Understanding Spacetime: Concepts and Implications
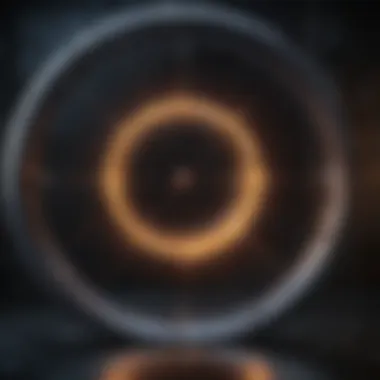
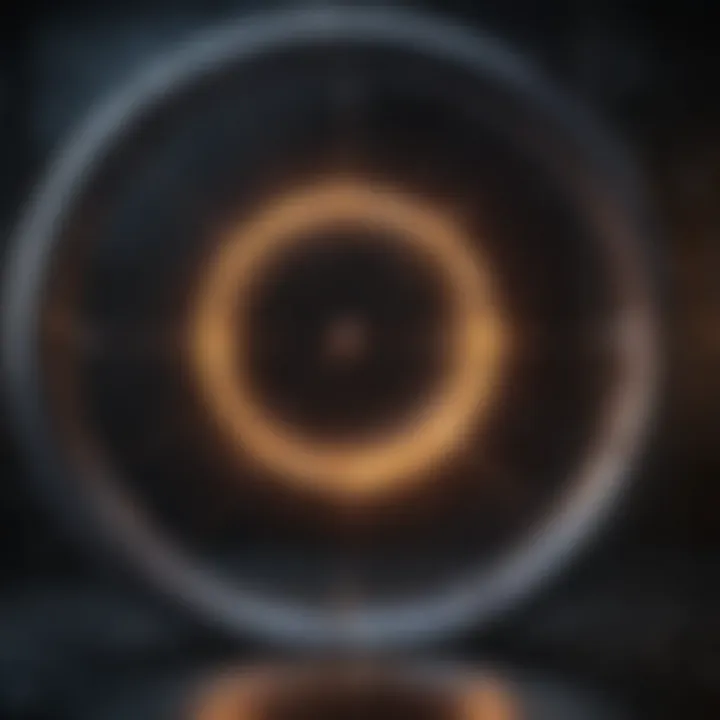
Intro
The intersection of space and time, a sphere often fraught with paradoxes and peculiarities, forms the bedrock of modern physics. Spacetime isn’t just a cool concept to throw around at cocktail parties; it's an essential framework that challenges our intuitive grasp of reality. Imagine trying to visualize a universe where the events unfold not just on a two-dimensional plane but are inherently intertwined with the passage of time. Understanding this fusion is pivotal to grasping everything from how galaxies collide to the very fabric of our existence.
At a glance, spacetime might seem like a theoretical playground reserved for scientists and mathematicians, but its implications reverberate through our daily lives. From the GPS systems that dictate our travel routes to the predictions of black holes’ behavior, spacetime is not merely abstract—it's incredibly practical. Through this article, we'll dissect its concepts, trace historical developments, and illuminate its significance.
As we marvel at the cosmos, we will venture from the dusty corners of classroom lectures to the forefront of innovative research, shedding light on the phenomena that science continues to unravel. Buckle up, as we embark on this journey through the vastness of spacetime.
Prologue to Spacetime
Understanding spacetime is no small feat—it’s the bedrock of modern physics, intertwining our grasp of both space and time into a seamless fabric. The significance of this topic cannot be overstated, as it has far-reaching implications in how we comprehend the universe and its operations. For students, researchers, and other inquisitive minds, delving into spacetime opens doors to concepts that reshape our understanding of gravity, motion, and the very essence of existence.
At its core, spacetime is more than just a fancy term. In practical terms, it influences various fields, from theoretical physics to cosmology. Every time we consider how objects move or how time flows, we are, in essence, engaging with spacetime principles. This article aims to navigate through these intricate notions, providing clarity and insight to the readers.
Key points to consider as we dive deeper into this exploration include:
- Defining Spacetime: Understanding the foundations upon which our concepts of space and time rest.
- Historical Context: Tracing the evolution from Newton's framework to Einstein's revolutionary ideas.
- Philosophical Implications: Considering how these scientific advancements influence our worldview and philosophical beliefs.
Without a doubt, this introduction serves as a springboard for more extensive exploration into spacetime, allowing for a comprehensive understanding of its concepts and real-world implications.
Defining Spacetime
Spacetime merges the three dimensions of space with time to form a coherent four-dimensional continuum. Rather than treating time and space as separate entities, the concept invites us to consider them as interconnected features of the universe. Each point in spacetime is characterized not only by where it is located in space but also by when it occurs.
This integration challenges the classical notions of both time and space, as it introduces complexities such as time dilation, where time behaves differently under varying conditions of speed and gravity. This phenomenon doesn’t just remain confined to theoretical discussions; it has practical applications in technologies like GPS.
Historical Context
Newtonian Framework
The Newtonian framework laid the groundwork for understanding motion and gravity. Sir Isaac Newton posited that space was absolute, serving as a stage where events unfold, while time flowed uniformly. This model played a crucial role in advancing physics for centuries.
- Key characteristic: Newton’s laws of motion remain integral to classical mechanics and engineering.
- Unique feature: The straightforward nature of Newtonian physics made it accessible, facilitating early understanding.
- Advantages/Disadvantages: While beneficial for practical calculations, it lacked the nuance to explain phenomena at very high speeds or in strong gravitational fields. Thus, it was eventually seen as limited in scope.
Einstein's Revolution
The transition from Newtonian concepts to Einstein’s ideas marked a pivotal turn in the scientific narrative. Einstein’s theory of relativity brought forth the notion that space and time could bend and curve in response to mass—an idea that reshaped the landscape of physics entirely.
- Key characteristic: The interdependence of space and time under gravitational effects created a new paradigm.
- Unique feature: This concept offered a mathematical framework that explained the behavior of celestial bodies far more accurately than Newtonian physics ever could.
- Advantages/Disadvantages: Though it provided deeper insights, it also added layers of complexity that required advanced math, potentially alienating those not steeped in scientific education.
Philosophical Implications
The interplay of spacetime concepts carries substantive philosophical implications. It challenges our intuitive perceptions of reality, urging us to reconsider our understanding of existence, causality, and the very fabric of the universe.
In grappling with these ideas, we confront questions that have lingered throughout human history: What is the nature of reality? How do we define existence beyond the immediate sight and experience? The evolution of thought about spacetime not only enriches scientific discourse but also invites a reflective consideration of our place within the cosmos.
This intricate dance of ideas highlights the necessity of merging scientific and philosophical perspectives, guiding us toward a holistic understanding of spacetime and its profound implications.
Mathematical Foundations of Spacetime
Mathematical foundations serve as the backbone of our understanding of spacetime. Without the precision of mathematics, concepts in physics would remain abstract and unquantifiable. It is through mathematical formulations that we can express the complexities of spacetime, facilitating clearer communication among scientists and enhancing our comprehension of the universe.
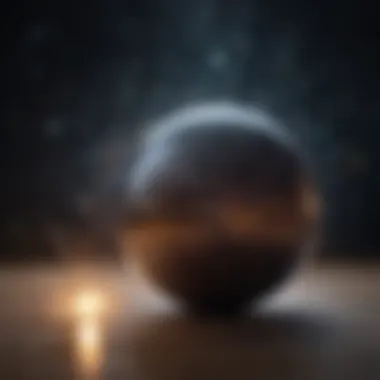
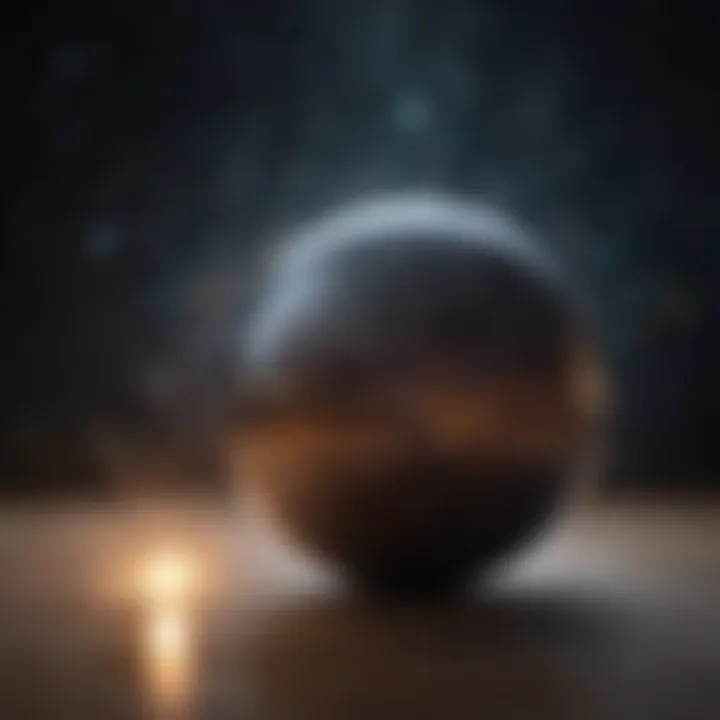
At the heart of this foundation lies the ability to analyze and represent the intricate relationships between space and time. By employing a variety of mathematical tools, researchers can develop models that encapsulate phenomena that were once considered mysterious. Considerations surrounding curvature, distance, and motion gain a form of clarity through these mathematical lenses.
Coordinate Systems
Coordinate systems are the frameworks that allow us to describe the location of points in space and time. Imagine trying to navigate a vast, unfamiliar landscape without a map—coordinate systems act much like that map, providing essential references.
In the context of spacetime, we often work with different coordinate systems, including Cartesian, polar, and more complex curvilinear setups. Each system has its own advantages:
- Cartesian coordinates are straightforward, using x, y, and z to pinpoint locations in three-dimensional space.
- Polar coordinates are particularly useful when dealing with circular or spherical objects, emphasizing radial distances and angles.
- Generalized coordinates come into play for more complex systems where traditional approaches become cumbersome.
The choice of a coordinate system impacts how we understand motion and forces. For example, in the study of General Relativity, spacetime is often described using a curved coordinate system that reflects the influence of gravity on the fabric of spacetime. This curvature signifies how mass and energy warp the geometry around them, influencing the path of objects.
Metric Tensor
The metric tensor is an essential construct that allows us to measure distances and angles in spacetime. It’s like a ruler but for the four-dimensional interplay of space and time.
Mathematically, the metric tensor is a symmetric second-rank tensor that captures the geometry of spacetime. It defines how to measure distances between nearby points. For instance, the way we come to terms with the curvature of space around a massive object like the Earth can be understood through the metric tensor. Specifically, in General Relativity, the metric tensor is central to Einstein’s field equations, serving as a bridge between the geometry of spacetime and gravitational fields.
"The metric tensor illuminates the path on which light travels and how objects move in the gravitational tapestry of the universe."
By analyzing the properties of the metric tensor, scientists can characterize different spacetime geometries and their implications. Understanding this tensor helps unravel themes of gravitational waves and the bending of light around massive objects, demonstrating the interconnectedness of geometry and physical phenomena.
Spacetime Diagrams
Spacetime diagrams provide a visual representation of events in the context of spacetime. They illustrate how time behaves differently depending on the observer's frame of reference. Picture this: two observers moving at different speeds view the same event, yet their interpretations may vary significantly. Spacetime diagrams help clarify these phenomena.
In essence, these diagrams use a two-dimensional axis, with one axis representing time and the other representing space. By plotting events along these axes, we can visualize complex ideas such as:
- Light cones, which demonstrate the limits of how information travels through spacetime.
- World lines, which visually depict the path of an object moving through spacetime.
- Simultaneity, showing how two events viewed by different observers may not occur at the same time.
These diagrams are pivotal in exploring concepts like time dilation and length contraction, illustrating how intertwined our understanding of time and space really is.
The mathematical foundations of spacetime ultimately pave the way for deeper explorations into the nature of the universe. With reference to the tools and ideas discussed here, we can begin to peel back the layers of complexity and enjoy a clearer view of the cosmos.
The Role of Spacetime in Physics
Spacetime is not merely an abstract concept but serves as the backbone of modern physics. Understanding spacetime allows us to fully grasp the forces shaping our universe, from the motion of planets to the very fabric of reality. Spacetime intertwines the three dimensions of space with time, offering a unified framework to analyze complex phenomena. By recognizing how objects behave in this intertwined dimension, physicists can predict outcomes with surprising accuracy, thus underlining the importance of spacetime in both theoretical and practical applications.
General Relativity
Gravity and Curvature
The relationship between gravity and curvature is a fundamental aspect that reveals the elegance of general relativity. Gravity isn't just a force drawing objects together; it stems from the curvature of spacetime itself. This curvature is caused by mass, meaning that large objects like stars and planets warp the spacetime around them, influencing the motion of other objects nearby.
This offers a key characteristic of gravity: it is not merely a push or pull but an effect of spacetime's geometry. This insight is a beneficial choice in this discussion as it transforms our understanding of gravity from a simplistic viewpoint to an intricate cosmic dance involving geometry.
One unique feature of this concept is its predictive power. For instance, the famous test of light bending around massive bodies during a solar eclipse confirmed Einstein's prediction and has practical implications in technologies like GPS, which must account for these effects. However, this complexity can make general relativity challenging to teach and understand. Traditional intuitions about forces can lead to misconceptions if one does not grasp the geometric nature of gravity.
Black Holes
Black holes present another fascinating aspect of spacetime in physics. They represent regions where spacetime curvature becomes so extreme that nothing, not even light, can escape their grasp. This characteristic makes black holes a popular topic for exploration in both theoretical and observational physics. Their existence leads to profound implications about the nature of reality, including concepts like event horizons and singularity.
The unique feature of black holes is their relatable yet mind-bending quality: they challenge the boundaries of current physical theories, merging quantum mechanics and general relativity. This merging is a double-edged sword; it sparks excitement and inquiry but also illustrates the limitations of our current understanding, highlighting major questions in physics yet to be resolved.
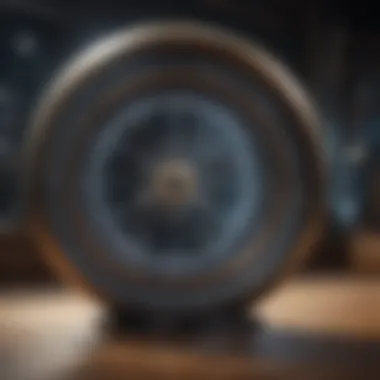
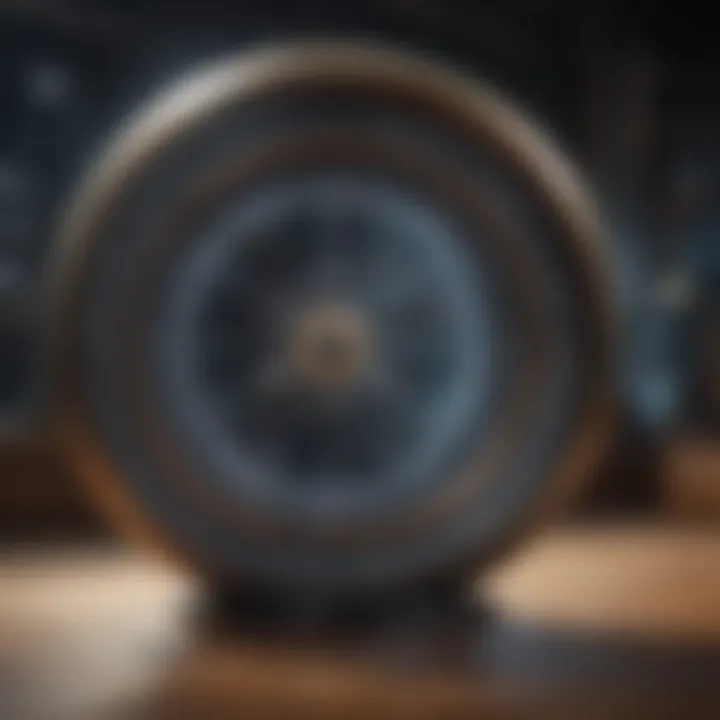
Quantum Mechanics and Spacetime
Entanglement
Entanglement stands out as one of the most intriguing aspects of quantum mechanics. It describes a scenario where particles become intertwined in such a way that the state of one instantaneously influences the other, regardless of distance. This challenge to classical notions of locality has profound implications, intertwined with spacetime dynamics.
The key characteristic of entanglement is its instantaneous nature, prompting discussions about causality and the fabric of spacetime itself. This makes entanglement a beneficial element for further explorations in our understanding of spacetime, suggesting a depth to reality that transcends classical physics.
Entanglement reveals a unique feature where information seems to travel faster than light, favoring new theories about spacetime. However, its enigmatic nature raises many questions. How information is transmitted and whether it adheres to the principles of spacetime fuel ongoing debates and experiments in both physics and philosophy.
Particle-Wave Duality
Particle-wave duality encapsulates another dimension of quantum mechanics and its interaction with spacetime. It fundamentally states that every particle or quantum entity can be described as both a particle and a wave. This notion plays into our understanding of spacetime as it stresses that energy and matter are not distinct entities but two expressions of the same phenomenon.
A key characteristic of this duality is its revolutionary impact on physics, demonstrating that what we once understood as clear boundaries have blurred significantly. This duality is a beneficial concept for understanding how spacetime operates, as it provides insights into the probabilistic nature of the universe, challenging deterministic views engendered by classical mechanics.
This perspective enforces a unique feature—understanding that light, for example, behaves differently depending on the context it is observed—underlines the vast implications of spacetime. Yet, it also generates confusion and debate about the fundamental nature of reality, particularly concerning measurement and observation.
Current Theories and Research
String Theory
String theory presents a compelling approach to understanding spacetime by proposing that the fundamental building blocks of the universe are not point particles but rather tiny strings vibrating at different frequencies. This radical idea has significant implications, suggesting that spacetime could be more intricate than currently perceived.
Its key characteristic lies in its unifying capacity; offering potential pathways to merging general relativity and quantum mechanics presents it as a beneficial topic in contemporary physics discussions. This unification could lead to a more holistic perspective of the universe and the forces interacting within it.
A unique feature of string theory is its reliance on additional spatial dimensions, beyond the familiar four of spacetime. This opens avenues for exploring concepts like multiverses but also draws criticism for not yet yielding empirical results that can be tested. Skepticism towards these extra dimensions showcases the ongoing debates within theoretical physics.
Loop Quantum Gravity
Loop quantum gravity serves as an alternative approach attempting to unify general relativity with quantum mechanics without resorting to additional dimensions. It posits that spacetime itself has a granular structure, fundamentally composed of discrete loops. This theory's distinctiveness lies in its inclination to uphold principles from both realms of physics, offering a unique perspective on gravity and spacetime.
A key characteristic of loop quantum gravity is its mathematical rigor. By presenting a beneficial framework for understanding black holes and the early universe, it helps bridge gaps between the macroscopic and microscopic realms of physics. Loop quantum gravity's unique feature—its integration into existing formulations without requiring drastic changes—makes it a significant player in theoretical physics.
However, the lack of experimental verification presents a drawback. Therefore, while it eases alignment within established theories, it must prove its worth against measurable phenomena to gain widespread acceptance in the scientific community.
Spacetime and Cosmology
Understanding the intricate bond between spacetime and cosmology is crucial in grasping how the universe operates. Spacetime offers a framework within which cosmic events unfold, shaping our perception of reality. When discussing cosmology, we delve into how the universe's structure and history connect with the four-dimensional spacetime continuum.
The Expanding Universe
Big Bang Theory
The Big Bang Theory stands as one of the pillars of modern cosmology, providing a framework for understanding the beginnings of our universe. This theory posits that the universe expanded from an extremely hot and dense state approximately 13.8 billion years ago. One of its key characteristics is the observable redshift of galaxies, indicating that the universe is still expanding today. This observation is not just a stroke of luck— it fundamentally changes our conception of both space and time, highlighting how the cosmos we see now is a young and dynamic entity.
Its popularity stems from its robust predictive power. The theory can accurately explain a plethora of phenomena, including the cosmic microwave background radiation, a remnant from the early universe. However, the Big Bang theory is not without its limitations, such as the inability to explain the conditions preceding the Big Bang effectively.
Among its unique features, the theory describes how all matter and energy were once condensed into an infinitesimal point called a singularity. This aspect remains a mystery and presents both advantages and challenges for physicists trying to uncover what came before this explosive event.
Dark Energy
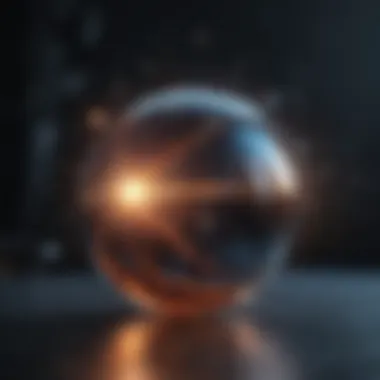
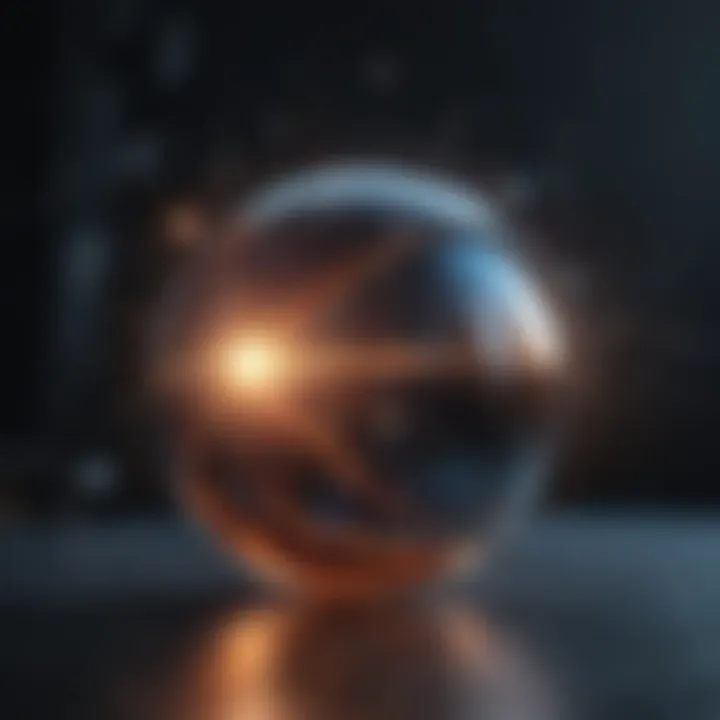
Dark Energy contributes significantly to our understanding of the universe's accelerated expansion. It is a mysterious energy that permeates space, driving galaxies apart at an ever-accelerating rate. A notable aspect of dark energy is that it comprises about 68% of the universe, making it an indispensable topic in modern cosmology. Its nature remains elusive, often described through a term referred to as the cosmological constant.
The implications of dark energy are wide-reaching. It forces us to reconsider long-held beliefs about cosmic dynamics and the fate of the universe. As a popular topic, it invites debate and further investigation; however, its abstract nature poses disadvantages as well. For instance, seeking a deeper understanding of dark energy requires advanced theoretical physics and complex mathematics, making its investigation a challenging endeavor.
The Fate of the Universe
Heat Death
The concept of Heat Death is a striking outcome of the Second Law of Thermodynamics. It suggests that the universe will eventually reach a state of maximum entropy where all energy becomes uniformly distributed and no work can be done. Its key characteristic is the inevitable cooling of matter as stars exhaust their fuel and galaxies drift apart. This concept serves as a crucial hypothetical ending to the lifespan of the universe, illustrating how all things may gradually fade into an endless void of uniformity.
Heat Death presents a pragmatic viewpoint, allowing scholars to explore the long-term outcomes of cosmic evolution. However, this theory also raises philosophical questions about the eventual futility of existence itself, making it a nuanced discussion point in cosmological studies.
Big Crunch
The Big Crunch offers a stark contrast to Heat Death, proposing that the universe might one day cease expanding and begin to collapse back into a singular state. This scenario is predicated on the idea that enough matter exists to counteract the expansion, eventually pulling everything back together in a catastrophic singularity.
One of the intriguing characteristics of the Big Crunch is its cyclical nature, suggesting the possibility of numerous universe generations—expanding, contracting, and repeating. This cyclical concept makes it notably engaging for various philosophical and theoretical discussions. Conversely, the Big Crunch is less favored in light of current observational data indicating an accelerating expansion of the universe. Such discrepancies illustrate its status as a theoretical possibility rather than a foregone conclusion.
"The universe may be but a temporary stage, an act in a grand play yet to unfold, driven by forces we are still striving to understand needlessly."
The narrative of spacetime and cosmology is not just about the stars and galaxies; it is about understanding our place in an ever-evolving cosmos. Each of these theories sheds light on humanity's questions regarding existence, expansion, and ultimate fate. In examining these aspects, we further grasp the complexities of spacetime, redefining our understanding of the universe and our role within it.
Practical Implications of Spacetime Concepts
Spacetime is not just an abstract framework for theoretical physics; it has real-world applications that influence modern technology and our understanding of the universe. By comprehending the nuances of spacetime, we gain insights into how various systems operate, how they can be improved, and what they reveal about the cosmos. This section highlights key practical implications, emphasizing why a solid grasp of spacetime is fundamental in several fields, especially technology and astrophysics.
GPS Technology
Global Positioning System (GPS) technology stands as a prime example of spacetime's practical application. Initially, it may seem like navigating from point A to point B has little to do with the measuring of time and space, but the opposite is true. GPS relies on an intricate interplay of satellites orbiting Earth that transmit signals to receivers on the ground.
To determine your location accurately, the system depends on precise timing, grounded in Einstein's theories of relativity. Here's how it works:
- Satellites: GPS satellites are at an altitude of approximately 20,200 kilometers. Their clocks tick differently due to gravitational effects; they run faster than those on earth.
- Time Synchronization: The technology must consider this time dilation to synchronize signals accurately. If this adjustment is not made, the GPS inaccuracies can lead to navigational errors of several kilometers, illustrating the real need to accommodate spacetime concepts in scientific and logistical frameworks.
- Calculation of Position: GPS calculates your position by triangulating signals from multiple satellites, which inherently involves calculating distances—not just in space, but also in time, making spacetime essential.
In summary, GPS technology highlights how our understanding of spacetime impacts daily life. Mismanagement of time perceptions can lead to significant errors in navigation, showcasing the practicality of these complex theoretical concepts.
Gravitational Waves
Another profound implication of spacetime is found in the study of gravitational waves. These ripples in spacetime produce from massive cosmic events, like the collision of black holes or neutron stars. The detection of these waves was a watershed moment for physics, aligning with predictions made over a century ago by Einstein.
- Detection: Instruments like LIGO (Laser Interferometer Gravitational-Wave Observatory) detect tiny deviations in distances caused by passing gravitational waves. These changes are minuscule, much smaller than the width of a human hair, proving the complexity and the subtlety of spacetime.
- Insights into the Universe: By studying gravitational waves, scientists can learn about the nature of the universe, giving unprecedented insights into phenomena that cannot be observed through traditional electromagnetic waves like light. This has broaden the understanding of stellar evolution and the behavior of extreme gravitational fields.
- Future Research: This area of research opens new doors for questions about the early cosmos, black holes, and dark matter. Each discovery keeps prompting scientists to refine their understanding of spacetime, proving this concept’s growing relevance.
"In many ways, spacetime is the stage upon which the universe plays its drama. Understanding it allows us to interpret the movements and interactions of all entities within it."
Finale
The concluding section of this article holds significance as it encapsulates the essence of spacetime as both a concept and an object of ongoing research. Understanding spacetime hinges on a multi-dimensional approach that informs us about the very fabric of our universe. This is not merely about delineating space from time. Instead, it's about appreciating how these two forces intertwine to shape our reality.
Summary of Key Points
- Spacetime Definition: The article laid foundations on defining spacetime as a four-dimensional continuum where time is integrated with three spatial dimensions.
- Historical Context: It touched on how the understanding of spacetime evolved from Newton’s absolute time and space to Einstein’s revolutionary insights.
- Mathematical and Practical Implications: The mathematical frameworks like the metric tensor and applications in technologies such as GPS demonstrate the power of spacetime concepts in practical scenarios.
- Theoretical Context: Insights into cosmology, black holes, and the implications for quantum mechanics further highlight spacetime's critical role across different physical theories.
- Future Prospects: The significance of advanced research avenues such as string theory underscores the ongoing exploration that challenges existing paradigms and prompts us to consider new theoretical landscapes.
The Future of Spacetime Research
As we look ahead, the study of spacetime is positioned at the frontier of modern physics. Noteworthy areas of future inquiry include:
- Higgs Boson and Field Theory: The relationship between Higgs dynamics and spacetime structure could provide insights into how mass influences spacetime curvature.
- Quantum Gravity: A push towards a unified theory that seamlessly merges general relativity with quantum mechanics might revolutionize our comprehension of the universe.
- Multi-dimensional Models: Exploring additional dimensions within string theory or other frameworks could expose new properties of spacetime not yet understood.
- Experimentation and Observation: Advancements in observational technology—like gravitational wave detectors—may yield empirical data that challenges or refines current models of spacetime.
Ultimately, as we deepen our understanding of spacetime, we also expand our grasp of the universe itself. If we can successfully bridge the gaps between different areas of physics, we might not only gain theoretical insights but also practical breakthroughs that could reshape technology and enhance our understanding of existence.