A Deep Dive into Particle Physics Discoveries
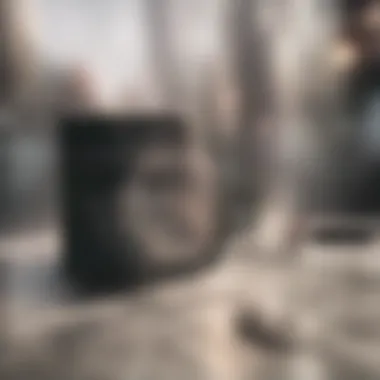
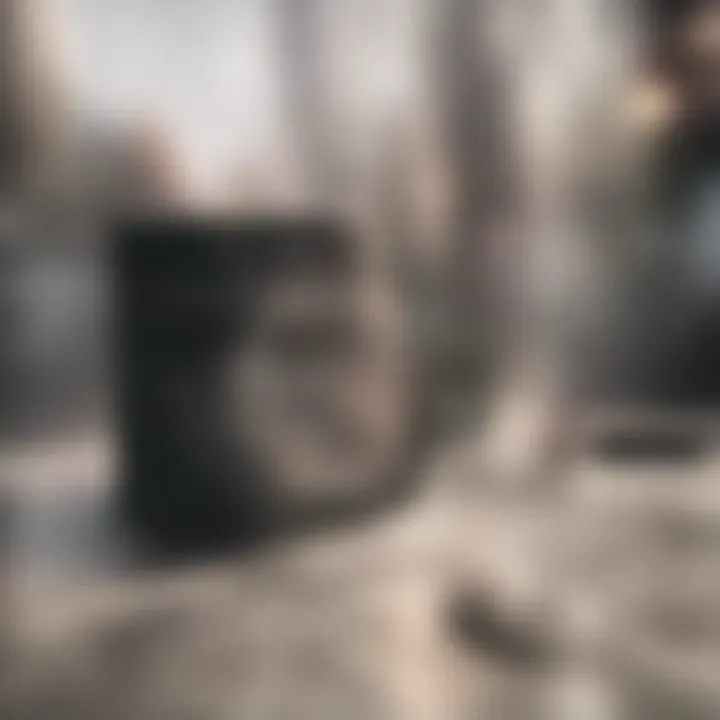
Intro
Particle physics is a branch of physics that studies the smallest constituents of matter and radiation. It seeks to understand the fundamental particles and forces that govern the universe. This field has rich historical roots and has led to revolutionary discoveries about the nature of reality. Understanding particle physics is crucial as it lays the groundwork for multiple scientific domains, from cosmology to material science.
In this article, we will delve into various facets of particle physics. This includes its historical development, formulation of the standard model, analysis of particle interactions, and a look into current technological advancements in experimental methodologies.
A comprehensive approach is necessary for grasping complex topics in particle physics. We aim to present these concepts in a way that is both engaging and accessible, offering insights to students, researchers, educators, and professionals.
Key Research Findings
Overview of Recent Discoveries
Recent discoveries in particle physics have advanced our knowledge significantly. One notable finding is the detection of the Higgs boson at CERNโs Large Hadron Collider in 2012. This particle provides mass to other fundamental particles, confirming a key aspect of the standard model. The Higgs boson discovery not only validated theoretical predictions but also opened new avenues for research.
Another vital development is the discovery of neutrino oscillation, which changes our understanding of these elusive particles. Neutrinos were previously thought to be massless. However, experiments indicate that they possess a small mass, challenging assumptions in particle physics and cosmology.
Additionally, advancements in technology allow for more precise measurements and the potential discovery of new particles. Ongoing experiments aim to identify dark matter candidates and explore physics beyond the standard model, opening pathways to new theories about the nature of the universe and fundamental forces.
Significance of Findings in the Field
The implications of these findings extend beyond theoretical frameworks.
- They enhance our understanding of the fundamental structure of matter.
- Discoveries like the Higgs boson influence the development of future technologies.
- The study of neutrinos contributes to understanding cosmic phenomena and the evolution of the universe.
Overall, ongoing research in particle physics aims to answer monumental questions about existence, the forces that shape our reality, and the very fabric of the universe.
Breakdown of Complex Concepts
Simplification of Advanced Theories
Particle physics is often laden with jargon and complex mathematics. To make these concepts digestible, it is essential to break them down into simpler terms. For instance, the concept of a particle can be explained as a tiny bit of matter or energy. Examples include electrons, quarks, and neutrinos. Recognizing how these particles interact helps connect theory to observable phenomena.
Each particle is governed by fundamental forces, specifically, gravitational, electromagnetic, weak nuclear, and strong nuclear forces. Simplifying these concepts allows a more profound understanding of how they govern the interactions between particles and consequently affect the universe as a whole.
Visual Aids and Infographics
Visual representations can dramatically aid in comprehending particle physics. Infographics displaying the standard model, for instance, illustrate how particles are categorized and the forces they experience. Utilizing such tools in educational settings not only clarifies concepts but also engages students and researchers alike. Hereโs a simple diagram of the standard model:
These diagrams can help visualize the interactions and classification of particles, paving the way for deeper exploration into their roles in the universe.
Intro to Particle Physics
Particle physics is a branch of physics that explores the fundamental constituents of matter and the forces by which they interact. This field is pivotal for understanding the universe at its core. In this section, we explore the definition and scope of particle physics, along with why it holds significant importance in both theoretical and applied sciences.
Definition and Scope
Particle physics studies the smallest building blocks of the universe. This includes basic particles such as quarks and leptons, as well as the interactions that govern their behavior. These interactions are described by the Standard Model, which encapsulates our current understanding of how matter behaves at the atomic and subatomic levels.
The scope of particle physics extends beyond mere identification of particles. It encompasses their properties, behaviors, and interactions under various conditions. This includes a variety of research methods, from collider experiments to cosmic ray observations, to grasp the dynamics of particles.
In essence, particle physics serves as a bridge linking fundamental operations at the micro level to observable phenomena in our macroscopic world.
Importance of Particle Physics
Understanding particle physics is crucial as it lays the groundwork for many scientific disciplines. Here are a few significant reasons that highlight its importance:
- Foundation of Science: Particle physics informs our comprehension of the universe. It provides insights into cosmic events and the fundamental laws governing matter and energy.
- Technological Advances: Innovations in particle physics have led to technology that influences everyday life. Medical technologies, such as PET scans, come from research into particle interactions.
- Philosophical Implications: Studies in this field challenge and expand our understanding of existence, identity, and the behavior of matter and energy.
- Environmental Impact: Research into particle phenomena can lead to improvements in energy production, potentially creating more sustainable technologies.
"Particle physics not only seeks to solve the mysteries of matter but also has profound implications on technology and philosophy."
In summary, the exploration of particle physics is not merely an academic pursuit. It has ramifications across various domains including technology, health, and even our philosophical perspectives on reality. Understanding the principles behind particle physics enriches our grasp of fundamental science and any related advances.
Historical Overview
The historical overview serves as a crucial element in the field of particle physics, as it lays the foundation upon which current theories and experimental practices are built. Understanding the evolution of concepts and ideas helps to clarify how our comprehension of matter has vastly changed over time. This section outlines the pivotal moments and discoveries that have shaped our understanding of fundamental particles, their interactions, and the forces governing them.
Early Discoveries
The journey into particle physics began in the late 19th century. James Clerk Maxwell's equations unified electricity and magnetism, paving the way for future discoveries. The discovery of the electron by J.J. Thomson in 1897 marked a significant milestone in understanding atomic structure. This was not just the identification of a new particle; it provided insight into the composition of atoms themselves, suggesting they were not indivisible as previously thought.
Further advancements came with Ernest Rutherfordโs gold foil experiment in 1909, which led to the conclusion that atoms have a nucleus. This experiment demonstrated that most of an atom is empty space, with electrons orbiting a dense center of protons and neutronsโa radical departure from existing models at the time. Such insights were foundational for future research.
Development of the Standard Model
The Standard Model of particle physics emerged in the mid-20th century as a robust framework for understanding the fundamental particles and their interactions. By the 1970s, physicists like Sheldon Glashow, Abdus Salam, and Steven Weinberg formulated the electroweak theory, which unified the electromagnetic force and weak nuclear force. This theoretical fusion was pivotal as it established a critical model for particle interactions.
The subsequent inclusion of quantum chromodynamics explained the strong nuclear force among quarks. By the 1980s and 1990s, experiments conducted at particle accelerators like CERNโs Large Electron-Positron Collider provided evidence supporting the Standard Model. This model successfully described all known particles, culminating with the prediction of the Higgs boson, which was eventually discovered in 2012 at the Large Hadron Collider. Thus, this model became a cornerstone in particle physics, offering a comprehensive understanding of the interactions within the universe.
Landmark Experiments
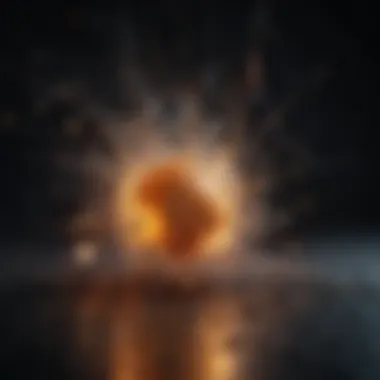
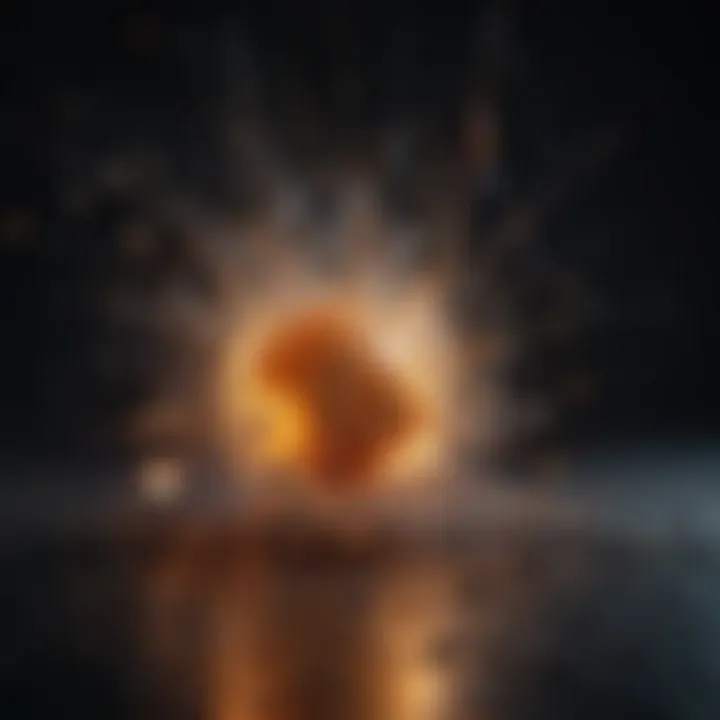
Landmark experiments have played a significant role in validating theoretical advances in particle physics. Many experiments helped to discover new particles or phenomena that enriched the Standard Model. For instance, the discovery of the muon in 1936 challenged existing theories about the decay of particles.
In the 1950s, the development of bubble chambers allowed for visualization of particle interactions. This technology made a significant impact, leading to discoveries such as the charmed quark in the 1970s. The findings from these experiments have been vital for confirming or refuting theoretical predictions.
"Experiments are the key to understanding the universe. They either confirm the theories we hold dear or necessitate a reevaluation of our concepts."
Overall, the historical overview succinctly charts the development of particle physics from its inception to the establishment of the Standard Model. It highlights how experimental breakthroughs have complemented theoretical advancements, providing a framework that continues to guide research today.
The Standard Model
The Standard Model is a cornerstone of particle physics. It provides a theoretical framework that explains the fundamental particles and forces in the universe. Through precise mathematical language, this model connects the observable phenomena we see in nature to a deeper understanding of the physical world. Its importance cannot be overstated as it forms the basis for much of modern particle physics, guiding researchers in their explorations.
Fundamental Particles
Fundamental particles are the building blocks of matter in the universe. They fall into three main categories: quarks, leptons, and gauge bosons.
Quarks
Quarks are essential constituents of protons and neutrons, which in turn form the nucleus of atoms. Each quark possesses a unique property named color charge that is crucial in strong interactions. There are six flavors of quarks: up, down, charm, strange, top, and bottom. Their significance lies in their role within the strong force, helping to bind protons and neutrons together, ensuring atomic stability.
The key characteristic of quarks is their fractional electric charge. This quality allows complex interactions within particles. However, quarks alone cannot exist in isolation due to confinement; they are always found in groups, typically as pairs or triples. This unique feature of confinement is advantageous when discussing particle creation and annihilation in high-energy environments.
Leptons
Leptons are another fundamental type of particle that includes the well-known electron, along with muons and taus. Neutrinos, which are nearly massless and interact very weakly, also belong to this category. Leptons are crucial because they do not experience the strong force, allowing them to exist independently in various forms throughout the universe.
One key characteristic of leptons is their role in weak interactions. This aspect is important in processes such as beta decay, where a neutron transforms into a proton. Leptons are a popular choice for investigation in particle physics due to their observable properties. Despite their low mass, their implications in astrophysics and cosmology, especially concerning neutrinos, give them substantial importance.
Gauge Bosons
Gauge bosons mediate the fundamental forces of nature. These include the photon, W and Z bosons, and gluons. Gauge bosons are essential in transmitting forces between matter particles, acting as the agents of interaction. For instance, photons mediate electromagnetic interactions, ensuring the stability of atoms while enabling a variety of electromagnetic phenomena.
The unique feature of these particles is their spin, which determines how they interact with other particles. Their role as force carriers makes them indispensable in the Standard Model. Understanding gauge bosons leads to insights into energy dynamics and fundamental forces.
Forces and Interactions
The forces among these particles are crucial for maintaining the structure of matter and enabling interactions. They can be classified into three main interactions: electromagnetic force, strong force, and weak force.
Electromagnetic Force
The electromagnetic force governs how particles with electric charge interact. It is one of the four fundamental interactions and is responsible for a wide array of phenomenon, from the behavior of atoms to the propagation of light. The electromagnetic force is characterized by the exchange of photons among charged particles.
This force enables the formation of atoms and molecules, essential for chemistry and biology. Its significance in this article arises from its foundational role in the universe and its observed effects.
Strong Force
The strong force is the most powerful of the four fundamental forces. It binds quarks together to form protons and neutrons and holds the atomic nucleus intact. This force operates through the exchange of gluons and exhibits characteristics such as confinement, where quarks cannot exist independently.
The strong force is crucial in particle collisions and reactions in high-energy environments, including those found in stars. Its unique nature is beneficial in explaining why atomic nuclei hold together against electromagnetic repulsion.
Weak Force
The weak force is responsible for certain types of nuclear decay, such as beta decay, which occurs in unstable nuclei. It operates at a very short range and involves the exchange of W and Z bosons. Its significance lies in its role in processes that change one type of particle into another, influencing particle stability and generation of neutrinos in the universe.
This force is lesser-known compared to electromagnetic and strong forces, yet it plays a critical role in the evolution of stars and other cosmic phenomena.
Higgs Boson Discovery
The Higgs boson discovery in 2012 was a milestone in particle physics. Its identification at CERN's Large Hadron Collider provided crucial evidence for the Higgs field, which permeates the universe and imparts mass to fundamental particles. The Higgs boson essentially confirms the Standard Model by validating the mechanism described by Peter Higgs and others.
The search for the Higgs boson intensified understanding of mass generation and opened discussions about beyond the Standard Model theories. Its discovery paved pathways for investigations into dark matter and other unresolved questions in particle physics. The ongoing study of the Higgs boson continues to shape modern research, holding potential keys to understanding the cosmos on a deeper level.
"The discovery of the Higgs boson is not just a triumph; it represents a deeper insight into the fundamental nature of the universe."
Particle Interactions
Understanding particle interactions is fundamental to grasping the dynamics of particle physics. These interactions determine how particles collide, scatter, and transform, contributing significantly to our broader understanding of physical processes in the universe. Various interaction types not only reveal underlying principles of matter but also facilitate the detection and study of fundamental particles. Thus, knowledge of these interactions is crucial for both theoretical and experimental physicists.
Types of Interactions
Elastic and Inelastic Scattering
Elastic scattering occurs when two particles collide and bounce off each other without changing their internal states. This type of interaction is critical because it preserves the kinetic energy of the system. It is characterized by the angle at which particles scatter, depending on their momenta and energies before the interaction. The ability to measure these angles allows scientists to deduce properties about the particles involved, such as mass and charge.
In contrast, inelastic scattering involves a change in the internal energy state of one or both particles after the interaction. This phenomenon can lead to the production of new particles, providing insights into processes that cannot be observed through elastic interactions alone.
- Key Characteristic: Elastic scattering maintains total kinetic energy, while inelastic scattering converts some energy into mass.
- Popular Choice for This Article: The discussion of both types highlights how particle collisions give us critical data about particle properties.
- Advantages and Disadvantages: Elastic scattering is simpler to analyze but limited in the information it provides. Inelastic scattering, while more complex, often reveals new physics.
Decay Processes
Decay processes refer to the transformation of unstable particles into more stable particles as they lose energy over time. This phenomenon includes various mechanisms, such as beta decay and alpha decay. Studying decay processes offers a glimpse into the stability and lifetimes of particles. Furthermore, they allow us to understand fundamental forces that govern particle interactions.
- Key Characteristic: Decay processes involve a particle losing energy, leading to a change in form or state.
- Beneficial Choice for This Article: These processes illustrate how particles interact with each other and their environments over time, providing temporal data that is invaluable for theorizing particle behavior.
- Advantages and Disadvantages: While decay processes are observable and provide significant information about particle lifetimes, they can lead to uncertainties due to the random nature of the decay events.
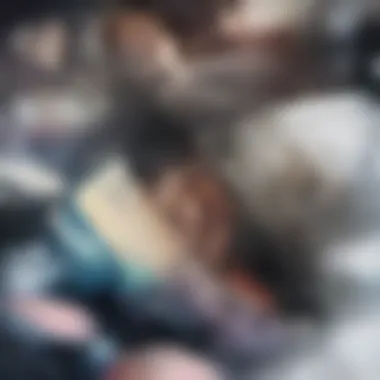
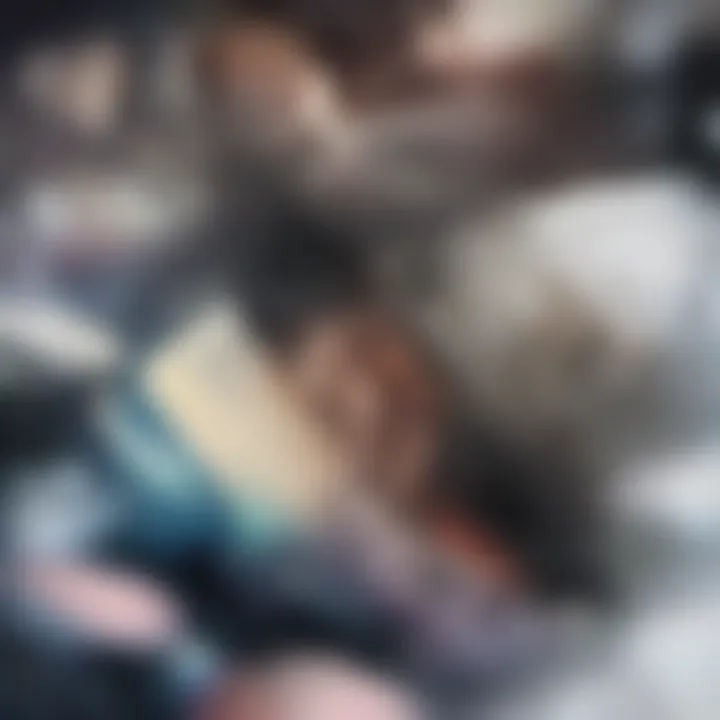
Cross-Section and Scattering Theory
Cross-section is a measure of the probability of an interaction occurring between particles. It is an essential concept in scattering theory, quantifying the effective area for an interaction that can occur in a given process. A larger cross-section indicates a higher likelihood of interaction, hence more observable events in collecting experimental data.
Scattering theory aids in predicting the outcomes of particle collisions based on initial and final states of involved particles. This framework incorporates various approaches, from classical mechanics to quantum field theories. In particle physics, understanding the scattering processes and their theoretical implications can reveal crucial details about the fundamental forces at work.
The significance of particle interactions cannot be overstated; they form the backbone of experimental particle physics, driving discoveries that redefine our comprehension of the universe.
Experimental Techniques
Experimental techniques are foundational in particle physics, enabling scientists to probe the fundamental particles that compose our universe. These techniques play a crucial role in validating theories, corroborating the existence of particles, and measuring their properties. The advances in experimental approaches drive the field forward, allowing for deeper insight into both established concepts and evolving theories.
Particle Accelerators
Functionality
Particle accelerators are essential tools in modern particle physics. They function by accelerating charged particles to high speeds, often approaching the speed of light, before directing them to collide with other particles. This collision creates conditions similar to those just after the Big Bang, allowing scientists to study newly formed particles from these interactions. The key characteristic of particle accelerators is their ability to produce extremely high-energy collisions, which result in the creation of a variety of particles.
One beneficial aspect of particle accelerators is their versatility. They can be designed for different types of experiments, from probing fundamental forces to searching for new particles. However, the substantial energy requirements and complex engineering involved in constructing these facilities can be considerable drawbacks.
Major Facilities
Major facilities for particle acceleration are strategically vital in advancing particle physics research. These typically include large colliders like the Large Hadron Collider at CERN and the Fermilab National Accelerator Laboratory in the United States. Their contributions extend beyond simple particle production; they enable extensive collaborative research efforts and garner global attention.
A key characteristic of these facilities is their capacity for conducting large-scale experiments involving thousands of scientists and engineers. This collaborative environment fosters the sharing of knowledge and resources, which is beneficial for innovative research. However, the significant financial investment and the long timelines required for development can pose challenges.
Detectors and Measurement
Detectors are crucial for capturing data from particle interactions resulting from collisions in accelerators. They measure various properties such as energy, momentum, and charge of the produced particles. Detectors vary in design, including those that use electromagnetic and hadronic techniques to differentiate between various particles. This allows researchers to derive meaningful insights from the vast amounts of data generated in experiments.
Effective measurement techniques must offer precision to ensure that results are reliable. Advanced technologies, such as silicon trackers and calorimeters, provide detailed information about the events occurring during collisions. However, building and maintaining these detectors can be resource-intensive and complex.
Data Analysis Techniques
Data analysis techniques are integral to interpreting the vast amounts of information produced by experiments. These methods involve sophisticated algorithms and computational techniques to clean, classify, and analyze data efficiently. Statistical methods play a crucial role in distinguishing between signal and background noise, allowing physicists to identify significant results with high confidence.
The growing role of machine learning in automating data analysis is noteworthy. With the increase in data volume derived from experiments, automated techniques can significantly enhance efficiency and accuracy. Nevertheless, developing these complex algorithms requires extensive expertise in both physics and computer science.
Current Research Trends
Current research trends in particle physics are pivotal for understanding the complex fabric of the universe. As we delve deeper into the fundamental aspects of matter and energy, new pathways reveal themselves that challenge established theories and suggest potential extensions to our knowledge. These trends not only highlight where the scientific inquiry is headed but also emphasize the significance of exploring questions that lie beyond the Standard Model of particle physics.
Beyond the Standard Model
Supersymmetry
Supersymmetry is a theoretical framework proposed to address several shortcomings of the Standard Model. One of its specific aspects is the expectation of partner particles for each known particle. This symmetry implies that every fermion has a bosonic partner and vice versa. This relationship could help unify the forces of nature and provide potential solutions to issues like dark matter.
The key characteristic of supersymmetry is its potential to stabilize the mass of the Higgs boson, addressing what is known as the hierarchy problem. Supersymmetry remains a popular area of study in this article due to its implications for unifying particle physics, cosmology, and gravity.
However, there are challenges associated with it. One unique feature is the predicted mass range of supersymmetric particles, which has not yet been confirmed by experiments. Thus, while it offers advantages in theoretical integration, its empirical validation has become a point of contention in the particle physics community.
String Theory
String theory presents a different foundational concept than traditional particle physics. This theoretical framework suggests that the fundamental constituents of the universe are not point-like particles but rather one-dimensional strings. These strings can vibrate at different frequencies, and their various vibrational modes correspond to different particle types.
The key characteristic of string theory is its ability to potentially unify quantum mechanics and general relativity. This makes it a beneficial part of this article as it attempts to encompass not only particle interactions but also gravitational phenomena.
A unique feature of string theory is its requirement of additional dimensions beyond the familiar four-dimensional spacetime. However, this aspect can also pose challenges; primarily, the lack of direct experimental evidence makes it more speculative than other theories currently being tested. Despite these challenges, string theory remains an essential consideration in advancing our understanding of the universe.
Dark Matter and Dark Energy
Dark matter and dark energy are two of the most intriguing subjects in current research. Dark matter accounts for approximately 27% of the universe's total mass and energy but remains elusive, as it does not emit light or interact with electromagnetic forces. Its existence is inferred from gravitational effects observed in galaxies and galaxy clusters. Understanding its composition could lead to significant breakthroughs in particle physics.
Dark energy, conversely, makes up about 68% of the universe and is driving its accelerated expansion. Research into dark energy focuses on understanding its nature and influence on cosmic evolution, which could also involve revisions to existing theories in physics. The potential synergy between dark matter and dark energy research continues to be an active area of investigation.
Quantum Gravity
Quantum gravity represents one of the crowning challenges in modern theoretical physics. It seeks to harmonize the principles of quantum mechanics with those of general relativity. As the quest to understand gravity at a quantum level continues, various approaches have emerged. These include loop quantum gravity and causal set theory, among others. Researchers hope that insights into quantum gravity could redefine our perception of spacetime and lead to a deeper understanding of the universe.
Efforts in quantum gravity intersect significantly with particle physics, offering potential frameworks that unite the known forces within a single theoretical construct. The implications of these studies can reshape our understanding of foundational aspects of the cosmos.
The quest to understand current trends in particle physics is not just about answering existing questions; it is about exploring the very nature of reality.
Applications of Particle Physics
Particle physics has significant applications that extend beyond theoretical realms. These applications have the potential to transform various fields, including medicine and industry, showcasing the far-reaching impact of particle research. Understanding these applications highlights the importance of ongoing research in particle physics and its contributions to practical problems.
Medical Applications
Diagnostics
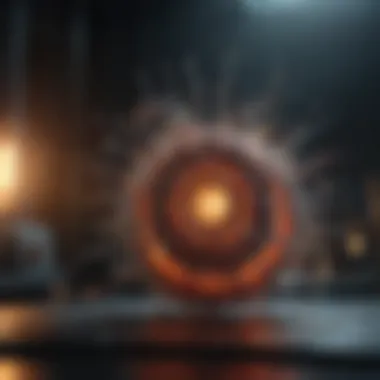
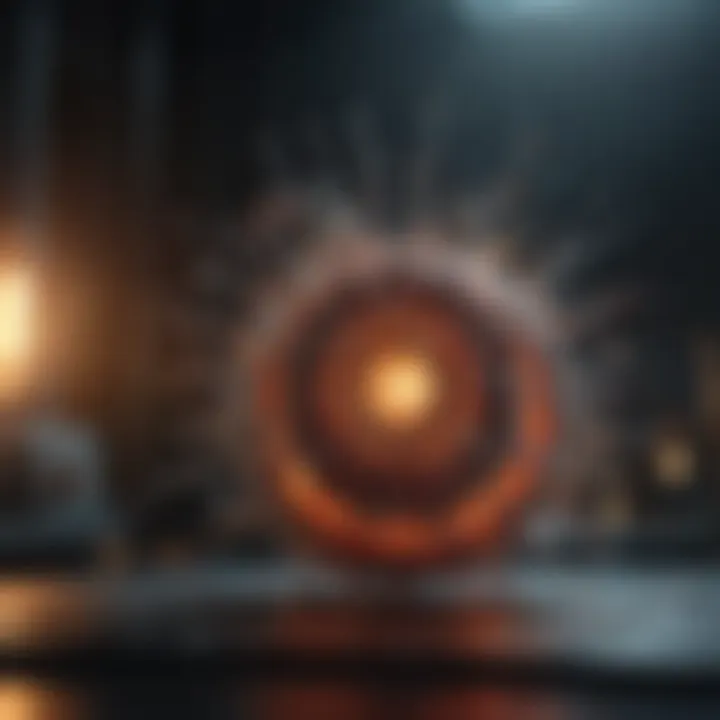
Diagnostics in medicine has benefitted from particle physics research, specifically through the use of imaging techniques such as positron emission tomography (PET). This method allows clinicians to visualize metabolic processes in the body, aiding in the early detection of conditions like cancer. The unique characteristic of PET is its ability to provide functional imaging, which is more informative than standard anatomical imaging techniques. This is why it is a popular choice among diagnostic tools. Additionally, the sensitivity of this method means that it can detect changes in the body sooner than traditional imaging methods, which enhances the potential for effective treatment.
However, one disadvantage is the use of radioactive tracers, which necessitates stringent regulations surrounding their use and disposal. This aspect raises important considerations regarding patient safety, cost, and accessibility in healthcare settings.
Treatment Techniques
Treatment techniques derived from particle physics include radiation therapy, widely used in the treatment of cancer. This method targets malignant cells using high-energy radiation, effectively damaging their ability to grow and divide. The key characteristic of radiation therapy is precision. Advances in this field, such as proton therapy, offer precision that spares surrounding healthy tissue. This makes it a beneficial choice for targeted cancer treatment, particularly in cases where tumors are located near sensitive organs.
One unique feature of treatment techniques in particle physics is their adaptability and ongoing improvement. New technologies continuously emerge to enhance treatment effectiveness and reduce side effects. Nevertheless, these advanced techniques often come with high costs and require specialized facilities, which may limit their availability in some regions.
Industrial Uses
Radiation in Manufacturing
In manufacturing, radiation plays a crucial role in quality control processes and material characterization. One specific aspect is the application of gamma ray testing for detecting flaws in welded joints and metal structures. This non-destructive testing method is valuable because it assures the integrity of materials without damaging them. The key characteristic of radiation in this context is its penetrating ability, which allows for internal inspections.
This application is beneficial as it increases safety and reliability in industries such as construction and aerospace. However, the use of radiation demands careful handling and adherence to safety regulations, which can complicate its application in regular manufacturing workflows.
Material Analysis
Material analysis techniques, informed by particle physics principles, are critical for assessing the composition and properties of various materials. Techniques such as neutron activation analysis allow researchers to determine the elemental composition of materials. This method is highly sensitive and can detect trace amounts of elements. The unique feature of this analysis is its non-destructive nature, making it suitable for studying artifacts and samples without damage.
This application is beneficial for research, industry, and even environmental monitoring. However, the complexity of the process and the need for specialized equipment might limit its use in smaller laboratories or by individual researchers.
"A thorough understanding of particle physics can enhance technologies across sectors, emphasizing its importance beyond theoretical confines."
Overall, the applications of particle physics in medicine and industry illustrate its relevance and utility. As research evolves, it continues to pave the way for new advancements that address contemporary challenges, underscoring the importance of ongoing studies in this dynamic field.
Implications for Fundamental Science
The exploration of particle physics holds substantial implications for our understanding of fundamental science. This field offers insights that extend beyond mere particles and forces; it reveals the basic components that constitute our universe. The discoveries in particle physics have a profound effect on various scientific disciplines, influencing theories in cosmology, philosophy, and even technology.
Understanding the Universe
In grasping the intricate workings of particle interactions, we gain a clearer perspective on the universe's structure and origins. This understanding is pivotal because:
- Cosmological Insights: Particle physics helps explain phenomena such as the Big Bang, dark matter, and dark energy. These elements are crucial for our understanding of cosmic evolution.
- Matter-Antimatter Asymmetry: One significant question is why our universe is predominantly matter. Research into particle interactions sheds light on this imbalance, which is essential for understanding the observable universe.
- Fundamental Forces: By studying the fundamental forces such as electromagnetism and gravity, scientists can develop a more comprehensive view of how different aspects of physics relate to each other. This integrated perspective is vital for constructing unified theories.
"The quest to understand particle physics is essentially the quest to comprehend the universe itself. No aspect is too small to matter."
Philosophical Considerations
The implications of particle physics extend into philosophical realms, posing questions that challenge existing paradigms and open up new dialogues. Some key considerations include:
- Nature of Reality: Particle physics invites reflection on what constitutes reality. If particles like quarks and electrons are indeed the building blocks of everything, it raises questions about existence and perception. How do we perceive an underlying reality that is so small and seemingly intangible?
- Determinism vs. Free Will: Quantum mechanics introduces probability and uncertainty into the fabric of reality. This presents challenges to deterministic philosophies, suggesting that outcomes are not strictly predictable, thus impacting notions of free will and determinism.
- Ethics of Research: As we delve deeper into particle physics, ethical considerations arise, especially concerning experimental methods and technology applications. There is a need for ongoing discussions about the moral implications of manipulating the fundamental constituents of nature.
Future Directions in Particle Physics
The exploration of future directions in particle physics is an essential aspect of this field, shaping the trajectory of research and technological advancement. The pursuit of new knowledge drives scientists to investigate phenomena that challenge current theories, ultimately leading to a deeper understanding of the universe. This section will focus on upcoming experiments and the integration of artificial intelligence in the field.
Upcoming Experiments
Future experiments are pivotal in expanding our grasp of fundamental particles and their interactions. One of the most significant upcoming projects is the Large Hadron Collider (LHC) at CERN, where scientists are planning to enhance its capabilities in the search for rare particles. The experiments aim to delve deeper into unresolved questions, such as the nature of dark matter and the potential existence of supersymmetry.
Other notable experiments include:
- Muon g-2 experiment: This project seeks to understand the anomalies in the behavior of muons, which may reveal new physics beyond the Standard Model.
- DUNE (Deep Underground Neutrino Experiment): This aims to study neutrinos, crucial for exploring the matter-antimatter imbalance in the universe.
- ICARUS: This detector will play a key role in neutrino physics, located in a near-surface facility to study events induced by neutrino interactions.
These initiatives not only promise to enhance theoretical frameworks but also aspire to lead to discoveries that could revolutionize our understanding of particle physics.
The Role of Artificial Intelligence
Artificial intelligence (AI) is increasingly becoming an integral part of particle physics, providing transformative capabilities in data collection, analysis, and modeling. The complexity of data generated from high-energy particle collisions necessitates innovative approaches. AI's ability to process vast amounts of information quickly ensures that researchers can glean insights more efficiently.
Some key areas where AI is making an impact include:
- Data analysis: Machine learning algorithms are utilized to identify patterns within experimental data that may otherwise remain hidden.
- Simulation and modeling: AI aids in creating more accurate simulations of particle interactions, offering predictive insights that can guide further research.
- Optimization of experiments: By analyzing experimental designs, AI can suggest modifications to maximize the efficiency and effectiveness of upcoming experiments.
These advancements exemplify how the synergy between particle physics and artificial intelligence can foster innovation and discovery. As we delve into these future directions, it is clear that the ongoing research efforts signify a commitment to enhancing human understanding of the universe.
Epilogue
In this article, we have journeyed through the intricate realm of particle physics, illuminating essential concepts, historical milestones, and current research trends. Each section contributed to a multifaceted understanding of how particles govern the fabric of the universe.
Summary of Key Points
The exploration began with an introduction to particle physics, defining its scope and importance in modern science. We then reviewed the historical overview, from early discoveries to the establishment of the Standard Model. The discussion of fundamental particles, interactions, and experimental techniques allowed us to appreciate the complexity of the field. Recent advancements in research trends, including investigations beyond the Standard Model, provided insights into unresolved questions such as dark matter and quantum gravity.
- Historical milestones shaped particle physics.
- The Standard Model explains fundamental particles.
- Neutron and proton interactions occur through strong forces.
- Experimental techniques involve particle accelerators.
- Ongoing research aims to explore the unknown realms of physics.
The Importance of Ongoing Research
Ongoing research in particle physics cannot be overstated. It plays a critical role in advancing scientific knowledge and has far-reaching implications for various fields. Continued investigation leads to technological innovations, some that filter into everyday life. For instance, medical imaging techniques, like PET scans, have roots in particle physics. As researchers delve deeper, they open doors to novel phenomena that could redefine our understanding of the universe.
As the boundaries of our knowledge expand, we uncover complexities that challenge existing theories, prompting the need for new frameworks. This cycle of inquiry and discovery drives scientific progress, ultimately enhancing our comprehension of fundamental questions about existence and the universe.
"The pursuit of particle physics not only reveals the universe's secrets but also fosters a quest for knowledge that transcends disciplinary boundaries."
By fostering a culture of inquiry, we prepare the next generation of scientists to tackle the challenges ahead, ensuring a vibrant future for research in particle physics. We must remain committed to this endeavor, appreciating the significance of both small-scale discoveries and grand theories.