The Lasting Influence of Einstein's Discoveries
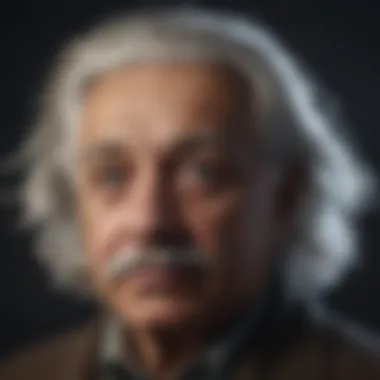
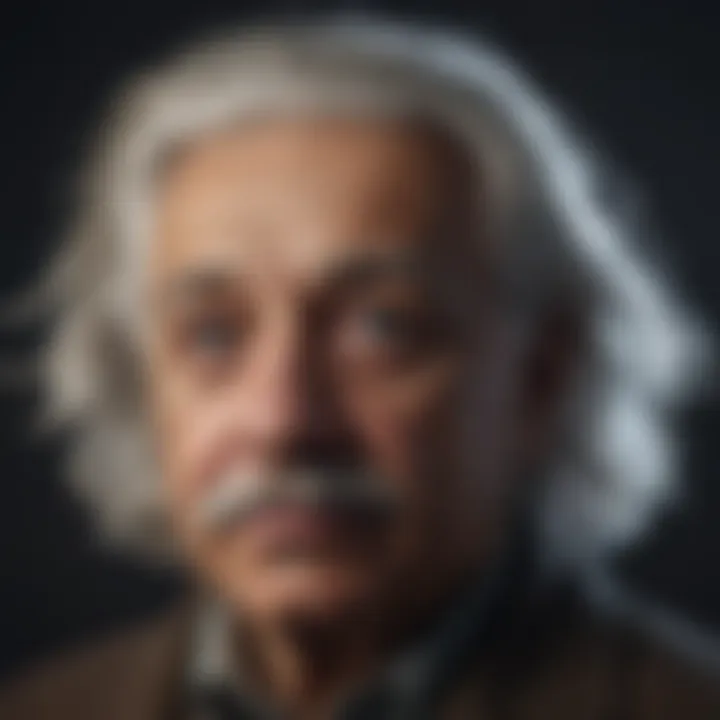
Intro
Albert Einstein's contributions to physics resonate well beyond his lifetime. His work forms the backbone of modern scientific thought. In this article, we will dissect his pivotal discoveries and examine their implications on our understanding of the universe and society at large.
Einstein's theories first emerged during a time of significant progress in physics. His revolutionary findings challenged established norms and encouraged a new way of thinking. From relativity to quantum physics, his insights laid the foundations for advances in various scientific fields. This exploration aims to provide clarity on how his work continues to influence ongoing research and technological advancements in contemporary society.
Key Research Findings
Overview of Recent Discoveries
Recent explorations into Einstein's work have unveiled further implications of his theories. For instance, the detection of gravitational waves has provided evidence for his general theory of relativity. This discovery adds a significant layer to our understanding of astrophysical phenomena. Additionally, the photoelectric effect, initially described in Einstein's 1905 paper, has led to advancements in solar energy technologies.
Significance of Findings in the Field
Einstein's theories have become a cornerstone in modern physics. His assertion that mass and energy are interchangeable changed the way scientists view matter. The equation E=mc^2 is pivotal in areas such as nuclear energy and particle physics. Furthermore, his work on quantum mechanics, though initially controversial, has gained acceptance, influencing a range of technologies including semiconductors and quantum computing.
"Knowledge of what is possible is the beginning of all wisdom."
Breakdown of Complex Concepts
Simplification of Advanced Theories
Complex theories, such as general relativity, often seem inaccessible. However, breaking them down into simpler concepts can aid comprehension. For example, understanding gravity not as a force but as the curvature of spacetime makes the abstract concept more tangible. This approach can foster a better grasp of the underlying principles that Einstein articulated.
Visual Aids and Infographics
Visual aids can enhance understanding of intricate theories. Diagrams illustrating spacetime curvature or energy-mass equivalence can simplify complex ideas. Infographics can effectively communicate the implications of Einstein's discoveries, making them more accessible.
Preface to Albert Einstein
Albert Einstein's work stands as a cornerstone of modern physics. His theories not only reframed our understanding of the universe but also challenged prevailing notions within science. Delving into Einstein’s contributions provides a vital context for understanding many contemporary scientific principles.
In this section, we will explore two fundamental aspects of Einstein’s legacy: his biographical details and the scientific environment he navigated prior to making his groundbreaking discoveries. Knowing the man behind the theories adds depth to the discussion.
Additionally, comprehending the state of scientific knowledge before Einstein's time highlights the dramatic shifts his work initiated. Studying the backdrop of existing theories makes it easier to appreciate the brilliance and impact of Einstein’s contributions as they reshaped basic principles in physics.
This exploration is beneficial not only for students and researchers but also serves educators and professionals as it offers insights into how Einstein's innovations resonate within their fields today.
Biographical Overview
Albert Einstein was born on March 14, 1879, in Ulm, Germany. His family later moved to Munich, where he began his education. From early on, he displayed an affinity for mathematics and physics, although traditional education was not always a favorable experience for him.
At seventeen, Einstein enrolled in the Polytechnic Institute in Zurich. Here, he studied various subjects, laying the foundation for his future work. After graduation, he struggled to find a position, eventually securing a job at the Swiss Patent Office in Bern. This position afforded him time to ponder theoretical physics, leading to the development of some of his most significant concepts.
In 1905, a year often referred to as Einstein's annus mirabilis or miraculous year, he published four groundbreaking papers. They included the special theory of relativity and the photoelectric effect, both of which would have profound implications in physics and beyond.
Einstein's life was not only defined by his scientific achievements. He became an iconic figure, advocating for pacifism, global disarmament, and civil rights. His migration to the United States in 1933, amid the rise of the Nazi regime, marked a new chapter in his life. He held a position at Princeton University until his passing in 1955, cementing his role as a crucial influencer in both science and society.
The Scientific Landscape Before Einstein
Prior to Einstein's breakthroughs, the scientific world was under the influence of Newtonian mechanics, which effectively described many physical phenomena. Sir Isaac Newton's laws of motion and universal gravitation dominated. However, there were inconsistencies, especially when dealing with light and electromagnetism.
Maxwell's equations described electromagnetic waves and light comprehensively but did not align neatly with Newtonian physics. This discrepancy created a tension within the scientific community. While scientists were beginning to embrace concepts such as the ether theory, the need for a cohesive framework for understanding the relationship between energy and mass was becoming apparent.
In this context, physicists were trying to reconcile these principles. Concepts such as Lorentz transformations were being explored, hinting at the idea of relative motion. Einstein's perspective would come to reframe these discussions entirely.
Ultimately, understanding the conditions that existed prior to Einstein’s theories allows us to appreciate the magnitude of his insights and the marvellous ways in which he revolutionized the field of physics.
The Special Theory of Relativity
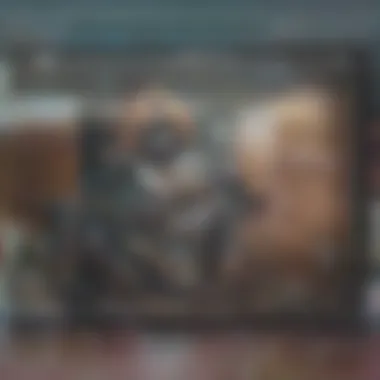
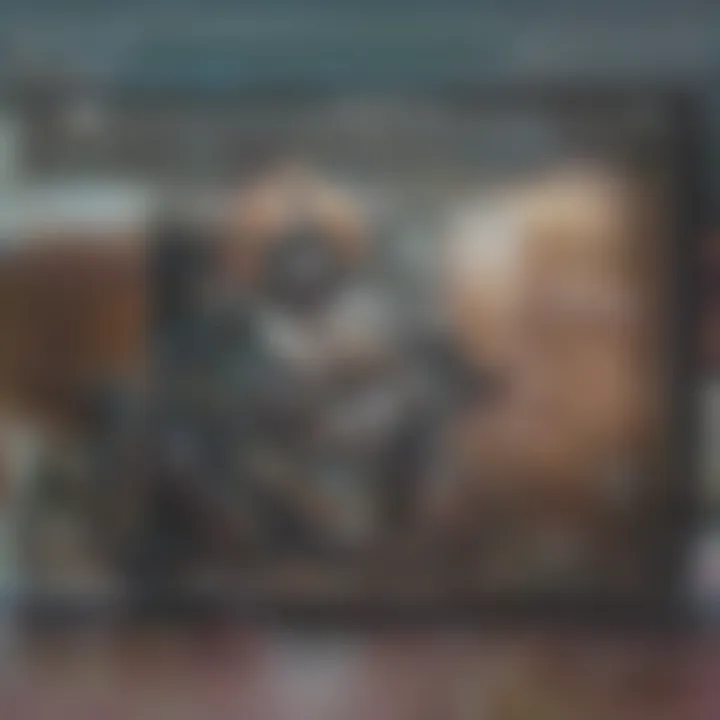
The Special Theory of Relativity, which was published by Albert Einstein in 1905, represents a cornerstone in modern physics. Its principles challenge and redefine conventional notions of space and time. This theory posits that the laws of physics are the same for all observers in uniform motion relative to one another. This idea is not merely abstract; it carries profound ramifications for how we understand the universe.
When we delve into the Special Theory of Relativity, we begin to see how it enhances our understanding of fundamental physical phenomena. Important elements include the concepts of time dilation and space contraction, which will be analyzed in the sections below. The theory fundamentally alters our perception of two crucial factors: the behavior of objects moving at high velocities and the interdependence of space and time, leading to the unification of the two into a single four-dimensional continuum known as spacetime.
Concept of Time Dilation
Time dilation is one of the more intriguing concepts derived from the Special Theory of Relativity. It suggests that time is not an absolute measurement. Instead, it varies according to the relative speeds of observers. When an object moves at a significant fraction of the speed of light, time for that object appears to pass slower compared to an observer at rest.
This phenomenon has been empirically validated through various experiments, including those involving atomic clocks on aircraft or satellites, which have consistently shown discrepancies when compared to stationary clocks on the ground. The implications of time dilation affect both theoretical physics and practical applications, such as the operation of the Global Positioning System (GPS), where satellite clocks need to adjust for differences in the passage of time.
"Time dilation has significant implications, especially in technology reliant on precise timekeeping."
Space Contraction
Space contraction, also known as length contraction, is another critical component of Einstein’s theory. It states that an object’s length is measured to be shorter when it is moving at speeds close to the speed of light relative to an observer. This effect becomes pronounced only at extreme velocities that are not commonly encountered in everyday life.
However, it significantly alters how we conceptualize distances in the universe. As one approaches the speed of light, the length of the travel path manifests differently than within traditional Newtonian physics. Such nuances invite a reevaluation of how we understand motion and distance in the cosmos.
Consequences of Special Relativity
The consequences of the Special Theory of Relativity extend far beyond academic curiosity. They invite a new perspective on reality and have practical applications across many facets of modern life. Important outcomes of the theory include the formulation of the mass-energy equivalence principle, encapsulated in the famous equation E=mc², which revolutionized our understanding of energy and mass.
In addition, relativity has implications in fields such as particle physics and cosmology. Technologies such as particle accelerators and theories regarding the behavior of black holes rely on the tenets established by Einstein. With each revelation related to the Special Theory of Relativity, we are reminded of the remarkable shift in intellectual thought that Einstein’s work catalyzed.
The General Theory of Relativity
The General Theory of Relativity is one of Albert Einstein’s most significant contributions to physics. This theory advanced scientists' comprehension of gravity and changed the paradigms within physics. Through this theory, Einstein not only reformulated the understanding of gravity but also introduced revolutionary concepts that have deep implications in both theoretical and observational astrophysics.
Principles of Equivalence
Einstein introduced the concept of the equivalence principle, which posits that gravitational force experienced by an object in a gravitational field is indistinguishable from the forces experienced by an object undergoing acceleration. This idea laid the groundwork for understanding how gravity operates not merely as a force, but as a curvature of space and time itself, fundamentally altering previous Newtonian views. The implications of this principle extend far beyond theoretical discussions, applying to real-world phenomena through satellite technology and GPS systems that require precise calculations of time and motion.
Gravitational Time Dilation
Gravitational time dilation is a striking consequence of the general relativity theory. It describes how time is affected by gravity, illustrating that time passes differently in stronger gravitational fields compared to weaker ones. For example, clocks closer to a massive object, like Earth, tick slower than those further away. This phenomenon has been experimentally verified and shows how gravity influences the flow of time itself. Understanding this effect is crucial for technologies that rely on synchronizing time, such as global positioning systems, allowing them to correct for time discrepancies caused by different gravitational fields.
Curvature of Space-Time
At the core of the General Theory of Relativity is the idea that gravity is not merely a force but a curvature of space-time created by mass. Massive objects like planets and stars deform the fabric of space-time around them, causing the paths of other objects to bend when they move through this region. This concept radically reshaped the understanding of cosmic phenomena. It provided explanations for the orbits of planets and the motion of light around massive bodies, which has been observed during solar eclipses, confirming Einstein’s predictions.
Observational Proofs
The General Theory of Relativity has withstood over a century of rigorous testing and scrutiny. Numerous observational proofs validate its concepts. The bending of light by gravity, observed during the solar eclipse in 1919, was one of the first confirmations that stunningly displayed the reality of Einstein's theories. Further validations include the precise measurement of gravitational waves, detected by LIGO, and the phenomena of black holes. These experiments support the theory's predictions and demonstrate its profound impact on modern astrophysics.
"No amount of experimentation can ever prove me right; a single experiment can prove me wrong."
— Albert Einstein
Mass-Energy Equivalence
Mass-energy equivalence is a fundamental principle of physics, originally captured in the equation E=mc². This formula illustrates a profound insight into the nature of mass and energy, demonstrating that they are interchangeable forms of the same entity. Albert Einstein first introduced this concept in 1905 as a part of his special theory of relativity, marking a pivotal moment in the understanding of physical principles.
The significance of mass-energy equivalence extends beyond theoretical physics. It provides the groundwork for understanding various phenomena, ranging from nuclear reactions to the mechanics of stars. In layman’s terms, it tells us that mass can be converted into energy and vice versa, which has far-reaching implications in both scientific theory and practical applications.
Moreover, mass-energy equivalence challenges our conventional notions of matter and energy. It revises the classical understanding of rest mass and kinetic energy, leading to new predictions about how particles behave at high velocities. Consequently, this principle has become a cornerstone of modern physics, deeply influencing fields such as particle physics and cosmology.
"Mass and energy are not separate entities; they are intertwined aspects of a unified reality, shifting our perception in profound ways."
Understanding E=mc²
The equation E=mc² is perhaps one of the most famous formulas in physics. In this equation:
- E represents energy.
- m denotes mass.
- c refers to the speed of light in a vacuum (approximately 299,792,458 meters per second).
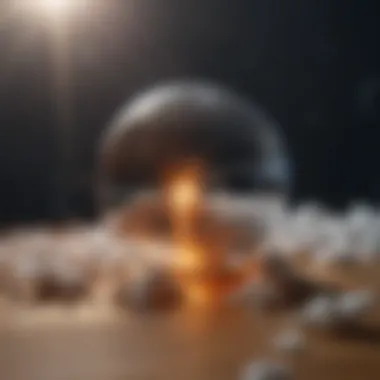
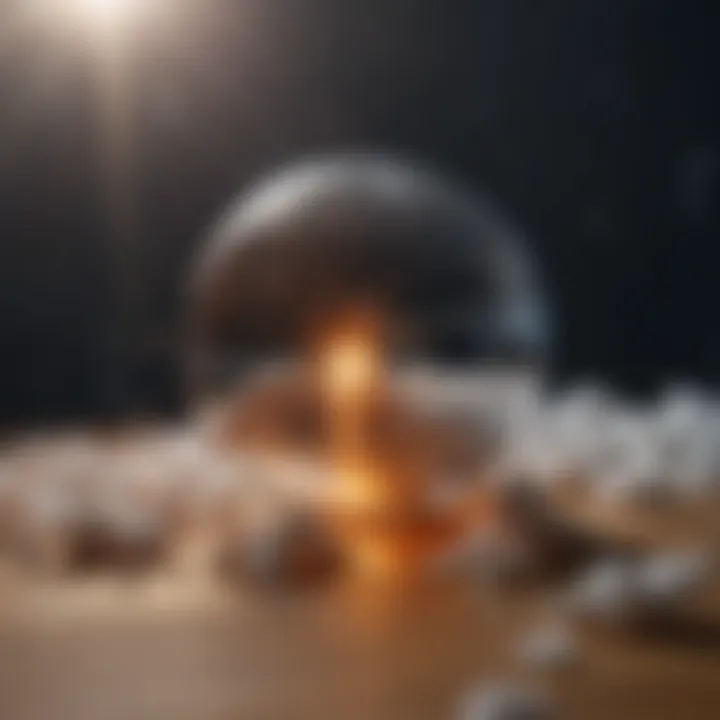
This equation indicates that a small amount of mass can be converted into a large amount of energy, due to the square of the speed of light being a very large number. For example, in nuclear fission, only a tiny fraction of the mass of the atomic nucleus is converted into energy, yet this results in vast amounts of energy being released. Thus, E=mc² is not only a theoretical statement but has actual real-world applicability, particularly in the understanding of atomic and subatomic particles.
Furthermore, the implications of mass-energy equivalence ripple through many aspects of physics, offering insights into the interplay between gravity and energy in the fabric of the universe.
Applications in Nuclear Physics
In nuclear physics, the principle of mass-energy equivalence is central to explaining various processes that release energy. One prominent example is nuclear fission, which occurs in reactors such as the ones found in nuclear power plants. Here, the nucleus of a heavy atom, like Uranium-235, splits into smaller nuclei, thereby releasing energy due to the mass loss during the reaction.
Another application is in nuclear fusion, the process that powers the sun and other stars. In fusion, light nuclei combine to form a heavier nucleus, which also results in a substantial release of energy. The mass of the resulting nucleus is less than the sum of its components due to the energy that is released, further exemplifying E=mc² in action.
In addition, this principle underlines the development of atomic bombs, where uncontrolled nuclear reactions lead to explosive releases of energy. These applications highlight not only the potency of mass-energy equivalence but also the ethical and safety considerations that surround its use in technology and warfare.
The Photoelectric Effect
The photoelectric effect is a fundamental phenomenon in physics that has significant implications for our understanding of light and matter. This concept is crucial as it was pivotal in the development of quantum mechanics and showcases how light can behave both as a wave and a particle. Albert Einstein's work on the photoelectric effect brought a new level of understanding to the relationship between energy and light, emphasizing its role in explaining various physical processes.
Experimental Observations
In investigating the photoelectric effect, Einstein built upon previous experiments, notably those by Heinrich Hertz. Hertz discovered that ultraviolet light could cause sparks to jump between two metal electrodes. However, he lacked a comprehensive explanation for this occurrence. Einstein proposed that light consists of discrete packets of energy, called photons.
Experiments demonstrated that when light shines on a metal surface, electrons are ejected if the light frequency exceeds a certain threshold. This threshold frequency varies among different metals. Notably, increasing the intensity of light does not lead to ejection of electrons unless the frequency is above the threshold. This observation contradicts classical wave theory, which would argue that higher intensity should result in more energetic waves.
Quantization of Light
Einstein's theoretical approach posited that light does not merely behave as a wave but consists of quantized energy packets. He discussed how the energy of each photon is proportional to its frequency, articulated mathematically as:
[ E = hf ]
Where ( E ) is the energy, ( h ) is Planck's constant, and ( f ) is the frequency of the light. This quantization reveals why only light of certain frequencies can dislodge electrons from a material. Moreover, this understanding is the cornerstone of photonics and has led to advancements in various technologies, including solar cells and photodetectors.
Implications for Quantum Mechanics
The implications of the photoelectric effect extend beyond classical physics, ultimately shaping the framework of quantum mechanics. Einstein's explanation validated the concept of quantized energy levels, laying the groundwork for later developments by physicists such as Niels Bohr and Louis de Broglie.
A major takeaway from this phenomenon is the dual nature of light. It behaves both as a continuous wave and as a stream of particles. This duality is not merely an academic curiosity; it serves as a foundation for many modern technologies, such as laser medicine and the functioning of everyday electronic devices.
"Albert Einstein's insights into the photoelectric effect catalyzed a shift in perspective, moving physics toward a more integrated approach incorporating both particle and wave ideas."
In summary, the photoelectric effect forged critical links in our grasp of quantum phenomena. Understanding this effect has not only historical significance but also plays a vital role in contemporary scientific research, emphasizing Einstein's lasting influence on modern physics.
Brownian Motion
Brownian motion is a fundamental concept in the realm of physics, particularly in the context of statistical mechanics and thermodynamics. This phenomenon describes the erratic and random movement of microscopic particles suspended in a fluid. Albert Einstein's work on Brownian motion provided substantial evidence for the existence of atoms and molecules, playing a crucial role in the acceptance of atomic theory in the scientific community.
Observing Microscopic Particles
When Einstein studied Brownian motion, he aimed to explain the behavior of tiny particles, such as pollen grains, as they moved through water. His calculations demonstrated how these particles were bombarded by the surrounding fluid molecules. This movement is not merely random; it follows a statistical distribution over time. By observing these microscopic particles, scientists were able to gather more empirical data on their motion and interactions with the fluid medium. This was groundbreaking because it presented a tangible way to witness the effects of atomic and molecular activity.
Einstein's theoretical insights allowed researchers to link the observable motion of particles to the statistical mechanics underlining their behaviors. Notably, his equations quantitatively predicted the mean squared displacement of particles over time. This predictive ability was significant in validating atomic theory, as it provided clear, observable phenomena directly tied to the existence of atoms.
Confirmation of Atomic Theory
The implications of Einstein's work on Brownian motion extend far beyond the microscopic level. His explanations formed a pivotal component in confirming atomic theory, which posits that matter is composed of discrete units. Prior to Einstein, the existence of atoms was still a subject of debate. By providing a mathematical framework that connected the movement of particles to the molecular structure of matter, Einstein furnished empirical support for the idea that atoms and molecules constitute the foundation of physical substances.
The experimental validation of Brownian motion not only boosted the credibility of atomic theory but also led to the development of further research in statistical physics and chemistry. The understanding of Brownian motion laid the groundwork for many modern scientific explorations, ranging from the study of chemical reactions to the examination of biological processes at the cellular level.
"Einstein's exploration of Brownian motion gave physicists tangible evidence of the atomic structure of matter, establishing a key link between theoretical physics and experimental observation."
Influence on Cosmology
Albert Einstein’s discoveries fundamentally shaped the field of cosmology. Before his theories, the understanding of the universe was largely speculative and influenced by philosophical considerations more than scientific inquiry. Einstein redirected cosmology toward a more mathematical and empirical approach. His work instilled a new sense of rigor and precision in the study of the cosmos, making a lasting impact that continues to resonate in modern science.
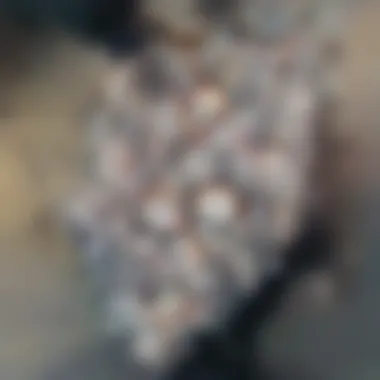
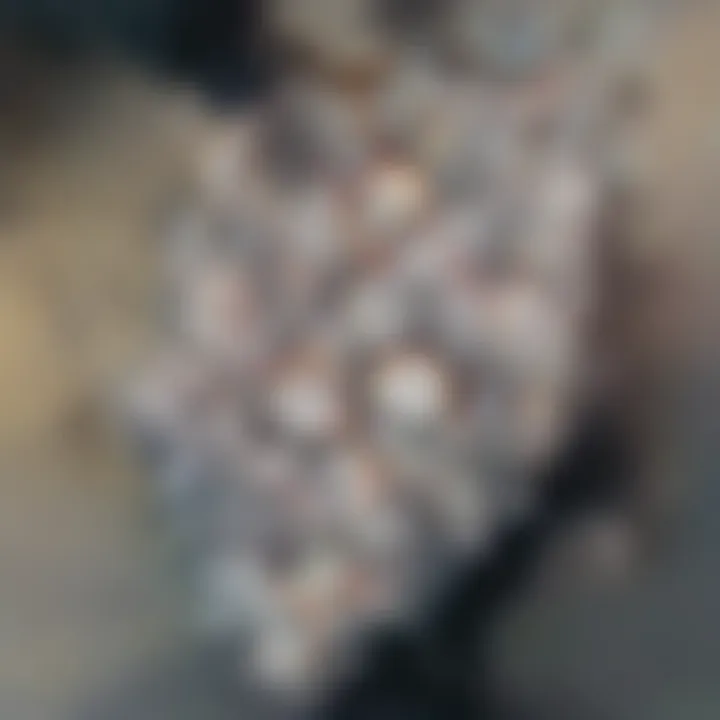
One of the most significant contributions stemming from Einstein's theories is the Field Equations of General Relativity. These equations provided a new framework for understanding how matter and energy influence the fabric of space-time. Before Einstein, the gravitational model posited by Isaac Newton was the predominant view. Einstein's radical approach transformed this perspective by demonstrating that gravity is not merely a force but an effect of curving space-time due to mass.
This conceptual shift laid the groundwork for various cosmological advancements, including the study of black holes and the dynamics of galaxies within the universe. The recognition that mass influences the geometry of space permeates our comprehension of the universe’s structure and behavior.
Further, his work on cosmology prompted scientific inquiry into the universe's nature—with rapid advancements in related fields such as astrophysics and observational astronomy. Consequently, this led to remarkable progress in our comprehension of cosmic phenomena.
Einstein's Field Equations
Einstein's Field Equations are at the core of his General Theory of Relativity. They describe how matter and energy in the universe dictate the curvature of space-time. This set of ten interrelated differential equations replace classical gravitational models with a more holistic understanding of the universe. The equations are expressed as:
In this equation:
- R represents the Ricci curvature tensor, which encapsulates gravitational effects.
- g symbolizes the metric tensor, defining distances and angles in curved space.
- T is the stress-energy tensor, portraying the distribution of matter and energy.
- Λ is the cosmological constant, introduced by Einstein, to allow for static universe solutions.
The implications of these equations extend beyond theoretical physics. They have been instrumental in predicting phenomena like gravitational waves and black holes. The relevance of Einstein’s Field Equations resonates in the realm of cosmology, guiding modern astrophysical investigations and scientific explorations of the universe.
Static Universe vs. Expanding Universe
Before Einstein, the prevailing view of the universe was primarily static, with a belief in an eternal, unchanging cosmos. However, Einstein challenged this notion, particularly through his introduction of the cosmological constant. Initially, his equations suggested that the universe must either contract or expand. To align with the contemporary understanding, he proposed the cosmological constant to support a static model.
This idea persisted until observations by Edwin Hubble revealed that the universe is, in fact, expanding. Hubble's discovery emphasized that galaxies are moving away from one another, an observation that profoundly shifted our understanding of cosmology. The expansion has led to the formulation of multiple cosmological models based on Einstein’s equations.
The acceptance of an expanding universe has numerous implications:
- It suggests that the universe had a beginning, leading to the Big Bang theory.
- This expansion affects our understanding of cosmic background radiation and the formation of large-scale structures in the universe.
- It raises questions about the fate of the universe, impacting theories like dark energy and the ultimate fate of cosmic expansion.
"Einstein's work redefined the universe, opening doors to ideas that were once thought impossible." - Anonymous
For further reading, consider visiting Wikipedia or Britannica for more detailed insights into Einstein’s contributions.
Legacy and Recognition
Albert Einstein's legacy is rich and multifaceted, forming a cornerstone upon which contemporary physics stands. His recognition comes not only from his groundbreaking theories but also from the philosophical shifts he fostered within the scientific community. Einstein's work has had lasting implications on both theoretical understanding and practical applications, influencing fields such as nuclear physics, quantum mechanics, and cosmology. This profound impact emphasizes the necessity of discussing his legacy, illuminating the relevance of his discoveries today.
Nobel Prize in Physics
In 1921, Albert Einstein was awarded the Nobel Prize in Physics for his explanation of the photoelectric effect. Although he is often associated with his theories of relativity, this particular accolade highlighted his contributions to quantum theory. The photoelectric effect demonstrated that light can be understood as both a wave and a particle, which fundamentally challenged existing notions about the nature of light. This recognition marked a pivotal moment in his career, solidifying his reputation in the scientific world. It also helped catalyze further research into quantum mechanics, shaping modern physics.
The award brought Einstein into the limelight. It illustrated how experimental evidence could validate controversial theories, ultimately merging empirical science with abstract ideas. His Nobel Prize win underscored the idea that groundbreaking theories require both theoretical and experimental justifications. The implications of this award contributed to the legitimization of quantum mechanics, influencing a generation of physicists and researchers.
Role in Modern Science
Einstein's role in modern science cannot be overstated. His groundbreaking theories have influenced countless domains beyond physics, including philosophy, technology, and even the arts. His notions of relativity changed the fundamental understanding of space and time, presenting new ways to comprehend the universe. Through his work, he laid the groundwork for advancements in numerous scientific areas, such as GPS technology, which depends on principles of time dilation to function accurately.
Moreover, Einstein's theories fostered the development of concepts like black holes and cosmological models that revolutionized our understanding of the universe's structure and evolution. Scientists today continue to build upon his work, utilizing his ideas to explore the cosmos, from studying the origins of the universe to understanding dark matter and dark energy.
Einstein's impact transcends traditional boundaries of science, prompting interdisciplinary exploration and collaboration.
Closure
The conclusions drawn in this article about Einstein's work are essential for understanding not just his theories, but also their profound significance across multiple fields of science and society. The analysis of Einstein's contributions reveals a rich tapestry of ideas that challenged and revolutionized existing paradigms. His theories, especially the Special Theory of Relativity and the General Theory of Relativity, have reshaped our comprehension of space, time, and gravity.
Enduring Influence of Einstein's Work
Albert Einstein's influence endures within both theoretical physics and applied sciences. His equation E=mc² is not merely a formula; it embodies the relationship between mass and energy that is foundational in nuclear physics and cosmology. Einstein's insights laid the groundwork for various technological advancements, including nuclear energy, and they continue to inspire research in quantum mechanics.
From his early experiments in the photoelectric effect to his later work on cosmic phenomena, his ideas consistently push the boundaries of what is possible. They encourage ongoing inquiries into the universe's most elusive mysteries.
His impact extends into educational spheres, influencing curricula in physics and related fields. Students and educators today still grapple with the implications of his findings, fostering a new generation of critical thinkers.
"A person can be intelligent, but not be wise." - Albert Einstein
The legacy of Einstein manifests in the following ways:
- It cultivates a culture of inquiry and skepticism within scientific communities.
- It encourages interdisciplinary approaches, blending physics with philosophy and the arts.
- It promotes understanding and awareness of scientific principles in public discourse.
In summary, Einstein's discoveries are not just historical footnotes; they are alive in today's scientific discussions and continue to shape our understanding of the universe. As we reflect on his work, it becomes apparent that this legacy is both a challenge and an invitation for further exploration.