Exploring Biological Breakthroughs: Transformations and Innovations in Life Sciences
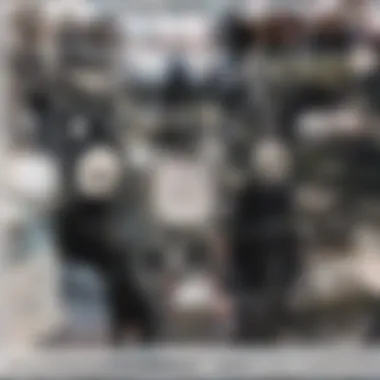
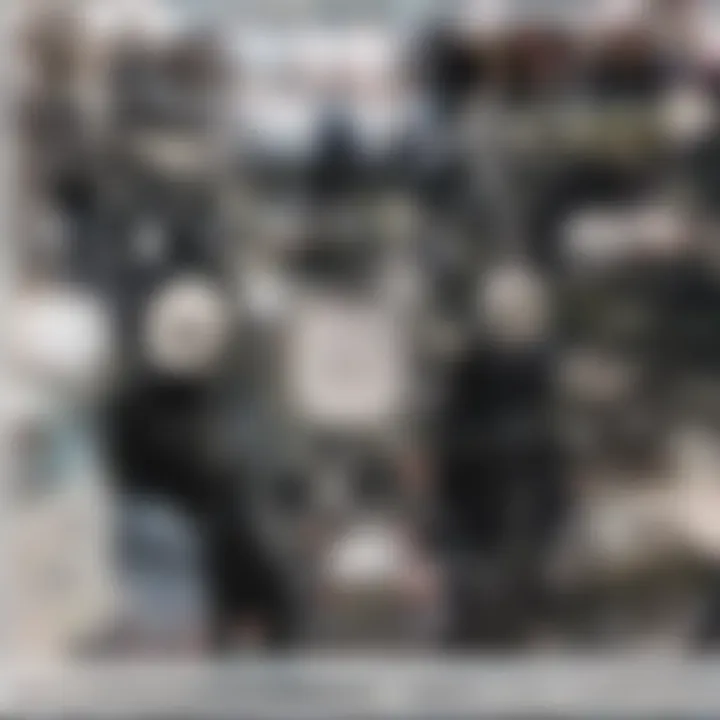
Intro
The field of life sciences is currently undergoing a transformative phase marked by groundbreaking research and innovative approaches. These biological breakthroughs have significant implications, offering insights into complex biological systems and potential applications that can enhance health outcomes, environmental sustainability, and overall societal well-being.
Key Research Findings
Overview of Recent Discoveries
In recent years, research in biotechnology, genomics, immunology, and synthetic biology has progressed rapidly. Key discoveries have emerged, such as the development of CRISPR-Cas9 technology, which allows for precise genome editing. This technology has paved the way for advancements in gene therapy, agriculture, and medicine.
Moreover, the mapping of the human microbiome has illuminated the complex relationships between microorganisms and human health. Studies indicate that these microbial communities play a crucial role in digestion, immunity, and even mental health. Researchers are now exploring how to manipulate these microbiomes for therapeutic purposes.
Significance of Findings in the Field
These breakthroughs carry vast implications. For instance, gene editing can potentially eradicate genetic disorders and enhance crop resilience against climate change. Furthermore, understanding the microbiome can lead to personalized medicine, where treatments are tailored to an individualโs unique microbial composition. Such developments are not only promising but also necessary in addressing pressing global challenges, like food security and disease management.
"Advancements in life sciences represent a leap towards not just understanding, but also manipulating biological systems for human benefit."
Breakdown of Complex Concepts
Simplification of Advanced Theories
Many of the concepts in life sciences can seem complex at first glance. CRISPR technology, for instance, involves understanding nucleotides, enzymes, and cellular mechanisms. Simplifying these concepts for broader comprehension often requires clear definitions and relatable examples. A simplified explanation could describe CRISPR as a way for scientists to cut and paste DNA in a highly controlled manner, akin to editing a text document.
Visual Aids and Infographics
Visuals play a key role in educating audiences about advanced theories. Infographics can illustrate processes like genome editing or the human microbiome's interaction with the body. Charts and diagrams help break down sophisticated information into digestible parts, making it more accessible to students and professionals alike.
Defining Biological Breakthroughs
Understanding what constitutes a biological breakthrough is essential for appreciating the advancements within life sciences. These breakthroughs represent significant leaps in knowledge and technology. They can transform existing paradigms and open doors to new ways of thinking about biology. Whether through discoveries that illuminate cellular processes or innovations that enable precise manipulation of genetic material, each breakthrough carries the potential to reshape our understanding and application of biological science.
In the context of this article, biological breakthroughs are not merely events or discoveries; they reflect the progressive nature of scientific inquiry. They lead to improved treatments, enhanced understanding of diseases, and novel approaches to conservation. Each breakthrough contributes to a collective knowledge that influences public health, environmental policy, and technology. The importance of defining these breakthroughs lies in recognizing their implications for future research and societal impact.
What Constitutes a Breakthrough?
A biological breakthrough typically meets several criteria:
- Novelty: It presents a new concept or technique that has not been previously explored or recognized.
- Significance: The breakthrough must have substantial implications for science, medicine, or ecology. This might include innovative methods for disease treatment or new insights into genetic functions.
- Validation: The discovery should withstand rigorous testing and peer review, assuring the scientific community of its validity.
- Application: Finally, a genuine breakthrough offers real-world applications that can enhance human life, health, or the environment.
Overall, the definition of a breakthrough encompasses the excitement of discovery and the practicality of application, emphasizing both theoretical advances and tangible benefits.
Historical Context of Breakthroughs in Biology
The evolution of biology is marked by pivotal breakthroughs that redefined scientific landscapes. Historically, milestones such as the discovery of penicillin by Alexander Fleming in 1928 revolutionized medicine. It ushered in the age of antibiotics, transforming the treatment of bacterial infections and saving millions of lives.
Furthermore, the structure of DNA, unveiled by James Watson and Francis Crick in 1953, fundamentally altered our perception of genetics. This advance triggered the field of molecular biology, paving the way for advancements in genetic engineering and biotechnology.
Another important shift occurred with the Human Genome Project. Completed in 2003, this endeavor mapped the entirety of human DNA, a breakthrough that has profound implications for personalized medicine, genetic research, and evolutionary studies.
Each of these historical breakthroughs showcases not only the progression of scientific thought but also the interconnectedness of discoveries over time. They highlight how past innovations lay the groundwork for future explorations in biology, illustrating the continuum of knowledge within the scientific community.
"Historical breakthroughs are the stepping stones that pave the way for contemporary advancements in biology."
These historical examples demonstrate how defining biological breakthroughs illustrates shifts in scientific paradigms and societal applications, further emphasizing the ongoing evolution of life sciences.
Recent Advances in Biotechnology
Biotechnology has ushered in an era of unprecedented innovation, fundamentally transforming various sectors such as medicine, agriculture, and environmental science. Recent advances in this field leverage biological systems and organisms to develop solutions that address complex challenges. These advancements not only aim to improve human health but also enhance food security and contribute to sustainable development. In this article, we will focus particularly on the remarkable advancements in gene editing through CRISPR technology and the evolving field of synthetic biology.
CRISPR and Gene Editing
Mechanism of CRISPR
The CRISPR (Clustered Regularly Interspaced Short Palindromic Repeats) system is a revolutionary tool in genetic editing. Its mechanism involves a guide RNA that directs the Cas9 protein to a specific location on the DNA strand, where it creates a double-strand break. This ability to cut DNA at predetermined sites is what makes CRISPR a powerful option for genetic modifications. One key characteristic of CRISPR is its precision, allowing for targeted alterations with minimal off-target effects. This has positioned CRISPR as a beneficial choice for various scientific and medical applications. A unique feature of CRISPR is its simplicity and low cost, making it accessible for many laboratories worldwide, thus accelerating research and applications in genetic engineering.
Applications in Medicine and Agriculture
The applications of CRISPR in both medicine and agriculture are vast. In medicine, CRISPR is being explored for therapies targeting genetic disorders such as sickle cell disease and cystic fibrosis. The ability to edit genes at precise locations allows for the treatment of conditions that were previously deemed untreatable. Meanwhile, in agriculture, CRISPR technology enables the development of crops that are resistant to pests and diseases, requiring fewer chemicals and improving yield sustainability. Its key characteristic is this dual versatility, making it a popular tool in both sectors. However, the unique feature of rapid advancements in these applications raises questions about regulatory standards and public acceptance, which needs addressing to harness its full potential.
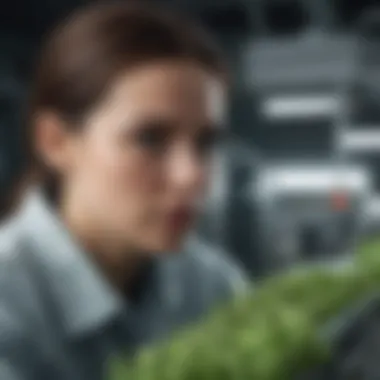
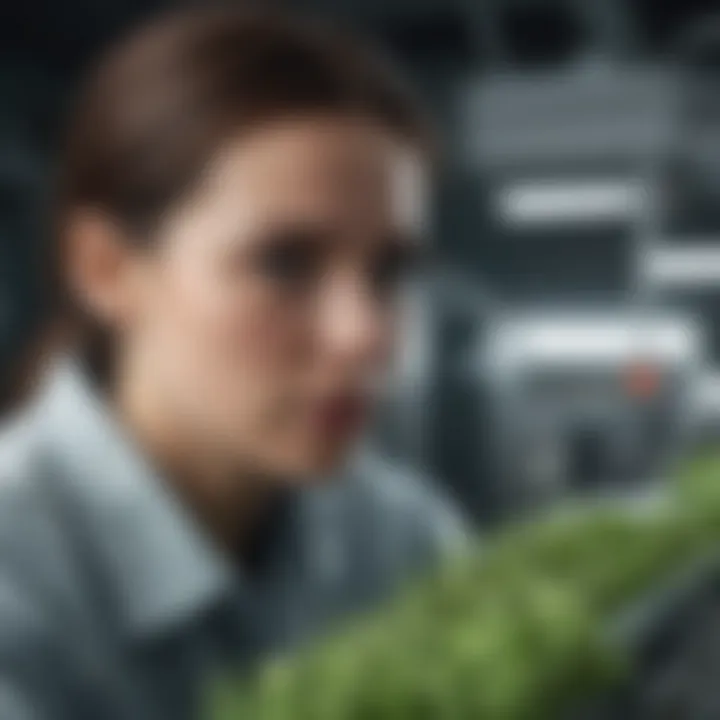
Ethical Implications
As we venture further into gene editing, ethical implications arise. The discussions surrounding germline editing, which alters genes passed on to future generations, poses significant ethical questions. A key characteristic of this debate is the societal impact of playing a role in human evolution. This is a critical choice for our article, as ethical considerations must keep pace with scientific progress. Unique features of this discourse include potential disparities in access to these technologies, which might exacerbate existing inequalities. Furthermore, ethical frameworks need to evolve to ensure responsible use in research and application.
Synthetic Biology
Building Biological Parts
Synthetic biology aims to design and construct new biological parts and systems. This burgeoning field allows scientists to manipulate biological components to address pressing biological challenges. A key characteristic of synthetic biology is its interdisciplinary approach, merging engineering principles with biological research. This makes it a beneficial tool for developing innovative solutions. The unique feature of this science is its capacity to create synthetic organisms with tailored functions, offering advantages in medical therapies and biofuels. However, ethical considerations and ecological concerns must be navigated cautiously while developing these organisms.
Applications in Energy and Materials
In the realms of energy and materials, synthetic biology has the potential to revolutionize production processes. Innovations such as biofuels made from engineered microbes demonstrate how biological systems can replace traditional fossil fuels. The key characteristic of these applications is sustainability, providing greener alternatives to current practices. This makes them a popular focus of research. The unique feature of harnessing microorganisms to produce high-value chemicals, like biodegradable plastics, offers significant advantages over conventional chemical manufacturing. However, the scalability of these technologies remains a challenge needing further exploration.
Challenges and Future Directions
While the potential of synthetic biology is vast, several challenges require attention. Regulatory hurdles can limit progress, as establishing suitable guidelines is complex. The key characteristic of these challenges is the balancing act between innovation and safety. This makes addressing these issues a critical part of the future of biotechnology. A unique feature of the upcoming directions in synthetic biology includes greater integration with data science to enhance research and development. As we look forward, fostering collaboration among scientists, regulators, and the public will be instrumental in overcoming these obstacles.
Genomics and Its Impact
Genomics has rapidly become a cornerstone in the study of biological sciences. The exploration of genomes provides insight into the fundamental building blocks of life. This article focuses on the advancements in genomics and how they affect various fields such as medicine, agriculture, and environmental science. The implications are vast, affecting how we understand genetic disorders and develop new therapies.
As we delve into specific topics within genomics, the focus is on both the technological advancements and the broader societal impacts. Emphasizing the integration of genomics with other disciplines illustrates its role in fostering interdisciplinary research.
Next-Generation Sequencing Technologies
Advancements Over Traditional Methods
Next-generation sequencing technologies represent a significant leap over traditional sequencing methods. These technologies allow massive amounts of DNA to be sequenced quickly and accurately. One key characteristic of next-generation sequencing (NGS) is its capacity to produce data at a much lower cost and in a fraction of the time compared to earlier methods like Sanger sequencing. The high throughput of NGS provides researchers with comprehensive information, promoting innovative research across many life science fields.
One unique feature of NGS is its ability to analyze numerous samples simultaneously, making it a scalable solution for large studies. However, challenges such as data management and quality control still remain pertinent to this technology. Despite these challenges, the benefits of applying NGS greatly outweigh the disadvantages.
Data Analysis and Interpretation
Data analysis and interpretation are crucial components of genomics. With the vast amount of data generated by sequencing technologies, effective analysis methods have become necessary. The key characteristic of data analysis is its role in transforming raw sequencing data into meaningful biological insights. This process involves utilizing bioinformatics tools that enable researchers to decode complex genomic information.
The unique feature of modern data analysis is the integration of artificial intelligence techniques, which enhance data interpretation and predictive modeling. While these advancements lead to greater accuracy in findings, there remains the issue of computational resource demands, which can be a barrier for some research settings.
Personalized Medicine
Personalized medicine is an emerging aspect of genomics that tailors medical treatment to the individual characteristics of each patient. The key characteristic of this approach lies in its focus on genetic information to guide treatment options. Personalized medicine holds potential for significantly improving therapeutic efficacy and minimizing adverse effects. It uses genetic profiles to select the suitable drug and dosage for each patient, which enhances overall treatment outcomes.
One unique feature of personalized medicine is its proactive nature, allowing healthcare professionals to anticipate health issues and address them effectively. However, challenges regarding cost, accessibility, and ethical considerations persist.
Human Genome Project and Beyond
Significant Findings
The Human Genome Project, completed in 2003, produced many significant findings that shifted the understanding of human genetics. One key characteristic of this project was its mapping of the entire human genome, identifying over 20,000 genes. This monumental effort has been instrumental in genetic research and biotechnology applications. The benefit of such detailed genetic information allows for targeted research on hereditary diseases and complex traits.
However, there are limitations regarding the understanding of gene functions and interactions that remain under investigation.
Implications for Human Health
Implications for human health have been profound since the completion of the Human Genome Project. Insights gained from the project have paved the way for biomarker development, allowing early detection of diseases. The key feature is the potential to create preventive strategies based on individual genetic predispositions. This shift towards preventive healthcare can significantly reduce the incidence of certain diseases.
Yet, ethical concerns regarding genetic privacy and discrimination continue to be debated in this context.
Contributions to Evolutionary Biology
Contributions to evolutionary biology have been notable since the Human Genome Project. The comprehensive mapping of the human genome has enabled a deeper understanding of human evolution and genetic diversity. One defining trait is the ability to compare human DNA with that of other species, enriching the study of phylogenetics and evolutionary relationships.
These comparisons lead to insights into traits that are unique to humans, aiding in the understanding of evolutionary adaptations. Nevertheless, challenges such as interpreting the meaning behind genetic variations require constant scientific investigation.
Immunology Breakthroughs
Immunology breakthroughs play a crucial role in advancing our understanding of diseases and developing therapies. With the rise of previously unmanageable illnesses, the ability to manipulate immune responses opens numerous avenues for treatment. These innovations not only address current health crises but also prepare the infrastructure for tackling future health challenges.
Vaccine Development Innovations
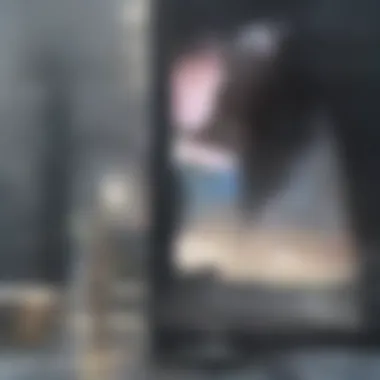
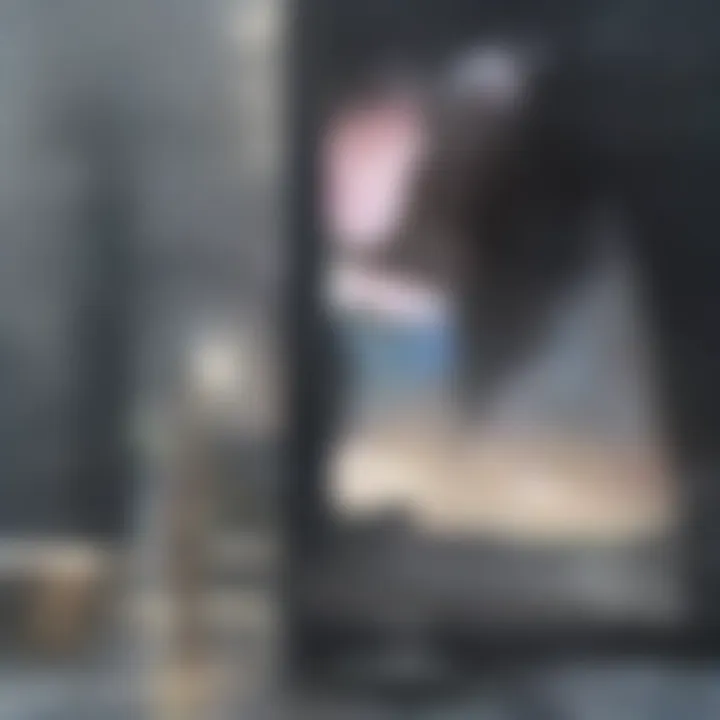
Messenger RNA Vaccines
Messenger RNA vaccines, like the ones developed for COVID-19, represent a pivotal innovation in vaccine technology. They use a small strand of genetic material to instruct cells to produce a harmless piece of the virus, generating an immune response without introducing live pathogens. This is a significant advancement due to its speed of development and adaptability for new variants. The key characteristic is its ability to provoke a strong immune response while being easier to produce than traditional vaccines, making it a beneficial choice.
The unique feature of messenger RNA vaccines is their capacity to be modified swiftly, accommodating changes in viral genomes. This flexibility can be advantageous in managing outbreaks, but it also presents challenges relating to public trust and understanding around new vaccine technologies.
Challenges in Global Vaccination
Challenges in global vaccination remain significant. Distribution barriers, logistical challenges, and vaccine hesitancy affect vaccination campaigns worldwide. The key characteristic here is the disparity in access to vaccines between developed and developing nations. Addressing this issue is crucial for global health equity.
Uniquely, these challenges reveal systemic issues in healthcare infrastructure, causing delays and uneven access. The advantages of overcoming these barriers include a more equitable health response, yet failures to address them may result in prolonged outbreaks and continued health disparities across populations.
Future of Vaccine Development
The future of vaccine development looks promising, characterized by more personalized and effective vaccines. Innovations are aimed at creating broader protection against various strains of pathogens. The key characteristic of this future direction is the increased integration of technology and data analytics in vaccine design, providing a data-driven approach.
Its unique feature will likely involve combination vaccines that can target multiple diseases at once. This advancement offers the potential to improve public health outcomes but also poses challenges in regulatory approval processes and ensuring safety across combinations.
Immunotherapy for Cancer Treatment
Mechanisms of Action
The mechanisms of action in immunotherapy have transformed oncology by making it possible for the immune system to recognize and combat cancer cells more effectively. This method enhances the bodyโs natural defenses against cancer. The key characteristic is the activation and enhancement of immune responses tailored to individual tumor requirements, making it a powerful treatment option.
Uniquely, these mechanisms can foster immune memory that allows the body to combat recurring malignancies. While promising, challenges include dealing with immune-related side effects and understanding the complexity of patient-specific responses.
Clinical Trials and Success Stories
Clinical trials and success stories reveal the real-world impact of immunotherapy. They provide incrdible insights into treatment efficacy and patient experiences. The hallmark of these trials is the rigorous processes that ensure safety and effectiveness. These stories often highlight patient transformations, showcasing the profound impact of advancements in treatment.
The unique aspect of these success stories is their potential to inspire further research and investment in immunotherapy. However, it remains a challenge to ensure equitable access to these cutting-edge treatments amidst disparities in health systems.
Barriers to Access
Barriers to access for immunotherapy can undermine advancements made in this field. High costs, limited availability, and complex treatment regimens often restrict patient access. The key characteristic involves economic and systemic factors that limit the reach of these treatments.
The unique feature of these barriers shows how important it is to develop strategies for broader accessibility. While innovative solutions exist, without addressing these barriers, many may still be left without effective treatment options.
Emerging Fields in Biology
Emerging fields in biology are at the forefront of scientific evolution. They redefine our understanding of life and its interactions. Research in these areas brings forth technologies and insights that have profound implications for health, agriculture, and the environment. By investigating these frontiers, we can harness the potential of biology to solve complex problems.
Microbiome Research
Understanding Microbial Communities
Understanding microbial communities is essential for grasping how these organisms impact ecosystems and human health. Microbial communities consist of trillions of microorganisms living within and around us. They contribute to digestion, immunity, and even mental health. This section emphasizes how studying these interactions can create strategies for improving human health and environmental outcomes. The key characteristic of understanding microorganisms is their complexity. This complexity makes it a crucial area for research. The unique feature of this field is that it connects health and ecology, providing a holistic view of biological systems. However, challenges remain, such as the difficulty in culturing many microbes in a lab setting.
Health Implications
Health implications of microbiome research are substantial. The composition of microbial communities can influence disease susceptibility, metabolic health, and overall wellness. This area is gaining popularity due to the growing evidence linking gut health to a wide array of diseases, including obesity and autoimmune disorders. The key feature of this research is its potential for personalized medicine. By understanding an individualโs microbiome, a more tailored treatment plan can be developed. Nevertheless, the complexity of these interactions presents challenges. Not all relationships in microbiome studies are well understood, and this can complicate treatment approaches.
Therapeutic Applications
Therapeutic applications related to microbiome research are promising. This includes the use of probiotics to restore healthy microbial balance. Such applications are significant in treating conditions like irritable bowel syndrome and allergies. The strong characteristic of this approach is its non-invasive nature. Restoring the microbiome can be done through diet or supplements rather than more invasive methods. However, solo application of these therapies may not suffice. Complex interactions mean that a multifaceted approach is often necessary for effective treatment.
Bioinformatics and Computational Biology
Role of Data Analysis in Biology
The role of data analysis in biology cannot be overstated. With the volume and complexity of biological data increasing, bioinformatics provides necessary tools for interpretation. This discipline allows for the analysis of genomic, proteomic, and metabolomic data. Its importance lies in its ability to convert raw data into actionable insights. The key characteristic here is the integration of computer science and biology, which opens new avenues for research. A specific feature of this field is the use of algorithms to make sense of vast datasets. While data can provide crucial insights, over-reliance on computational predictions without biological validation can lead to errors in conclusions.
Applications in Drug Discovery
Applications in drug discovery have expanded due to bioinformatics. By analyzing biological data, researchers can identify new drug targets more efficiently. This marks a shift from traditional methods, where trial and error were commonplace. The key feature of current applications is the speed of analysis, allowing for high-throughput screening of potential drug candidates. Despite its advantages, there are limitations. Data quality and accessibility vary greatly, which can hinder research consistency.
Future Trends
Future trends in bioinformatics promise continued innovation. Areas such as artificial intelligence and machine learning are set to revolutionize how biological data is analyzed. These technologies can enhance predictive modeling and personal medicine approaches. The key consideration here is the impact on the speed and accuracy of biological research. A unique aspect is the ongoing integration of disciplines, leading to more comprehensive understandings of biological processes. Yet, the complexity of biological systems means that predictions may still hold uncertainty, thus requiring careful interpretation.
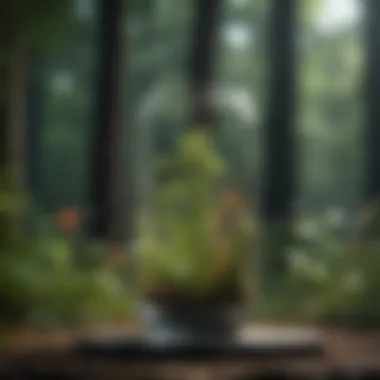
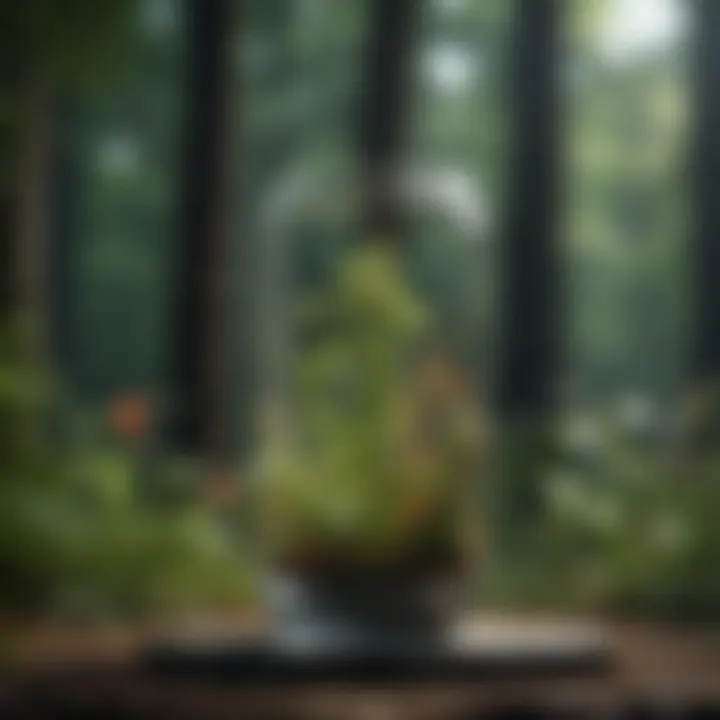
Ethical Considerations in Biological Research
Ethics play a crucial role in biological research. As advancements in genetics, biotechnology, and other disciplines accelerate, it becomes ever more essential to reflect on the moral implications of these innovations. Ethical considerations address potential impacts on society, the environment, and personal human rights. By exploring these matters, researchers and the public can engage in informed discussions about the challenges and responsibilities that come with scientific progress.
Ethical Dilemmas in Genetic Engineering
Societal Implications
Genetic engineering raises important societal implications. These implications often revolve around equity, safety, and the potential for discrimination. It is vital to consider who benefits from these technologies and who gets left behind. The ability to alter genetic traits poses questions about what it means to be human and whether certain attributes might be deemed more desirable than others.
Moreover, societies may face challenges regarding accessibility. The democratization of these advancements can foster a sense of fairness, while limited access can lead to societal divides. As such, understanding the societal implications holds significance in shaping future public policy and ensuring equitable access to benefits.
Regulatory Frameworks
Regulatory frameworks are essential to ensure that genetic engineering is carried out responsibly. These frameworks vary globally, influencing how research and applications are conducted. They aim to balance innovation with safety and ethical considerations.
Key characteristics of regulatory frameworks include their ability to adapt to emerging technologies. As genetic engineering evolves, regulations must also evolve to address new challenges. However, bureaucracy may slow down the pace of innovation. Therefore, finding a balance between comprehensive oversight and allowing flexibility is crucial.
Public Perception
Public perception significantly influences the acceptance of genetic engineering. This acceptance often hinges on trust in scientists and regulatory bodies. Negative media portrayals can lead to public fear and skepticism. When the public perceives research as misaligned with ethical standards, it can result in backlash against scientific endeavors.
A unique feature of public perception is its variability across different demographics. Cultural, educational, and socio-economic backgrounds shape opinions on genetic modifications. Engaging in open dialogue can help bridge gaps in understanding, fostering a more informed public.
Environmental Impacts of Biotechnology
Sustainability Considerations
Sustainability is a key consideration in the biotechnology field. Innovative biotechnological practices have the potential to create sustainable agricultural practices, reduce waste, and lessen reliance on fossil fuels. The role of biotechnology in promoting sustainability cannot be overstated.
Key characteristics include a focus on reducing human environmental footprints. However, there are challenges to consider, such as the sustainability of biotechnology itself. Some methods may lead to unforeseen consequences on ecosystems, necessitating careful evaluation.
Conservation Efforts
Conservation efforts are directly tied to biotechnological advancements. Techniques such as gene editing can enhance the adaptation of endangered species to changing environments. These innovations contribute to biodiversity conservation, potentially preventing extinctions.
Key characteristic of conservation efforts includes the need for balancing technological intervention with natural processes. Conservation strategies must align with ethical considerations, ensuring that interventions do more harm than good. Thus, awareness of these efforts fosters informed discussions in biology.
Long-term Effects on Biodiversity
The long-term effects of biotechnology on biodiversity are a topic of ongoing debate. While certain innovations can create positive outcomes, they may also lead to unexpected challenges. Monocultures, stemming from genetically modified crops, for instance, can harm ecological diversity.
Key feature of studying these effects is the emphasis on monitoring and adaptive management. Understanding the ecological consequences of biotechnological applications allows for more sustainable practices. Hence, this inquiry ensures that biological advancements align with the health of ecosystems.
Future Directions in Life Sciences
The future directions in life sciences signify a critical juncture for research and innovation. Technological convergence, increased interdisciplinary collaboration, and an emphasis on education create new opportunities. These advancements not only propel scientific discoveries but also address pressing societal challenges, such as health disparities and environmental crises. Therefore, understanding these elements is essential for anyone invested in the life sciences.
Integration of Technologies
Collaboration Across Disciplines
Collaboration across disciplines is pivotal in driving biological breakthroughs. Scientists, engineers, and social scientists collaborate to share unique insights and methodologies. This interdisciplinary approach fosters a culture of innovation. For instance, bioinformatics combines biology and computer science for enhanced data analysis in genomics. The distinct advantage of this collaboration is a holistic understanding of complex biological systems. However, maintaining effective communication between diverse fields can be a challenge, sometimes leading to misunderstandings in project goals or methodologies.
Impact on Research and Development
The impact on research and development is profound. The combination of various technologies accelerates the pace of scientific advancement. Modern developments, such as machine learning in drug discovery, exemplify this synergy. This approach achieves efficiency, but it also raises concerns about reliability and reproducibility of results. Emphasizing high-quality validation processes is necessary to ensure the integrity of findings.
Real-world Applications
Real-world applications of biological innovations bridge the gap between laboratory discoveries and societal needs. Advanced techniques in tissue engineering, vaccine development, and personalized medicine demonstrate tangible benefits. These applications address health care challenges directly. However, translating scientific research to practical solutions can face hurdles, such as regulatory approvals and public trust in novel technologies.
Preparing for the Next Generation of Scientists
Education and Training
Education and training are foundational to fostering the next generation of scientists. Programs that integrate ethical considerations into scientific education prepare students for real-world challenges. This holistic approach equips emerging scientists with not just technical skills but also an understanding of the societal context of their work. The downside could be the lengthy duration required to integrate these components deeply into curricula, potentially limiting immediate hands-on training.
Interdisciplinary Approaches
Interdisciplinary approaches enhance the problem-solving capabilities of upcoming scientists. By integrating disciplines like engineering, computer science, and biological sciences, students learn to tackle complex challenges innovatively. These methods promote creativity and adaptability but may complicate curriculum development due to varying academic standards.
Encouraging Public Engagement in Science
Encouraging public engagement in science cultivates a scientifically literate society. Efforts, such as community science projects, help bridge gaps between scientists and the public. This engagement increases awareness of scientific issues. However, ensuring accurate communication can be a hurdle, as misinformation may still disseminate widely, affecting public perception.
Conclusion: Future directions in life sciences hinge on integrating technologies and preparing the next generation of scientists. These elements are crucial for sustaining innovation and addressing global challenges efficiently. By collaborating across disciplines, focusing on impactful research, and emphasizing education, the field can reach promising new heights.