Understanding Universe Simulation: A Comprehensive Exploration
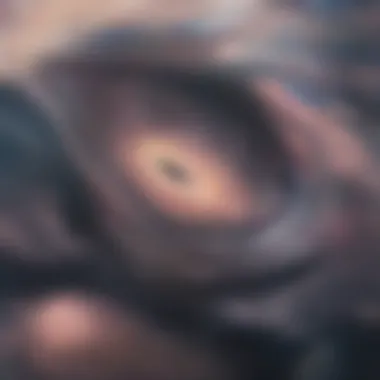
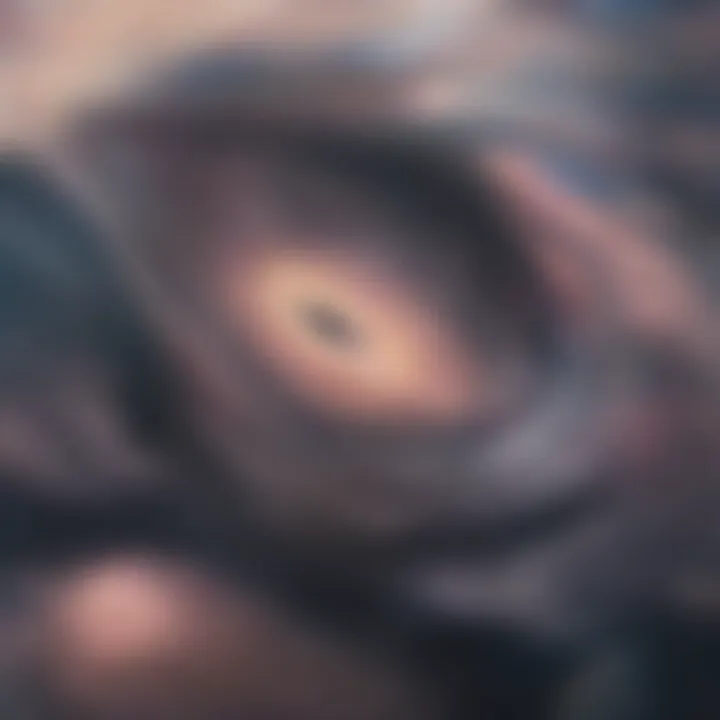
Intro
The concept of universe simulation brings together complex theories and cutting-edge technology, enabling researchers to explore cosmic phenomena in unprecedented ways. These simulations serve as computational models that mimic the dynamics of the universe. As our understanding of astrophysics deepens, so too does the necessity to accurately model celestial systems. This article aims to provide a meticulous exploration of universe simulation, addressing its theoretical underpinnings as well as its practical implications for scientific inquiry and philosophical discourse.
Advancements in computational capabilities have made it feasible to create detailed simulations. However, each model comes with its strengths and limitations. Understanding these nuances is crucial for researchers and educators alike, as it shapes how we interpret data and theories in astrophysics. In the coming sections, we will delve into significant research findings, clarify intricate concepts, and discuss the relevance of these simulations in various scientific fields.
Key Research Findings
Overview of Recent Discoveries
Recent advancements in universe simulation have illuminated key discoveries regarding cosmic structures. High-resolution simulations have revealed intricate details about galaxy formation and evolution. Studies using tools such as the Illustris simulation have provided insights into the distribution of dark matter and its role in shaping galaxies. Moreover, the interplay between dark energy and galaxy clusters is becoming clearer, fostering a deeper understanding of the universe's expansion.
Significance of Findings in the Field
The insights gained from these simulations hold substantial significance.
- Understanding Cosmology: Simulations help affirm or challenge existing models of cosmic evolution.
- Guiding Observational Studies: Results from simulations often direct observational programs, allowing astronomers to target specific celestial phenomena for further study.
- Educating Future Researchers: These findings serve as crucial teaching tools in academia, offering students a glimpse into advanced astrophysical concepts.
"Simulation is not merely a tool; it is a fundamental aspect of modern astrophysical research that redefines our cosmic narrative."
Breakdown of Complex Concepts
Simplification of Advanced Theories
Understanding the underpinning theories of universe simulation can be daunting. Some of the complex ideas include N-body simulations, hydrodynamic processes, and cosmological parameters. To simplify:
- N-body Simulations: These focus on gravitational interactions, allowing researchers to study the behavior of astronomical bodies over time.
- Hydrodynamic Simulations: These incorporate fluid dynamics to understand gas flow in the universe, crucial for modeling star formation.
- Cosmological Parameters: Constants such as the Hubble constant are vital for simulating different epochs of cosmic history.
Visual Aids and Infographics
Visual representations enhance comprehension significantly. For instance, visualizations of galaxy formations can provide an immediate intuitive grasp of complex interactions.
In forthcoming sections, we will delve deeper into the methodologies, technological advancements, and ethical considerations surrounding universe simulations. This exploration aims to enrich our understanding of not just the cosmos, but also the very nature of reality we seek to comprehend.
Intro to Universe Simulation
Universe simulation is a pivotal tool in modern astrophysics, serving as a bridge between theoretical concepts and empirical research. With the capacity to reconstruct cosmic phenomena, these simulations enhance our understanding of complex processes such as galaxy formation and the behavior of dark matter. Results from universe simulations provide insights that are often unattainable through traditional observational methods, highlighting their fundamental importance in scientific discourse.
The significance of universe simulation lies not only in its practical applications but also in the implications it holds for broader scientific inquiry. As researchers push the boundaries of simulation capabilities, they grapple with significant questions regarding the nature of reality itself. By creating models that mimic the intricate workings of the universe, scientists challenge our perceptions and deepen our understanding of fundamental laws governing the cosmos.
As we proceed to dissect the topic, key elements will emerge. These will encompass a detailed definition of universe simulation, its historical evolution, and the methodologies employed in creating such intricate models. Understanding these facets not only reveals the technical prowess utilized but also emphasizes the evolving landscape of astrophysical research. Ultimately, the exploration of universe simulations aims to inform and provoke thoughtful dialogue among students, researchers, educators, and professionals in various scientific fields.
Defining Universe Simulation
Universe simulation can be defined as a computational method that generates representations of cosmic entities and their interactions based on the principles of physics. These simulations operate by using sophisticated algorithms to solve complex equations that describe the behavior of matter, energy, and the fabric of space-time. By representing different components of the cosmos, including galaxies, stars, and interstellar matter, universe simulations enable scientists to visualize processes and phenomena that span billions of years in a fraction of a second.
The resulting models provide a playground for theorists and observers alike, as simulations can predict outcomes and suggest new avenues for investigation. The interplay of various elements and factors within these models gives rise to deeper insights into gravitational interactions, cosmic expansion, and other critical astrophysical phenomena.
Historical Background
The history of universe simulation is intertwined with the development of computational technology and theoretical astrophysics. In the early days, simulations were rudimentary, primarily using basic numerical methods and limited computing resources. For example, the simulation of celestial motions as far back as the 19th century laid a groundwork for future advancements. However, it was during the latter half of the 20th century that significant strides began to emerge.
The advent of powerful supercomputers in the 1980s revolutionized the field, enabling researchers to simulate more complex systems. Early projects, such as the ones focused on simulating the dynamics of galaxy formation, marked a turning point in the ability to visualize cosmic evolution. As technology advanced, simulations became progressively sophisticated, incorporating elements like dark matter and dark energy into their frameworks. Today, advanced techniques involving grid-based methods and particle simulations, such as those used in the Illustris project and EAGLE simulation, continue to advance our understanding of the universe at colossal scales.
As we delve further into the complexities of universe simulation, a comprehensive examination of its theoretical underpinnings, technological advances, and interdisciplinary applications will unfold, presenting a clearer picture of this captivating field.
Theoretical Underpinnings
The theoretical underpinnings of universe simulation are essential for anyone looking to understand the complexity of cosmic phenomena. This section explores the fundamental concepts and mathematical frameworks that serve as the backbone for simulating the universe. Understanding these theories contributes to the overall goals of the article by providing insight into how simulations replicate and predict astronomical events. Each component discussed here highlights critical aspects that not only enhance the reliability of simulations but also shed light on the intricate nature of our universe.
Fundamental Concepts
Physics of Space-time
The physics of space-time is crucial for universe simulations. It embodies how time and space interact and influence various celestial phenomena. A key characteristic of space-time is its ability to unify spatial dimensions with temporal flow. This model is beneficial because it allows simulations to accurately reflect how gravity affects the motion of celestial bodies within a framework that is consistent with relativity.
A unique feature of space-time in simulations is the implementation of the general relativity equations. These equations improve the accuracy of simulations when predicting the trajectories of objects in space. However, this increased complexity can lead to higher computational demands, making resource allocation a significant consideration.
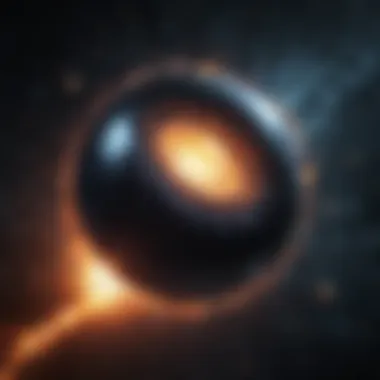
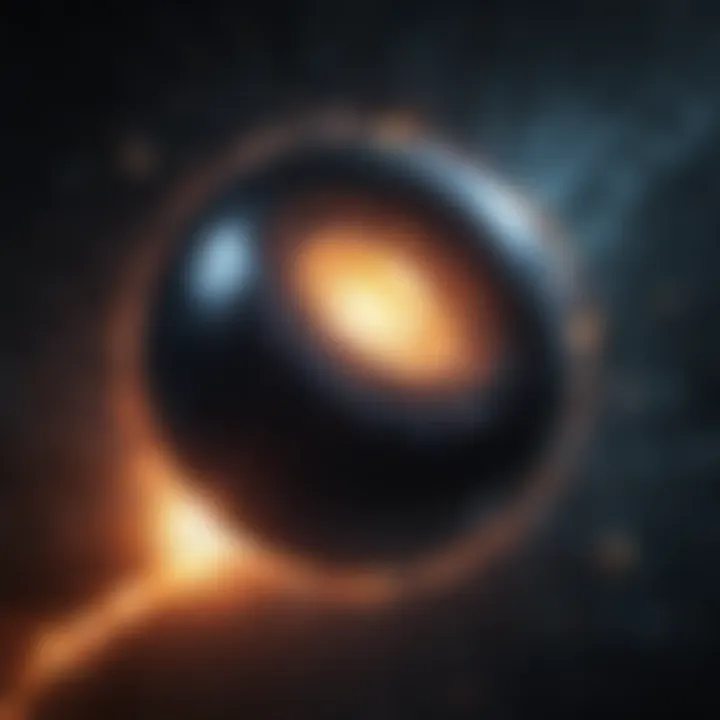
Cosmological Models
Cosmological models, such as the Lambda Cold Dark Matter model, are essential to universe simulations. They offer a systematic approach to understanding the universe's structure and dynamics. The key characteristic of these models is how they incorporate various parameters like dark matter and dark energy, which are integral in understanding cosmic expansion.
In simulations, cosmological models provide a framework that enables the study of large-scale structure formation over time. A unique aspect is their capacity to simulate different scenarios of cosmic evolution. These models, however, can introduce disadvantages, especially when faced with observational data that challenge their assumptions. Therefore, careful consideration is necessary to ensure that models remain a valid representation of physical reality.
Mathematical Frameworks
Mathematical frameworks guide the numerical simulations utilized in universe modeling. This segment focuses on two primary components: numerical methods and algorithm design, both of which are vital for achieving realistic and predictive simulations.
Numerical Methods
Numerical methods allow researchers to make calculations that would otherwise be impossible analytically. These methods can handle complex equations, making them essential in simulating dynamic astrophysical processes. A critical characteristic is their iterative nature, which lends itself well to refining results through repeated applications.
For instance, methods like finite difference and spectral analysis are often employed to approximate solutions of differential equations in simulations. Each method has unique features that offer different advantages; finite difference methods can be simpler to implement, while spectral methods may yield greater accuracy for specific problems. However, the choice of technique can affect the scalability of simulations and the accuracy of results, requiring a nuanced approach for optimal application.
Algorithm Design
Algorithm design underpins the efficiency of universe simulations. It focuses on creating procedures that dictate how simulations process and interpret data. This aspect is significant because a well-designed algorithm can dramatically reduce computational costs while enhancing output speed.
Key characteristics of effective algorithm design include adaptability and precision. An adaptable algorithm can adjust based on various inputs, which is beneficial in an ever-evolving field like astrophysics. A unique feature found in some algorithms is their hybrid nature, blending traditional methods with newer techniques, such as machine learning. While this can enhance simulations, the complexity of these algorithms might introduce challenges in debugging and optimization, necessitating careful development and testing.
Technological Advances in Simulation
The field of universe simulation is heavily influenced by technological advances. These developments enhance our capacity to model cosmic phenomena accurately and efficiently. Specifically, two areas stand out: computational power and software development. These advancements have significant implications for research across various scientific domains.
Computational Power
Supercomputers
Supercomputers are critical in running complex universe simulations. They offer substantial processing power, which is essential for handling large datasets and intricate calculations. This kind of computing device is capable of performing quadrillions of calculations per second, making them an indispensable resource for researchers. The key characteristic of supercomputers is their parallel processing capabilities, enabling simultaneous execution of multiple operations. This leads to faster processing times, which is particularly beneficial for time-sensitive experiments in astrophysics.
A unique feature of supercomputers is their architecture, which often involves thousands of processors working together. One advantage is that they can solve problems that traditional computers cannot manage. However, a disadvantage is their significant cost and energy consumption, which can restrict access for smaller research institutions.
Cloud Computing
Cloud computing offers a flexible alternative to supercomputers, democratizing access to computational resources. This technology allows researchers to utilize powerful servers hosted in data centers instead of needing local hardware. The main benefit is scalability; researchers can adjust their resource usage based on project needs without needing to invest in expensive equipment upfront.
A unique feature of cloud computing is its pay-as-you-go model, which can significantly reduce costs for short-term or experimental projects. While offering almost limitless processing power, cloud computing can still face challenges such as data transfer speed and security concerns.
Software Development
Simulation Packages
Simulation packages play a fundamental role in universe simulation by providing tools for creating models and running simulations. These packages often come with user-friendly interfaces, which make it easier for scientists to visualize complex data. A key feature of simulation packages is their ability to handle various astrophysical processes, enabling researchers to simulate and analyze different phenomena.
One notable advantage is that these packages often include extensive libraries and pre-built models. This can accelerate research and increase productivity. However, customization may be limited, which can restrict advanced users.
Open-source Platforms
Open-source platforms also provide valuable resources for universe simulation. They allow any user to access and modify the underlying code. This characteristic fosters collaboration and innovation among scientists, particularly in niche areas of research. Open-source platforms are beneficial as they promote a spirit of sharing and community.
The unique feature of these platforms is their flexibility, which enables users to tailor the software to meet specific research needs. Although they may lack full technical support, which can be a downside for some users, the cost-effectiveness and adaptability of open-source platforms make them a popular choice for academic institutions.
"Technological advances in simulation create new possibilities for understanding the universe and its complexities."
In summary, the progress in computational power and software development not only enhances the capability of universe simulations but also influences how researchers engage with cosmic data. The evolution of these technologies continues to shape the future of astrophysical research.
Applications of Universe Simulation
The study of universe simulation is not just an academic pursuit; it has practical applications that significantly impact various fields. This section highlights the importance of universe simulation in advancing knowledge, influencing research methodologies, and informing decision-making processes in both scientific and applied contexts. By understanding its applications, we can appreciate how universe simulations enhance our understanding of complex phenomena and drive innovations across disciplines.
Astrophysics Research
Galaxy Formation
Galaxy formation is a crucial aspect of astrophysics research where universe simulations play a vital role. Researchers utilize simulations to model the processes that lead to the formation and evolution of galaxies. The key characteristic of this field is its ability to replicate cosmic structures in a controlled computational environment. This makes it a beneficial choice for understanding the intricacies of how galaxies emerge, evolve, and interact within the cosmos.
One unique feature of galaxy formation simulations is their capacity to incorporate data from various observational sources, such as telescopic surveys. This integration allows scientists to refine their models over time to better reflect actual data. However, accuracy remains a challenge; the simulations require extensive computational resources, which can be a limitation for some research institutions.
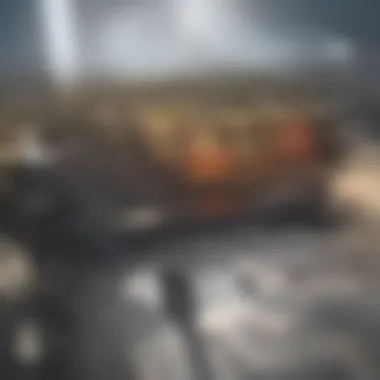
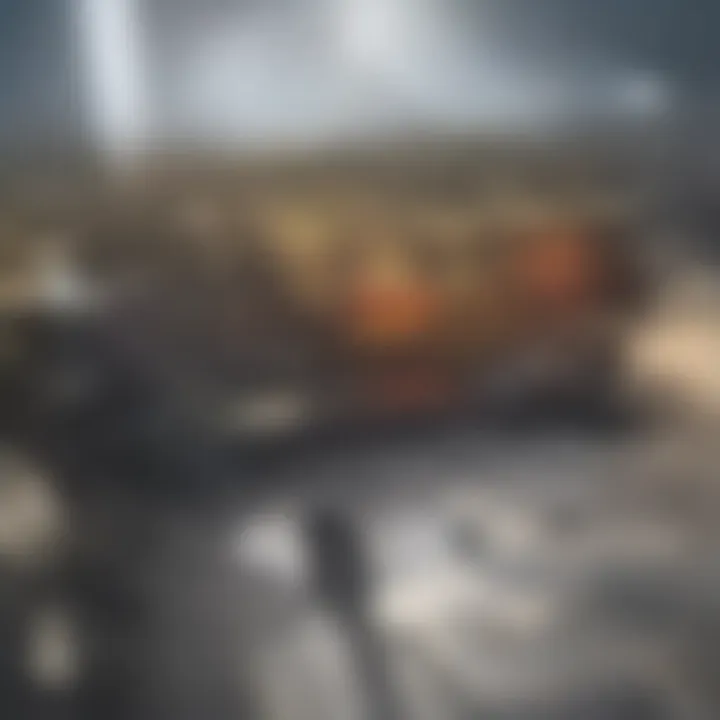
Black Hole Dynamics
Black hole dynamics is another area where universe simulations make significant contributions. These simulations help in studying the gravitational effects and behaviors of black holes under different scenarios. The key characteristic of black hole simulations is their complexity, given the extreme conditions involved. This makes it a popular choice for physicists seeking to understand phenomena such as accretion disks and gravitational waves.
A unique feature of black hole dynamics simulations is their ability to predict the outcomes of black hole mergers. This can provide insights into the nature of gravity and spacetime. Still, one disadvantage is that the high-energy scale involved often leads to computational challenges, requiring sophisticated techniques to manage the simulations effectively.
Cosmological Studies
Expansion of the Universe
The expansion of the universe is central to cosmology. Simulations allow scientists to explore this phenomenon in depth, offering crucial insights into the universe's growth. The key characteristic of this topic is its focus on large-scale structures and the laws governing cosmic expansion. This is a beneficial aspect of universe simulation as it helps refine cosmic models based on observational data.
One unique feature of researching the expansion of the universe is its integration with the study of cosmic microwave background radiation. Such simulations yield significant data about the energy density of the universe, although they can be computationally intensive, often requiring high-performance computing resources.
Dark Matter and Dark Energy
Dark matter and dark energy are two critical concepts in modern cosmology, significantly influencing the structure and fate of the universe. Universe simulations help visualize and theorize about their effects. The key characteristic here is that these simulations can model the mysterious components that make up a substantial part of the universe. This makes it a popular choice for investigations aiming to unravel the mysteries of dark matter and dark energy's roles.
A unique feature of simulating dark matter and dark energy is their ability to create different models examining how these substances interact with visible matter. However, a major challenge is that our understanding of their properties is still incomplete, leading to potential inaccuracies in the models used for simulations.
Interdisciplinary Impact
Environmental Simulations
Universe simulations extend beyond astrophysics and cosmology, impacting environmental sciences too. They enable scientists to model complex ecological systems and their interactions with cosmic phenomena. The key characteristic of environmental simulations is their ability to predict outcomes based on variations in environmental parameters. This is a beneficial application highlighting the interconnectedness of cosmic and terrestrial processes.
A notable unique feature of these simulations is their scalability for various applications, from climate modeling to resource management. However, the intricate nature of environmental systems can complicate simulations, yielding questions about accuracy and applicability in real-world scenarios.
Engineering Applications
In engineering, universe simulations enable the analysis of dynamic systems, particularly in aerospace and mechanical fields. They can model the behavior of materials and structures under different conditions. The key characteristic of engineering applications of simulations is their focus on precision and optimization. This makes it a beneficial choice for designing safer and more efficient structures.
The unique feature of these simulations is their capacity to analyze real-time data, making them adaptable for testing various engineering challenges. Nonetheless, the dependence on accurate initial conditions can introduce uncertainties, which may affect the reliability of the results.
Ethical and Philosophical Considerations
The exploration of universe simulation inevitably raises numerous ethical and philosophical questions. These considerations are vital for understanding the implications of creating detailed models of the cosmos. The ethical frameworks surrounding simulation encourage researchers to reflect critically on their work. This is not merely a mathematical endeavor; it is a construction of a potential reality that can shape both scientific advancements and societal perspectives.
The Ethics of Simulation
Manipulation of Data
Manipulating data within universe simulations serves as a double-edged sword. On one hand, it allows scientists to tweak parameters and enhance the fidelity of models. This can lead to profound insights into cosmic phenomena. However, it also poses a risk. Data manipulation can lead to misinterpretations or even deceptive results. This becomes a significant concern when findings influence public perception or policy decisions. A key characteristic of this manipulation is the delicate balance between necessary adjustments and potential overstepping. While it can improve simulations, it may also introduce biases and distortions. Thus, careful consideration of ethical guidelines in data manipulation is essential to maintain the integrity of research.
Implications of Virtual Universes
The implications of virtual universes stretch beyond academic circles into the realms of philosophy and ethics. Creating these universes raises questions about the nature of existence and reality itself. Virtual environments allow simulations to explore the laws of physics in ways that are unattainable in the real world. This capacity highlights a key characteristic: the ability to test theories without physical constraints. However, the benefits of creating such models must be weighed against the potential for misuse. The findings from these simulations may lead to generalized misconceptions about reality or foster a detachment from scientific evidence. Thus, it is crucial to engage in responsible practices regarding the meaning and implications of these virtual representations.
Philosophical Implications
Nature of Reality
The exploration of the nature of reality through universe simulations opens an intriguing dialogue. With the help of simulation, we can assess complex questions about what is real. This exploration highlights how our perceptions can be influenced by simulations that mirror or diverge from our understanding of the universe. The significant characteristic of these discussions is the challenge they pose to traditional views. By questioning realityβs fabric, we engage with a concept that is both enriching and unsettling. The advantages of this line of inquiry include a deeper appreciation for the intricacies of the cosmos. However, it may also instigate discomfort or confusion about our place in the universe, which makes philosophical engagement crucial.
Human Understanding of the Universe
Human understanding of the universe is deeply intertwined with universe simulation. Simulations present opportunities for learning and refinement of theories. They serve as a prism through which researchers can view astronomical phenomena. A unique feature of this understanding is its dynamic nature; as simulations evolve, so too does our grasp of the universe's complexities. This ongoing growth represents a substantial advantage, fostering scientific progress. Yet, it may also inspire overconfidence in our knowledge, leading to dogmatic beliefs about scientific truths. Therefore, maintaining a critical perspective on how simulations shape our understanding is essential.
"In the quest for knowledge, humility must accompany our discoveries; for every answer brings forth new questions."
Engaging ethically and philosophically with universe simulation enriches our comprehension and appreciation of the cosmos. While we delve into the science, it is equally important to remain cognizant of the broader implications of our explorations.
Challenges in Universe Simulation
Universe simulation presents a set of notable challenges that influence how researchers understand cosmic phenomena. Recognizing these challenges is critical in identifying the limitations and potential shortcomings of existing models and technologies. It becomes essential to contemplate both computational and accuracy-related hurdles when enhancing the realism and reliability of simulations. This section delves into the core issues of computational limitations and accuracy, as they dictate the trajectory of research in this dynamic field.
Computational Limitations
Resource Allocation
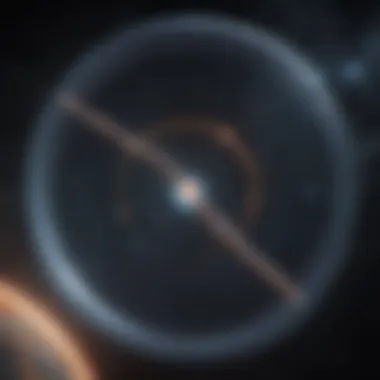
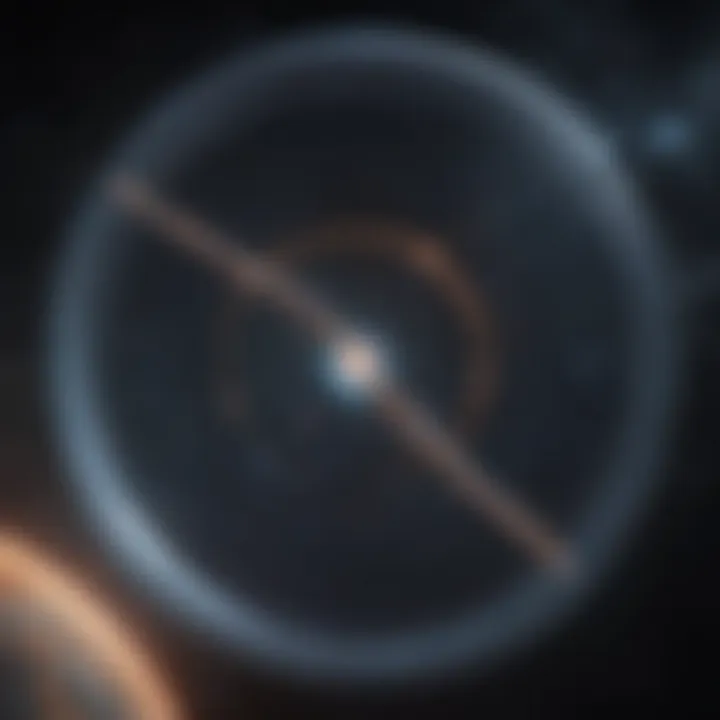
Resource allocation is a crucial aspect in universe simulation. It relates to the distribution of computational resources, such as processing power and memory, during simulation runs. Efficient resource allocation contributes to improved performance in simulations, enabling better handling of complex calculations that are nature of cosmic modeling. A key characteristic of this aspect is its impact on not just speed, but on the quality of the output as well.
One might explore the uniqueness of resource allocation strategies by looking at adaptive techniques that dynamically adjust resource usage based on simulation needs. These techniques present several advantages. They allow for optimized performance and enable simulations to run longer and more accurately. However, they can also introduce disadvantages, such as increased complexity in implementation and potential waste of resources if not managed correctly.
Scalability Issues
Scalability issues focus on the ability of simulation models to grow in complexity without a loss in performance. As scientific questions evolve, simulations often require enhancements, like higher resolution or longer duration. This directly ties to the notion of scalability, impacting how effectively a model can evolve with ongoing research. A central aspect of scalability is its influence on the duration and cost of simulations, making it a necessary consideration for researchers and institutions.
Unique features of scalability include parallel processing and cloud computing capabilities that allow researchers to access additional power as needed. Advantages of scalability are clear; models can accommodate larger datasets leading to more accurate results. Nonetheless, challenges remain. Disadvantages include potential bottlenecks in processing due to increased data workload or high dependencies among various computational tasks, which can complicate overall execution.
Accuracy of Simulations
Model Validation
Model validation serves as a bedrock for verifying the reliability of simulations concerning real-world observations. It involves comparing the outcomes of simulations with actual astronomical data. An important aspect of model validation is its reliance on observational evidence, which helps in fine-tuning models to better reflect reality. A key characteristic is the iterative nature of validationβmodels may require continual adjustments based on new data findings.
The uniqueness of model validation lies in its dual function: not only does it ensure accuracy, but it also fosters confidence in the models used. Advantages include establishing credible predictions that researchers can trust. On the flip side, disadvantages can arise when validation is limited by the availability of accurate observational data, leading to uncertainties in the models being utilized.
Error Propagation
Error propagation refers to the cumulative effect of errors in input data on the outcomes of simulations. This is of particular importance because even small inaccuracies can amplify significantly, skewing results. A key characteristic of error propagation is its relationship with the initial inputs of the simulation, as errors in these inputs may lead to substantial deviations in model outputs.
The unique feature of error propagation is its capacity to illuminate how errors at various stages affect the overall results. This provides insight for researchers on potential weak points in their simulations. Advantages of addressing error propagation include enhanced accuracy and robustness of simulations. However, the disadvantages include increased complexity in managing and mitigating these errors effectively, which can consume resources and time that would otherwise be applied elsewhere in the research process.
"Understanding these challenges allows researchers to refine their approaches and push the boundaries of what universe simulations can achieve."
In summary, addressing computational limitations and ensuring accuracy through rigorous check-points are crucial in improving universe simulations. These challenges demand constant attention as technology and methodologies evolve, thereby shaping the future of astrophysical research.
Future Directions and Innovations
Future directions in universe simulation are critical as they shape not only the methodologies used in narrow scientific scopes but also form the foundation for broad interdisciplinary applications. Understanding the trajectory of these innovations can amplify our ability to respond to complex cosmological questions and enhance our computational capabilities. These developments often pave the way for breakthroughs that redefine our understanding of the universe.
Emerging Technologies
Quantum Computing
Quantum computing represents a significant leap in computational theory and practice, particularly for tasks associated with universe simulation. It can process vast amounts of data with unprecedented speed due to its unique characteristic of superposition, allowing simultaneous calculations on multiple states. This capability makes quantum computing a popular choice for simulations that require high precision and extensive resource allocation.
A defining feature of quantum computing is entanglement, which enables complex problem-solving that classical computers struggle with. In the context of universe simulation, this could mean more accurate modeling of cosmic events, such as the interactions between particles at quantum levels, which are crucial for understanding phenomena like black holes or dark matter.
However, while quantum computing presents many advantages, such as increased processing power, it also faces challenges like error rates and the need for quantum error correction. Navigating these limitations is crucial for scientists aiming to implement quantum computing in universe simulations effectively.
AI in Simulations
Artificial intelligence (AI) is revolutionizing how simulations are conducted and interpreted. In universe simulations, AI contributes by automating complex tasks, enhancing model predictions, and improving data analysis efficiency. The key characteristic of AI is its ability to learn from incoming data and adapt, making it a powerful tool for predictive modeling and real-time analysis of cosmic events.
One unique feature of AI in simulations is its capability to process unstructured data, such as images from telescopes or even large datasets from experiments. This enables researchers to identify patterns and anomalies that might be invisible to human analysts. The implementation of AI can thus lead to richer insights into formation of galaxies or the behavior of celestial bodies.
The advantages of using AI include increased accuracy and reduced time for simulations. However, it is essential to remain cautious about the interpretability of AI models. As algorithms can sometimes yield results that are difficult to explain, this could lead to debates about validity and trust in simulative outputs.
Evolving Research Landscapes
The landscape of research in universe simulation is continuously evolving, driven by both technological advancements and interdisciplinary collaborations. Researchers are increasingly integrating knowledge from varied fields such as physics, computer science, and even social sciences to enhance their simulations. This multidisciplinary approach helps in constructing more holistic models that account for various factors influencing cosmic events.
Furthermore, as new technologies emerge, the ability to address complex cosmological questions increases significantly. This shift towards more integrated studies allows for richer, more informative simulations that can offer insights not only into astrophysics but also into related fields, ultimately benefiting the broader scientific community.
"The future of universe simulation lies not only in better technology but in the collaboration of diverse disciplines that enrich our understanding and application of these tools."
Each of these elements highlights the critical need for continuous innovation in the domain of universe simulation. As we stand at the cusp of new scientific horizons, recognizing and integrating these advancements will be fundamental for those engaged in this intricate field.
Culmination
The conclusion of this article serves as a pivotal element in understanding the intricate domain of universe simulation. It crystallizes the key insights acquired throughout the exploration, emphasizing the multifaceted contributions this field makes to science and philosophy. By summarizing the fundamental aspects discussed, the conclusion reinforces the relevance of universe simulations in contemporary research and thought.
Summarizing Key Insights
A variety of key points emerge from our comprehensive examination of universe simulation. We have explored its theoretical foundations, which lay the groundwork for accurately modeling cosmic phenomena. Historical context revealed how simulation has progressed, from rudimentary models to sophisticated systems powered by advanced computational techniques. Technological advancements such as supercomputers and AI demonstrate the increasing ability to perform complex simulations and tap into previously uncharted areas of astrophysics.
Moreover, the applications of universe simulations extend beyond astrophysics. Impacts on cosmology, engineering, and environmental science shed light on the profound connections between different scientific disciplines. Ethical and philosophical considerations are notable, challenging traditional notions of reality and the implications of constructing virtual universes. It is essential to be aware of these factors as they shape not only research methodologies but also societal perspectives.
The Continuous Journey of Discovery
As we conclude this exploration, it is vital to recognize that the journey of discovery within universe simulation is far from over. Emerging technologies, particularly quantum computing, hold the promise to revolutionize simulation capabilities. The artificial intelligence tools can enhance the accuracy and efficiency of models, opening up new avenues of inquiry.
The ongoing evolution of research landscapes in this field ensures that questions will continue to arise. How do these simulations affect our understanding of cosmic events? What new insights might they uncover about the fundamental nature of the universe? Such inquiries drive scientists, researchers, and educators alike to deepen their engagement with simulations.