Bacteria Sensors: Innovations and Implications
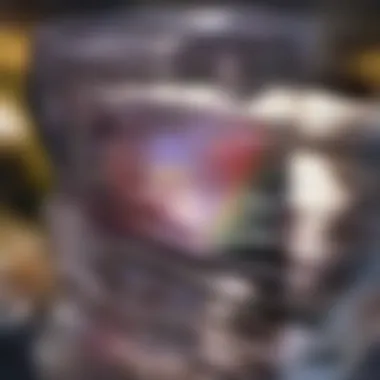
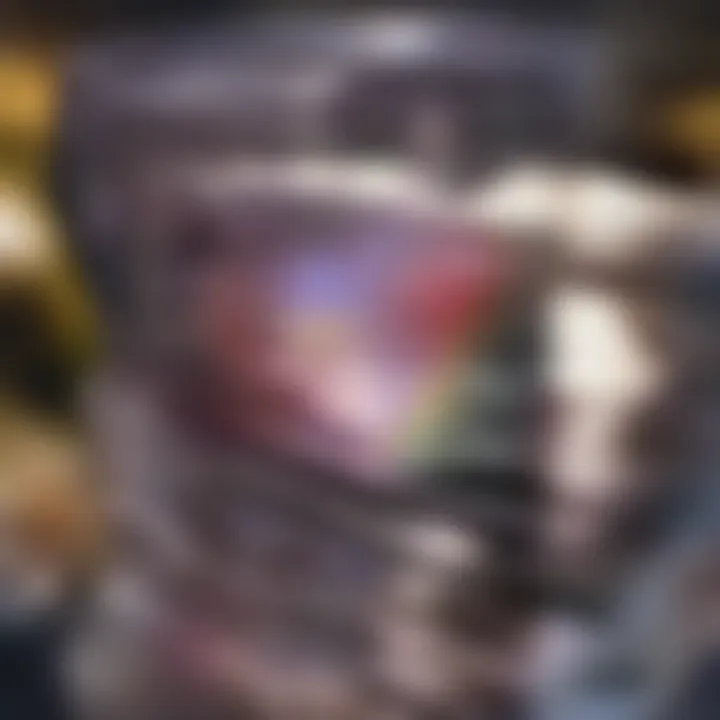
Intro
Bacteria sensors represent a crucial advancement in the field of microbial detection. These technologies play an instrumental role in various sectors, from public health to environmental monitoring. As the world increasingly faces challenges related to pathogens, these sensors offer innovative solutions. This article delves into the landscape of bacteria sensors, exploring recent findings, technologies, applications, and the future of this fascinating field.
Key Research Findings
Overview of Recent Discoveries
Innovations in bacteria sensor technology have surged recently. Researchers have developed various methods for bacterial detection, ranging from traditional culture methods to advanced biosensors. Nano-based sensors, for instance, demonstrate enhanced sensitivity, capable of detecting low-level bacterial contamination quickly. Recent studies highlight the efficacy of techniques such as CRISPR-based diagnostics, which revolutionize how we approach bacterial identification. These innovations not only provide rapid results but also increase accuracy, making them invaluable tools in clinical settings.
Significance of Findings in the Field
The implications of these findings are significant. Accurate and rapid detection of bacteria can lead to timely interventions in health crises. For example, in hospitals, sensors that detect antibiotic-resistant strains can efficiently manage outbreaks. Additionally, these technologies impact environmental safety, where monitoring water quality for bacterial content is essential.
"The continuous development of bacteria sensors is essential for safeguarding public health and the environment."
Understanding Sensor Mechanisms
To comprehend the advancements in bacteria sensors, understanding their mechanisms is necessary. Sensors generally utilize biological or chemical interactions to identify bacteria. For example, immunosensors employ antibodies that recognize specific bacterial antigens. Alternatively, electrochemical sensors use electrical signals generated by bacterial activity for detection. Each type has its strengths and limitations, influencing their applicability in diverse scenarios.
Application and Impact
Public Health Implications
Bacteria sensors are crucial in preventing and managing infectious diseases. Their application in healthcare settings supports rapid diagnosis and outbreak control. By promptly identifying the presence of pathogens, healthcare professionals can respond to infections effectively, minimizing potential health risks.
Environmental Monitoring
In the realm of environmental science, bacteria sensors play a pivotal role in monitoring ecosystems. These sensors help detect contamination in water bodies, ensuring public safety. They can identify bacterial loads, thus aiding in pollution control and restoration efforts.
Challenges and Future Prospects
While bacteria sensor technology shows promise, challenges remain. The need for standardization and cross-compatibility can hinder widespread implementation. Moreover, sensors must be cost-effective to be accessible for various applications. Future research aims to address these challenges, enhancing the efficiency and reliability of bacteria sensors. Collaboration between researchers and industry is essential for realizing the full potential of these technologies.
The End
Preamble to Bacteria Sensors
The interplay between science and technology has birthed innovative tools for microbial detection, notably bacteria sensors. These sensors are pivotal in a multitude of fields such as public health, environmental monitoring, and industrial applications. Understanding this topic is crucial for grasping the mechanisms of these sensors and their implications on society. Innovations in bacteria sensors hold the promise of enhancing detection methods, allowing for quicker and more accurate results in various contexts.
Definition and Purpose
Bacteria sensors are analytical devices designed to identify and quantify the presence of bacteria in various samples. The main purpose is to provide reliable data on microbial populations, which is vital for ensuring safety in food production, medical diagnoses, and environmental assessments. These sensors utilize sophisticated techniques to detect specific bacterial strains, thus serving both research and practical applications. They help in mitigating risks associated with bacterial contamination.
Historical Context
The development of bacteria sensors can be traced back to the early attempts in microbiology. Originally, culturing methods were the standard for bacteria detection, needing substantial time and resources. Over time, technologies evolved from classic culturing towards more advanced methods like molecular techniques in the 1980s. These innovations laid the groundwork for the sensors we have today.
Furthermore, the emergence of nanotechnology and biosensors in the late 20th century marked a significant turning point. They allowed for real-time monitoring of bacterial presence, paving the way for applications that we now take for granted.
Importance in Modern Science
In the context of modern science, the importance of bacteria sensors cannot be overstated. They play a crucial role in public health by enabling rapid pathogen detection, which is essential in preventing outbreaks. Environmental monitoring benefits from these sensors as they detect harmful bacteria in water supplies and ecosystems. Moreover, in industrial settings, such as biotechnology and pharmaceuticals, these sensors ensure product safety and quality.
"The development of bacteria sensors stands as a cornerstone for guaranteeing safety across various sectors, impacting health policies and environmental strategies."
In summary, bacteria sensors represent a vital intersection of technology and microbiology, facilitating advancements in safety and efficiency. This understanding of their definition, historical development, and significance in contemporary research highlights their growing relevance in a world facing the challenges posed by microbial threats.
Types of Bacteria Sensors
Understanding the types of bacteria sensors is crucial for advancing microbial detection technology. Each type offers unique capabilities and specific applications, which have significant implications for health, safety, and industry. By studying different sensors, we can better appreciate their roles in improving public health responses, environmental monitoring, and various scientific disciplines.
Optical Sensors
Optical sensors represent a powerful method for detecting bacteria. They operate based on the interaction of light with bacterial cells. These sensors can identify bacteria by measuring changes in light properties such as absorbance, fluorescence, and refractive index. One of the prominent benefits of optical sensors is their high sensitivity and the ability to provide real-time data. This characteristic is particularly useful in clinical diagnostics or food safety testing.
Optical sensors, such as surface plasmon resonance devices, have shown substantial promise in detecting pathogens rapidly. However, they can be influenced by factors such as turbidity or color in the sample, which may hinder accuracy. Furthermore, the initial setup cost and lack of portability can pose limitations in some scenarios.
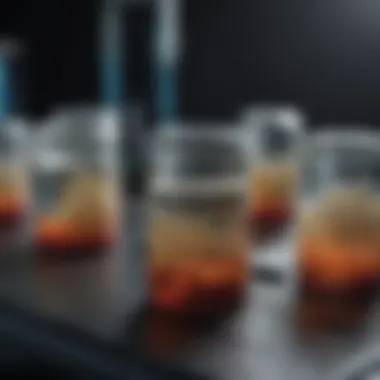
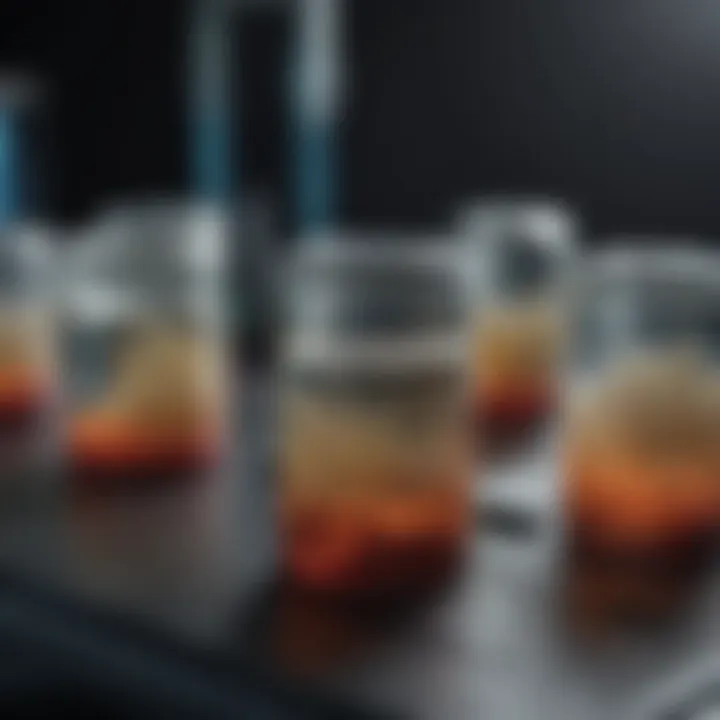
Electrochemical Sensors
Electrochemical sensors rely on electrochemical reactions to detect bacteria. Typically, these sensors measure changes in current or voltage as bacteria interact with specific electrodes. They offer several advantages, including portability, cost-effectiveness, and ease of use in various conditions.
For example, devices based on screen-printed electrodes allow for rapid testing without extensive sample processing. They can detect a range of pathogens, making them suitable for fieldwork, especially in environments with limited resources. Yet, challenges like maintaining sensor stability over time and the potential for false positive reactions must be addressed for broader application.
Microbial Biosensors
Microbial biosensors integrate living cells or cellular components for the detection of bacteria. These devices leverage biological recognition elements to produce measurable signals in response to specific microbial targets. The sensitivity of microbial biosensors often outperforms many traditional methods, allowing for the detection of pathogens at lower concentrations.
Such biosensors can be tailored for different applicationsโfrom monitoring food safety to testing hospital environments for healthcare-associated infections. However, integrating living cells poses challenges regarding stability and longevity. Furthermore, variations in microbial behavior due to environmental factors can affect reliability, presenting a significant hurdle for consistent results.
Nanotechnology in Bacteria Sensing
The application of nanotechnology in bacteria sensing is transforming the landscape of microbial detection. Nanoscale materials can enhance the sensitivity and selectivity of sensors, allowing for the identification of bacteria usually missed by larger-scale sensors. Techniques using gold nanoparticles or carbon nanotubes have demonstrated significant improvements in signal amplification and detection limits.
The use of nanotechnology also promotes miniaturization, making sensors more flexible and applicable in diverse environments. However, there are regulatory and safety concerns associated with nanomaterials. Understanding the interaction of nanoparticles with biological systems is essential as this area progresses.
"The integration of advanced materials at the nanoscale offers promising avenues for improved detection and response strategies in bacteria sensing."
In summary, recognizing the types of bacteria sensors informs not only the scientific community, but it also shapes practical applications in public health, environmental protection, and industrial settings. Each type comes with its own set of strengths and challenges, making it imperative to continue research and development in this evolving field.
Mechanisms of Action
Understanding the mechanisms of action is crucial for comprehending how bacteria sensors function. These sensors rely on intricate biological and physical processes to detect microbial presence. The effectiveness of these sensors hinges on two main aspects: the principles of detection and the techniques utilized. Each element plays a significant role in achieving accurate and rapid identification of bacteria. Without these mechanisms, the reliability of sensors would be severely compromised.
Detection Principles
Biological Recognition
Biological recognition serves as a foundational aspect of bacteria sensors. It involves the specific interaction between the sensor's recognition element and the target bacteria. This specificity ensures that the sensor can accurately identify particular microorganisms, which is essential for applications in public health and environmental monitoring. The key characteristic of biological recognition is its high selectivity, which prevents false positives in complex samples.
A unique feature of biological recognition is its basis in biochemistry. This aspect allows for the development of sensors that can operate under various conditions. The advantages are clear: higher specificity often leads to more reliable results. However, there can be disadvantages as well. For instance, the stability of recognition elements can fluctuate, impacting the sensor's long-term performance.
Signal Transduction
Signal transduction involves the conversion of biological signals into measurable electrical signals. This principle is vital for translating biological interactions into data that can be analyzed. A critical characteristic of signal transduction is its ability to provide real-time monitoring of bacterial presence. This capability makes it a preferred choice in rapid diagnostics, where time is often of the essence.
The unique aspect of this process lies in its versatility. Different types of signals can be transduced, allowing for various sensor designs. The advantages here include quick response times and the potential for miniaturization. However, signal transduction can suffer from challenges associated with signal noise, which could lead to inaccuracies if not managed properly.
Detection Limitations
While detection principles are essential, understanding their limitations is equally important. Detection limitations refer to the constraints that sensors face in accurately identifying bacteria. A noticeable characteristic is the sensorโs detection limit, which is the smallest concentration of bacteria that can be reliably detected. Understanding these limits is critical for effective application in settings where low concentrations of bacteria are of concern.
The unique feature of detection limitations is their influence on sensor design. Sensors must be tailored to overcome specific thresholds to increase their utility. The advantages of addressing these limitations include improved accuracy in a broader range of scenarios. On the downside, exceeding the detection capacity can result in significant errors, thus undermining the sensor's reliability.
Techniques Used
Fluorescence
Fluorescence techniques are widely employed in bacteria sensors due to their sensitivity and specificity. This method relies on the emission of light by a substance that has absorbed light. The core characteristic of fluorescence is its ability to detect low concentrations of bacteria effectively, making it popular in research and clinical applications.
A unique feature of this technique is its capability for multiplexing, allowing simultaneous detection of multiple bacterial species. Its advantages include high sensitivity, but it is also subject to interference from environmental factors, which can complicate results if not carefully managed.
Impedance
Impedance measurement offers a different approach to detecting bacteria by monitoring changes in electrical resistance. The key benefit of this technique lies in its label-free detection, meaning there is no need for fluorescent markers. This simplicity contributes to a lower cost and faster analysis time.
The unique aspect of impedance measurement is its ability to provide real-time monitoring of bacterial growth. Its advantages are significant, especially in food safety applications. However, variations in sample conditions can affect results and limit the applicability of this technique in diverse environments.
Mass Spectrometry
Mass spectrometry is a powerful analytical technique used for identifying bacteria by evaluating the mass-to-charge ratio of ions. The critical charateristic of mass spectrometry is its ability to provide highly detailed information about individual bacterial species, offering insights into their composition and structure.
One unique feature of this method is its capability for high-throughput analysis, making it suitable for large sample volumes. The advantages encompass high specificity and sensitivity. However, it requires complex equipment and skilled personnel, which can hinder accessibility for some labs.
Understanding the mechanisms of action not only illuminates the ways to improve these sensors but also enhances their application in real-world scenarios. The intricate balance between sensitivity, specificity, and practicality defines the future direction of bacteria sensor development.
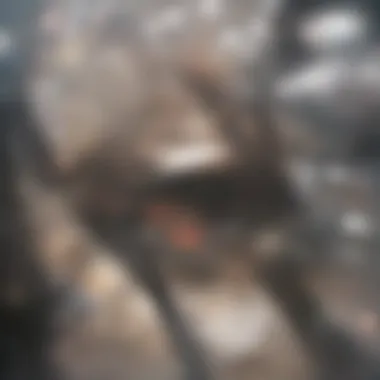
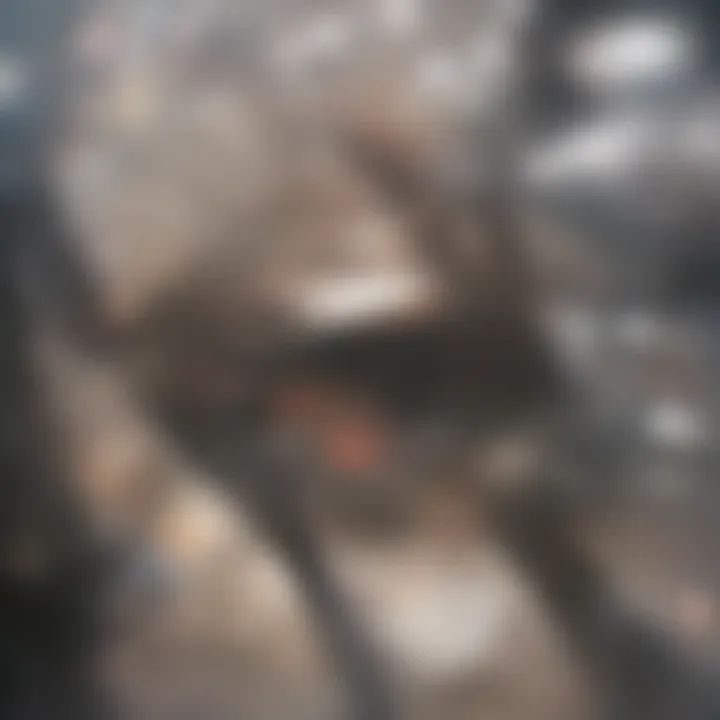
Applications of Bacteria Sensors
The realm of bacteria sensors has found diverse applications, spanning from public health to industrial uses. Their significance cannot be understated, as they play a crucial role in ensuring safety and advancing scientific knowledge. These sensors not only aid in detecting harmful microorganisms but also contribute to broader environmental sustainability and agricultural productivity. As we delve into the various domains of these applications, it becomes clear that the benefits are multifaceted.
Public Health
Pathogen Detection
Pathogen detection is a cornerstone of public health initiatives. The ability to swiftly identify harmful bacteria in environments such as hospitals, public venues, and food establishments is vital. The key characteristic of pathogen detection sensors lies within their accuracy and rapid response times. This ensures that outbreaks are addressed promptly, minimizing risk to the population.
A significant advantage is their capacity to detect multiple pathogens simultaneously, which enhances screening efficiency. However, challenges such as false positives and the necessity for specialized training can hinder their effectiveness in certain settings.
Food Safety Monitoring
Food safety monitoring employs bacteria sensors to ensure food products are free from contamination. These sensors offer continuous real-time data, making them a preferred choice for the food industry. A key feature of food safety monitoring is its preventive approach, which minimizes the chances of food-borne illnesses before products reach consumers.
The main benefit includes the integration of sensor technology in production processes, leading to improved safety protocols. Nevertheless, the cost of deploying these systems can pose a barrier for smaller enterprises, potentially limiting their widespread adoption.
Water Quality Assessment
Water quality assessment using bacteria sensors focuses on ensuring that drinking water and natural bodies of water are safe for consumption. These sensors detect pathogenic bacteria and assess overall water quality by providing immediate results. This ability to monitor changes rapidly is a defining feature that makes water quality sensors essential in both urban and rural settings.
One key advantage is the direct impact on public health, as timely intervention can prevent water-related diseases. However, sensors often require regular maintenance and calibration, which can pose logistical challenges in remote locations.
Environmental Monitoring
Bioremediation Efforts
Bioremediation efforts utilize bacteria sensors to monitor the efficacy of using microorganisms in cleaning contaminated environments. This application highlights a sustainable approach, as it leverages natural processes to restore ecological balance. A significant aspect of bioremediation is its ability to provide real-time feedback on pollutant degradation, allowing for timely adjustments in strategy.
Despite its advantages, such as cost-effectiveness and environmental friendliness, potential drawbacks include the complexity of environmental variables that can affect sensor readings.
Impact Assessments
Impact assessments look at the ecological effects of various industrial activities. Bacteria sensors play a vital role in measuring microbial health in ecosystems, providing data integral to evaluating environmental policies. Their key characteristic is the ability to offer precise measurements that inform decision-making processes.
This application supports sustainable development initiatives, but relies heavily on data interpretation, which can be influenced by external contaminants.
Pollution Tracking
Pollution tracking involves the ongoing assessment of contaminants in various environments, using bacteria sensors to identify and quantify microbial indicators of pollution. This is a critical aspect of regulatory compliance and environmental protection. The speed at which these sensors can detect pollution levels is a valued benefit, allowing for immediate responses to potential threats.
However, the need for sophisticated infrastructure to support real-time data transmission can limit the implementation of such tracking systems in developing regions.
Industrial Applications
Biotechnology Processes
In biotechnology processes, bacteria sensors are crucial for monitoring fermentation and other biological reactions. Their key characteristic lies in providing real-time data on microbial populations and their health, critical for optimizing production yields. This application has gained popularity due to its potential to enhance efficiency and product quality.
The unique feature here is the automation of data collection, which reduces human error. However, concerns about the technological feasibility of integrating these sensors often arise.
Pharmaceutical Development
Pharmaceutical development utilizes bacteria sensors to monitor the presence of contaminants during drug formulation. This is essential for ensuring the safety and efficacy of pharmaceuticals. Their ability to deliver quick and accurate results supports quality assurance processes, making them popular in the industry.
One unique advantage is their capacity to minimize the risk of product recalls due to contamination. Nonetheless, the high initial setup cost can restrict adoption among smaller pharmaceutical firms.
Crop Health
Bacteria sensors used in crop health assessment help farmers monitor soil and plant microbiomes. The integration of these sensors in agriculture indicates a shift towards precision farming, where monitoring promotes better yields and sustainable practices. A key characteristic is the ability to provide targeted information that can guide agricultural decisions.
Their unique feature lies in the link between microbial health and soil health. However, dependency on sensor technology may create barriers for farmers who lack technical expertise in managing such tools.
Challenges in Bacteria Sensor Development
The development of bacteria sensors is not without its obstacles. Addressing these challenges is crucial for advancing the field and ensuring that these sensors serve their intended purposes effectively. An understanding of the technical limitations, regulatory hurdles, and market accessibility issues is vital. Each element plays a significant role in the overall landscape of microbial detection technology, impacting research, public health initiatives, and environmental monitoring efforts.
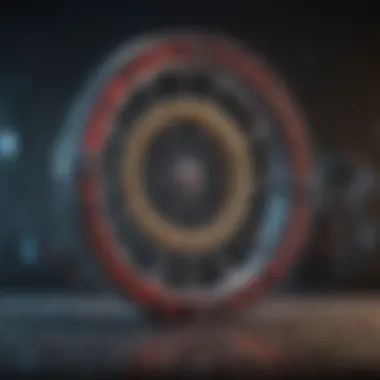
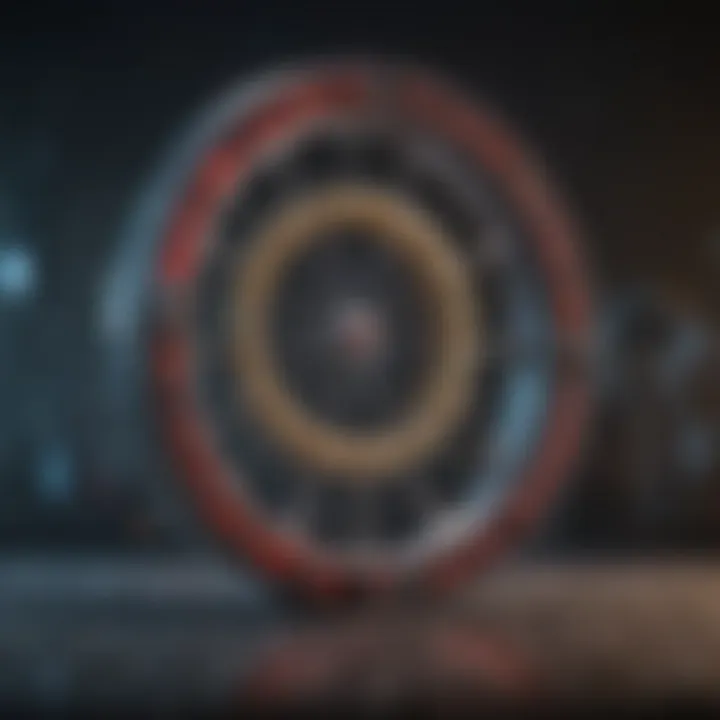
Technical Limitations
False Positives and Negatives
False positives and negatives are critical technical concerns in the realm of bacteria sensing. False positives occur when a sensor incorrectly indicates the presence of bacteria that are not actually there. This can lead to unnecessary actions, such as wasteful decontamination or patient treatment. Conversely, false negatives happen when the sensor fails to detect valid bacterial presence. This is especially dangerous in medical diagnostics, where missed detections can result in untreated infections.
The accuracy of detection determines the reliability of results, hence making it a priority in sensor development. These inaccuracies in measurement can arise from various factors, including environmental conditions and sensor calibration. A significant characteristic of false positives and negatives is their potential to undermine public trust in detection technologies. When the outcome of a test is uncertain, it discourages users from relying on these technologies. The benefit of focusing on this aspect lies in improving sensor design, leading to heightened specificity and sensitivity.
Cross-Sensitivity
Cross-sensitivity refers to a sensor's inability to distinguish between different types of bacteria or between bacteria and other biological materials. This issue contributes to the overall complexity of microbial detection processes. For instance, a sensor designed to detect E. coli may also react to other bacteria, leading to misleading results. This characteristic of cross-sensitivity complicates the interpretation of sensor data, which is critical in applications such as food inspection and clinical diagnostics.
Effective sensor validation is essential to mitigate the effects of cross-sensitivity. Identifying these limitations is beneficial in developing targeted solutions that enhance sensor performance. The unique feature of devising robust detection algorithms can help differentiate among closely related microorganisms, which would improve assay accuracy and efficacy.
Scalability Issues
Scalability issues refer to the challenges associated with producing sensors on a larger scale while maintaining quality and consistency. As demand for effective bacteria sensors grows in various sectors, the capacity to scale production while ensuring reliability becomes paramount. Production methods capable of mass-producing high-quality sensors are essential for widespread implementation.
One characteristic of scalability is the need for automated production environments to reduce human error and cost. This aspect is beneficial in fostering quick responses to growing market needs without sacrificing quality. Balancing production costs and sensor performance is a unique challenge, requiring innovative approaches and technologies to ensure scalability does not compromise sensitivity and specificity.
Regulatory Hurdles
Regulatory hurdles significantly impact the bacteria sensor market. Developing and bringing new sensors to market requires compliance with strict regulations from health and safety organizations. These regulations ensure that sensors are safe, effective, and reliable for public use. The process includes rigorous testing and validation, which can be time-consuming and costly.
Navigating regulatory frameworks can be a challenge for small enterprises and startups, often resulting in delayed launch timelines. In this landscape, extensive research and thorough documentation become mandatory. Understanding regulatory requirements can facilitate smoother development pathways and improve market acceptance.
Market Accessibility
Market accessibility encompasses the ability of consumers to acquire and utilize bacteria sensors effectively. Factors such as pricing, availability, and competition play a vital role in determining market health. High production costs can lead to inflated prices, limiting access for consumers, especially in developing regions. This limited accessibility can hamper advancements in public health efforts and environmental monitoring.
Manufacturers must evaluate the balance between production costs and technology quality to ensure broad accessibility. Developing affordable yet high-quality sensors is crucial for ensuring that these innovations reach underserved markets. Additionally, education and training initiatives can enhance user capability, fostering a better understanding of how to effectively utilize these sensors for public health and safety.
Future Directions
The exploration of bacteria sensors is at a pivotal juncture. Future directions in this field hold significant implications for technological advancement and public health. Innovations or potential developments could drastically enhance detection capabilities, making sensors more accurate and efficient. Furthermore, integrating different technologies, such as artificial intelligence, could facilitate real-time analysis and predictive modeling. Therefore, understanding and addressing these future possibilities is essential to advance microbial detection further.
Innovations on the Horizon
Several advancements are on the radar for bacteria sensors. This includes miniaturization, which allows for portable devices that can be used in various settings, from laboratories to fieldwork. Recent developments in nanomaterials endow sensors with improved sensitivity and specificity against a broader range of bacteria.
Additionally, the implementation of biosensors with bioinformatics capabilities allows the analysis of complex data sets, thereby providing richer insights into microbial behavior. Such innovations may revolutionize early detection in medical scenarios and enhance safety measures in food production.
Integrating AI in Bacteria Sensing
Artificial intelligence is becoming a crucial element in bacteria sensing technologies. AI algorithms can analyze data from sensors in real-time, identifying patterns and predicting bacterial presence before it becomes a larger issue. This predictive capability is particularly beneficial in public health situations.
Machine learning models can learn from an extensive database of microbial characteristics, improving detection accuracy over time. By automating data analysis, researchers can significantly reduce response time to microbial outbreaks. This integration leverages the power of AI while complementing existing technologies to forge a more resilient detection framework.
Collaborations in Research
Collaborative efforts between academia, industry, and government are vital for advancing bacteria sensor technology. Interdisciplinary research initiatives can facilitate knowledge sharing and pool resources, enabling more robust studies. Partnerships could lead to innovative projects that tackle current limitations in sensor technology.
These collaborations also promote a pragmatic approach to addressing challenges like regulatory hurdles or market accessibility. By working together, stakeholders can align their goals, ensuring that developments in bacteria sensors meet both scientific and public health needs effectively.
"The future of bacteria sensors hinges on innovation and collaboration, creating an ecosystem of advanced detection methodologies that could protect public health and environmental safety."
The path forward for bacteria sensors is bright, shaped by innovative solutions, technological integrations, and collaborative research efforts.
End
The conclusion serves a crucial purpose in summarizing the significance of bacteria sensors within the broader context of microbial detection. As outlined throughout this article, these sensors are not merely technical devices; they embody innovations that have vast implications for public health, environmental monitoring, and industrial applications.
Key elements of the topic include:
- The advancement of sensor technology that enables rapid and accurate detection of bacterial pathogens.
- The integration of these sensors into vital systems for food safety, water quality, and disease outbreak management.
- Future innovations that may incorporate artificial intelligence, enhancing detection capabilities even further.
The benefits of these developments are substantial. For instance, timely detection of pathogens can prevent outbreaks and safeguard public health. Moreover, in an environmental context, these sensors can play an essential role in tracking pollution and assessing the efficacy of bioremediation efforts.
However, considerations surrounding the reliability of these sensors remain. Issues such as false positives, false negatives, and regulatory compliance must be addressed to ensure widespread adoption.
"In the realm of microbial detection, the precision of bacteria sensors could very well dictate the efficiency of response strategies across multiple sectors."
In synthesizing the information presented, it becomes evident that the evolution of bacteria sensors is not just a matter of scientific interest but a vital element influencing contemporary public policy, healthcare, and industry standards. The future landscape of microbial detection will undoubtedly be shaped by ongoing innovations and the collaborative efforts of researchers, educators, and industry professionals. Embracing these advancements will pave the way for a safer and more efficient society.