govern Cosmic Development
The interplay between these fundamental forces has been essential in enabling the universe to evolve from a hot, dense state to its current structure. For instance, gravitational force plays a key role in clumping matter together, leading to the formation of galaxies. Electromagnetic forces allow atoms to bond, creating the matter that composes stars and planets.
"The interplay of these forces establishes the framework within which the universe organizes itself, from the smallest subatomic particles to the largest structures known."
Likewise, the strong nuclear force holds atomic nuclei together. Without it, atoms could not exist, and consequently, neither could any form of matter. The weak nuclear force allows for hydrogen to fuse into helium within stars, powering them and facilitating the nucleosynthesis of heavier elements.
Through this lens, we see that the four fundamental forces are not merely background elements in the cosmic story; they are active agents of change, shaping the fundamental tapestry of reality. An exploration into these forces reveals not only how the universe behaves on a grand scale but also how the very fabric of existence is woven together.
Evidence Supporting the Big Bang
Understanding the evidence that supports the Big Bang theory is essential for grasping cosmological concepts. This theory offers an explanation for the origin and evolution of the universe. The importance of this topic lies in its implications for our knowledge of cosmic history. By examining various forms of evidence, we can validate the theory and appreciate its significance in the broader context of astrophysics.
Cosmic Microwave Background Radiation
Cosmic Microwave Background Radiation (CMB) serves as a crucial cornerstone for the Big Bang theory. Discovered in the 1960s, this faint afterglow of the early universe provides a snapshot of the universe when it was merely 380,000 years old. At this stage, the universe had cooled enough for electrons and protons to combine and form neutral hydrogen. The CMB is nearly uniform in all directions, suggesting that the universe was once in a hot, dense state before expanding.
The significance of CMB lies in its temperature fluctuations, which represent regions of density. These fluctuations are precursors to the galaxy formations we see today. Scientists like George Gamow proposed models predicting the existence of such radiation. The later work by Arno Penzias and Robert Wilson confirmed this, earning them a Nobel Prize in Physics. The presence of CMB reaffirms the Big Bang theory’s timeline and validates its predictions.
Redshift Observations
Redshift observations play a vital role in demonstrating the expansion of the universe, a key feature of the Big Bang model. Edwin Hubble first observed that distant galaxies are moving away from us. This movement causes the light from these galaxies to shift toward the red end of the spectrum, hence the term ‘redshift.’ The greater the distance, the faster the galaxy is receding. This correlation is captured succinctly in Hubble's Law: ( v = H_0 d ), where ( v ) is the velocity of the galaxy, ( H_0 ) is the Hubble constant, and ( d ) is the distance.
Redshift observations have pivotal implications. They imply that the universe has been expanding since its inception. This observation argues against a static universe model and instead favors the dynamic nature of cosmic evolution as described by the Big Bang theory. It provides a mechanism for understanding how space itself expands, leading to the current structure of the cosmos.
Abundance of Light Elements
The abundance of light elements further supports the Big Bang theory. According to the model, during the early moments of the universe, nuclear reactions occurred, forming hydrogen, helium, and lithium in specific ratios. Observational data from astrophysical studies indicate that the primordial elements align with theoretical predictions derived from the Big Bang nucleosynthesis.
Hydrogen accounts for about 75% of the normal matter in the universe, with helium making up about 25%. Lithium remains as trace material. These proportions present strong evidence that the universe underwent a hot, dense phase that allowed these reactions to take place. The consistency of these elemental abundances between observed cosmic structures and theoretical expectations strongly validates the framework provided by the Big Bang theory.
"The evidence presented not only supports the Big Bang theory but reshapes our comprehension of the universe's evolution and structure."
Philosophical Considerations
Philosophical considerations play a crucial role in the discourse surrounding the Big Bang theory. This segment goes beyond mere scientific inquiry; it touches on our core understanding of existence and reality. As we investigate the origins of the universe, we encounter fundamental questions that challenge our perceptions of time, space, and what it means to exist. These inquiries are essential, as they bridge the gap between scientific understanding and deeper philosophical reflection.
The Quest for Origins
The quest for origins is a timeless endeavor. Humans have always sought answers to questions about our beginnings. The Big Bang theory provides a scientific framework for these inquiries, suggesting that our universe had a specific origin point. This is significant because it implies that everything we observe today arose from an event roughly 13.8 billion years ago.
Many thinkers argue that understanding the origins of the universe is tied to understanding our own existence. By exploring the circumstances that led to the Big Bang, we essentially explore what it means to be part of a universe governed by physical laws. This exploration raises profound questions:
- What existed before the Big Bang?
- Can we consider the universe to be infinite or finite?
- How do concepts of time and change emerge?
Such queries take us beyond the scientific realm and enter philosophical territory.
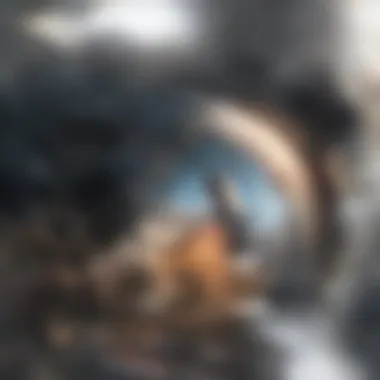
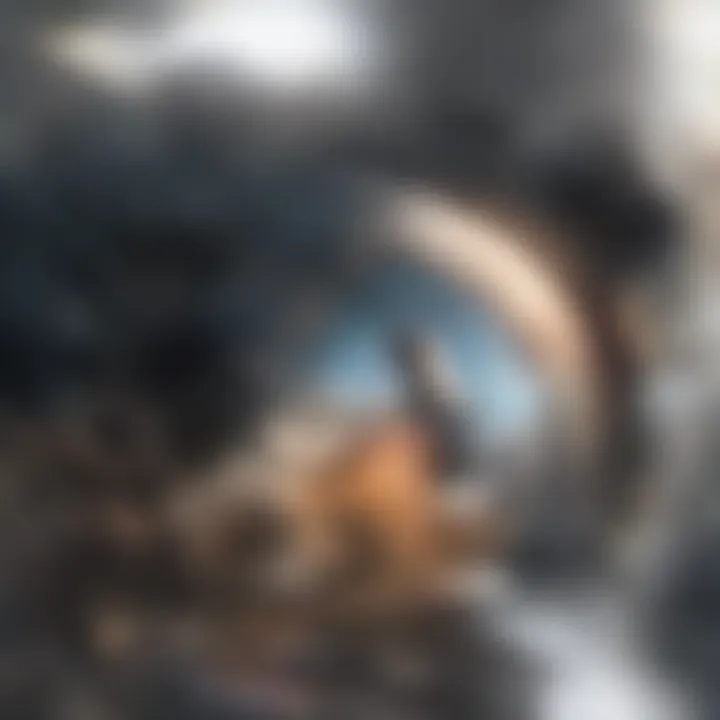
Implications for Our Understanding of Existence
Exploring the Big Bang has significant implications for our understanding of existence. The theory does not just describe physical phenomena; it challenges our very notions of reality. For instance, if the universe began from a singularity, one must consider whether time itself began at that moment.
Moreover, the framework of the Big Bang accommodates both scientific and philosophical discourse concerning creation and causality. It raises essential points on concepts of beginnings and endpoints. Researchers and philosophers alike delve into:
- The nature of causality in the universe
- How the universe's expansion affects our understanding of existence
- The implications of possible multiverses on our perception of reality
By examining these elements thoroughly, we can grasp the complexity of existence itself. The discussion reinforces the notion that scientific and philosophical pursuits are not necessarily separate; in fact, they can enhance each other profoundly.
"The quest for understanding our origins is as much a philosophical inquiry as it is a scientific one. Only through both lenses can we grasp the depths of existence."
Challenges to the Big Bang Theory
The Big Bang theory, while widely accepted as the leading explanation for the origins of the universe, faces several challenges that provoke thought and discussion among scientists and enthusiasts alike. Understanding these challenges is crucial for several reasons. First, they prompt deeper inquiry into the very fabric of cosmology and push the boundaries of human knowledge. Second, they highlight the scientific method's dynamic nature; knowledge evolves as new evidence and interpretations emerge. Lastly, these challenges foster discussions surrounding the fundamental questions of existence, the universe's beginning, and its eventual fate.
Alternative Cosmological Models
In response to the Big Bang theory, several alternative cosmological models have been proposed. These models attempt to explain the universe’s origins without relying solely on the traditional framework of the Big Bang. Notably, the Steady State Theory posits that the universe is eternal and unchanging, with matter being continuously created to maintain a constant density as it expands. This idea was once prominent but has since lost favor due to the accruing evidence supporting the Big Bang.
Another notable model is the Cyclic Universe model, which suggests that the universe undergoes infinite cycles of expansion and contraction. According to this theory, our universe is currently in a phase of expansion but will eventually contract before rebirthing anew. This concept challenges the notion of a singular beginning, raising profound implications about time and existence.
While each alternative model presents unique insights, they often face significant hurdles. Evidence such as cosmic microwave background radiation continues to lend support to the Big Bang theory. Still, ongoing research in cosmology means that these alternatives deserve attention for their potential to refine our understanding of the universe.
Addressing Anomalies and Gaps
Throughout the years, several anomalies have arisen that challenge the robustness of the Big Bang framework. One prominent issue is the Horizon Problem, which questions why distant regions of the universe, separated by vast distances, exhibit such remarkably uniform temperatures. This uniformity seems to contradict the Big Bang explanation of localized heat fluctuations.
Another area of concern involves the apparent flatness of the universe. Observations indicate that the universe is remarkably flat, which raises questions about the initial conditions required for such a state to occur. In contrast, the Big Bang theory would predict a curved structure under certain conditions.
Lastly, the observed distribution of galaxies and cosmic structures presents a puzzle. The large-scale structure of the universe shows patterns that some argue cannot be fully explained by the Big Bang alone. These discrepancies have led scientists to search for solutions, including the proposal of dark matter and dark energy, which may provide alternative explanations for these observations.
As we venture further into exploring these anomalies and their implications, ongoing research may reveal more about the universe's complexities and possibly lead to a more refined cosmological model that addresses both traditional and emerging challenges.
Current Research and Future Directions
The field of cosmology is ever-evolving, particularly in the context of understanding the Big Bang. Current research plays a crucial role in refining or challenging existing models that seek to explain the origins and development of the universe. The importance of diving into contemporary findings cannot be understated; these discoveries illuminate paths for amendments or expansions of established theories. Moreover, they fuel our quest for knowledge about fundamental principles of physics and deeply explore the very fabric of reality itself.
Recent Discoveries in Cosmology
Recent advancements in cosmology have been significant and indicate a shift towards a more unified understanding of our universe. For instance, the detection of gravitational waves has created new opportunities to study cosmic events in ways previously unimaginable. These waves provide direct evidence of cataclysmic events such as black hole mergers, affirming concepts of relativity and offering glimpses into the state of the universe shortly after the Big Bang.
Additionally, observations from telescopes like the James Webb Space Telescope have enhanced our ability to peer into the early universe. Findings from these observations include:
- Confirmation of earlier galaxies: Discoveries of ancient galaxies challenge previous models regarding the rate of star formation in the early universe.
- Detailed analysis of cosmic microwave background radiation: Continued studies have revealed subtle fluctuations that may provide insights into inflationary models.
- Deep learning applications: Utilizing AI to understand vast datasets has birthed new possibilities for identifying and analyzing cosmic phenomena.
These trends show not only the excitement of new scientific revelations but also highlight how inter-disciplinary approaches can lead to breakthroughs in understanding complex cosmic processes.
Potential Areas for Further Investigation
Despite the progress made, several areas still require meticulous exploration to unravel lingering mysteries surrounding the Big Bang and its impacts. Potential avenues for future research include:
- Understanding Dark Energy: The role dark energy plays in the universe's expansion remains poorly defined. Investigating its properties and effects could reshape our understanding of cosmic evolution.
- Quantum Gravity: Integrating general relativity with quantum mechanics remains a critical challenge. Research in this area could lead to better insights into the conditions of the early universe.
- Alternative Theories of Cosmic Evolution: Considering hypotheses that oppose the standard model, such as cyclic models or modifications to Einstein’s theory, may yield new perspectives on cosmic origins.
- Effect of Black Holes: The impact of black holes on surrounding space and time continues to be a fascinating subject. Investigating their influences could deepen our comprehension of spacetime dynamics.
- Observation Techniques: Continuous improvement in observation technology presents opportunities for enhanced empirical data collection, allowing more accurate and far-reaching studies in cosmology.
As research progresses, the implications of these inquiries are profound. They push the boundaries of what we know and encourage collaboration among different branches of science.
"To understand the universe, we must first understand ourselves and our place within it."