Understanding Quarks: The Core of Matter
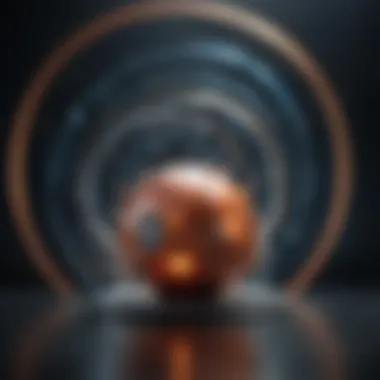
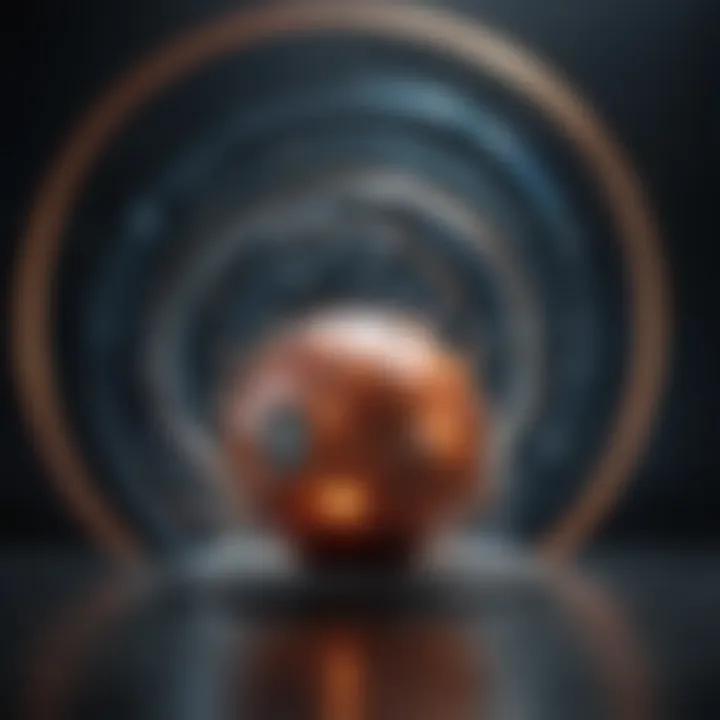
Intro
When one digs into the tiny universe of particle physics, the quark stands out as a cornerstone of matter. Though these particles may be exceedingly small, they hold a staggering significance in the realm of physics, altering our very understanding of how the universe works.
In this discussion, we will steer through the essential facets of quarks, dissecting their roles, characteristics, and interactions. This will offer a vista not only into their theoretical implications but also their experimental verification and contribution to modern physics. Quarks are not just abstract concepts—they are tangible entities that shape the atoms which constitute everything around us.
To really appreciate the marvel of quarks, one must consider the numerous discoveries and insights that have progressively unveiled their nature through extensive research.
Prologue to Quarks
To understand the underpinnings of matter, one cannot overlook the fundamental role of quarks. These elusive particles are the very building blocks that combine to form protons and neutrons, which in turn make up the atomic nucleus. Delving into the realm of quarks equips us to grasp not just the microcosm of particle physics, but the larger tapestry of the universe itself. By digging into this topic, we not only reveal the secrets of matter but also the intricate forces that govern its behavior.
This section sets the stage for a journey through the world of quarks, highlighting their significant role in our understanding of the cosmos. We will examine the historical context, which gives insight into how our comprehension of quarks evolved, as well as their critical position in the framework of matter.
Historical Context
The concept of quarks emerged in the mid-20th century, a time when physicists were desperately attempting to unravel the mysteries of atomic structure. In the early days, particles such as electrons and protons were considered the most fundamental entities. However, with advances in particle accelerator technology, it became evident that protons and neutrons themselves were not elementary but made up of smaller particles.
The term "quark" was first coined by Murray Gell-Mann in 1964, a name inspired by a line from James Joyce's Finnegans Wake. Gell-Mann, alongside George Zweig, proposed a model consisting of three types of quarks: up, down, and strange. The idea wasn’t without its naysayers, but the subsequent discovery of various particles like the muon, an unexpected variant of electrons, validated the notion that there existed a more intricate substructure to matter. Through subsequent research and experimental techniques like deep inelastic scattering, physicists were able to confirm the existence of quarks, marking a significant leap in the development of the Standard Model.
The Role of Quarks in Matter
Understanding quarks is key to unraveling the nature of matter itself. Quarks are not merely theoretical constructs; they play an essential part in the composition of protons and neutrons, which are the building blocks of atomic nuclei. For instance, a proton consists of two up quarks and one down quark, while a neutron is made up of two down quarks and one up quark. These arrangements are crucial because they determine the properties of the atom, including its charge, stability, and interactions with other particles.
Moreover, quarks contribute to the strong force that holds the nucleus together—a force much stronger than gravity but only effective at very short ranges. This force is mediated by particles known as gluons. Without quarks, there would be no strong force, and thus no stable matter as we know it. Therefore, to appreciate the complexity of matter, one must take a closer look at quarks and their interactions.
In summary, the journey into the world of quarks opens the door to understanding the fabric of reality. By examining the historical progress in the study of these particles and their vital function in the makeup of matter, we can pave the way for more profound insights into the universe. It is through this lens that we will further explore the theoretical foundations, types, confinement, and interactions of quarks.
Theoretical Foundations
Theoretical foundations in quark research are vital as they provide the framework for understanding how these tiny particles operate and interact. They enable researchers to construct models that not only describe phenomena observed in high-energy particle physics but also predict new behaviors and interactions. Developing a solid theoretical base allows scientists to make sense of the complex behaviors exhibited by particles at the subatomic level.
A significant aspect of theoretical foundations is the establishment of models that categorize quarks and their interactions. This aids in the classification of particles and helps to bridge gaps in understanding within the field of particle physics. By digging into these theoretical constructs, students, educators, researchers, and professionals can develop deeper insights into the fundamental building blocks that compose matter, leading to groundbreaking discoveries and advancements in technology.
Quark Model Basics
The quark model is an essential framework in theoretical particle physics that outlines how quarks come together to form hadrons, which include protons and neutrons. The model divides quarks into different flavors, namely up, down, strange, bottom, and top quarks. This classification is not just an arbitrary grouping but is based on the properties of quarks, such as their mass, charge, and behavior.
To understand the quark model, visualize it like mixing various ingredients to get a specific dish. Each quark flavor plays a crucial role in determining the properties of the resultant hadron. For instance, a proton consists of two up quarks and one down quark, giving it a positive charge, while a neutron comprises one up quark and two down quarks, rendering it electrically neutral.
It's worth noting that quarks are never found alone in nature; they are always confined within hadrons due to a phenomenon called confinement. Their interactions and relationships are outlined by the guidelines of quantum chromodynamics (QCD), which we shall delve into next.
Quantum Chromodynamics Explained
Quantum chromodynamics is the field of physics that describes the strong interaction, one of the four fundamental forces, responsible for holding quarks together within protons and neutrons. QCD posits that quarks possess a property known as color charge, which has nothing to do with visible colors but rather is a crucial aspect of how these particles interact via the exchange of particles called gluons. Each gluon mediates the strong force, effectively acting as the glue that binds quarks together.
QCD is a complex and nuanced area, requiring an understanding of several key elements:
- Color Charge: Just like electric charge in usual physics, color charge comes in three types: red, green, and blue. This makes the interactions more intricate than they would be under simpler models.
- Asymptotic Freedom: An essential aspect of QCD, which means that quarks become less interactive and free when they are close to each other and stronger when they are farther apart, adding a layer of complexity to quark interactions.
- Confinement: This further emphasizes that quarks cannot exist independently; they are perpetually bound in combinations that yield neutral color charges.
While QCD has made substantial advances in describing quark interactions at short distances, many challenges remain. Researchers continue to explore its implications, with ongoing studies examining the behavior of quarks under various conditions.
"In the realm of quantum chromodynamics, understanding the subtleties of quark interactions opens doors to comprehending the universe's very fabric."
In summary, the theoretical foundations of quarks establish a broad platform essential for researchers aiming to advance our understanding of the universe, revealing details about both the microcosm of particles and the broader implications in the realm of physics.
Types of Quarks
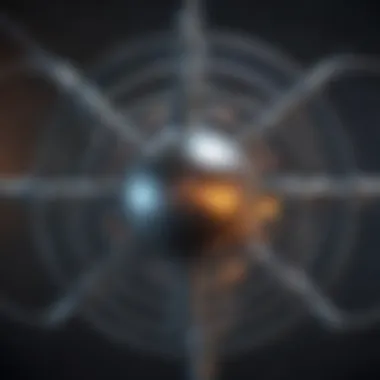
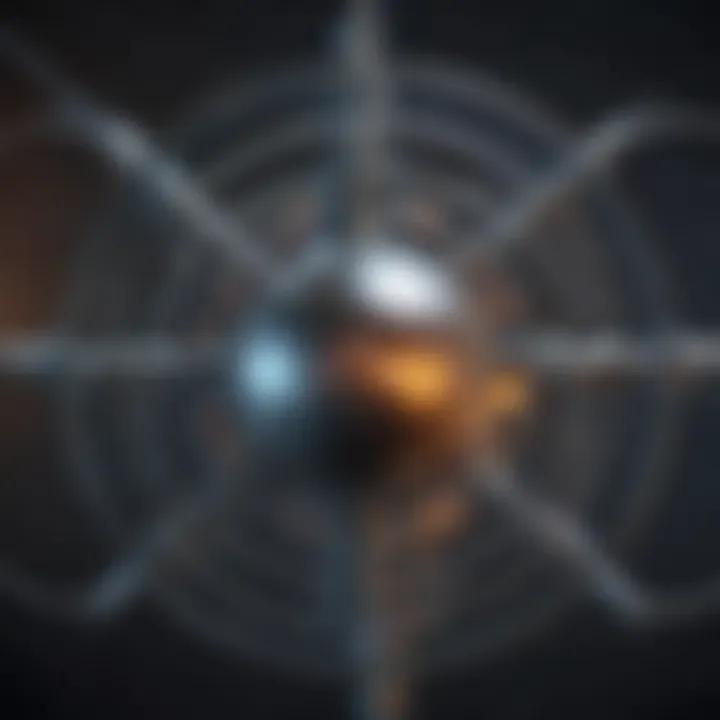
Understanding the various types of quarks is crucial for comprehending the overall structure of matter at its most fundamental level. Quarks are not just abstract concepts; they are tangible elements that combine to form protons and neutrons, which then build the atomic nuclei that make up everything around us. By breaking down the distinctions among different quark varieties, we gain insights into the interactions and forces that govern the behavior of matter. Beyond just academic pursuits, exploring these types can yield tangible applications in particle physics, cosmology, and even materials science.
Flavor Varieties
Delving into the flavor varieties of quarks allows us to appreciate the colorful tapestry of interactions that exist at the subatomic level. Each type of quark brings its own distinctive traits and contributions to the table, shaping the behavior of the particles made from them.
Up Quark
The Up quark is a real heavyweight in the quark spectrum, so to speak. It carries a charge of +2/3, which is pivotal in our understanding of how protons maximize stability. Since protons, essential components of atoms, are composed of two Up quarks and one Down quark, the Up quark plays a fundamental role in forming stable matter. Its unique characteristic of having a relative light mass compared to its counterparts makes it integral in binding pairs within even larger particles.
Down Quark
Contrastingly, the Down quark has a charge of -1/3. While often overshadowed by its up counterpart, the Down quark is vital for the formation of neutrons, the counterparts of protons in atomic nuclei. The balance between Up and Down quarks allows neutrons and protons to achieve stability and perform their roles in the atomic nucleus effectively. Furthermore, in certain interactions, its slightly heavier mass when compared to the Up quark may influence particle behaviors and decay modes, which are essential for ongoing research in physics.
Strange Quark
Then there's the Strange quark, which adds an interesting twist, quite literally. It has a charge of -1/3 and is heavier than both Up and Down quarks. The presence of Strange quarks in particles, known as strangeons, leads to unique properties and interactions that often defy the conventional wisdom rooted in standard particles. Their prominence in mesons is crucial for understanding phenomena such as particle asymmetry in the universe, sparking curiosity among physicists.
Bottom Quark
The Bottom quark dives even deeper, embodying more mass and a charge of -1/3. This quark's heavier weight becomes significant in high-energy physics scenarios, particularly in hadron colliders. Its relatively short lifespan makes it an intriguing subject for particle physicists studying the mechanics of B-mesons. The Bottom quark can teach us about flavor physics—the study of how quarks of different types can oscillate between states, ultimately enriching our knowledge of fundamental forces.
Top Quark
Lastly, the Top quark is the heavyweight champion of the quark family, carrying a whopping charge of +2/3 and residing at the upper end of the quark mass spectrum. Its discovery was a landmark in particle physics, owing to its surprising mass, which is significantly greater than that of the other quarks. The Top quark's fleeting existence challenges researchers to capture its behavior in experiments, and understanding it could lead to breakthroughs in our grasp of the Standard Model. Additionally, it plays a critical role in electroweak interactions, thereby highlighting its importance in mass generation processes.
Quarks and Their Charges
Quarks possess fractional electric charges, distinguishing them from the more familiar electrons or protons, which carry integer charges. This intriguing characteristic not only contributes to the stability of atomic structures but also aids in comprehending the dynamics of particles.
The various charges of quarks underscore the complexity of forces at play within the atom:
- Up quarks: +2/3
- Down quarks: -1/3
- Strange quarks: -1/3
- Bottom quarks: -1/3
- Top quarks: +2/3
This fractional charge system contributes to the strong-force interactions, and developments in our understanding of these charges usher us toward achieving a more profound comprehension of the universe and the particles that constitute it.
"Understanding the types of quarks is not just key to particle physics; it's fundamental to unraveling the very essence of matter itself."
With this foundational grasp on the types of quarks, readers should appreciate the intricate tapestry of subatomic particles while recognizing their broader implications on the field of theoretical and experimental physics.
Quark Confinement
Quark confinement is a fundamental principle that sheds light on the behavior of quarks and their interactions. Unlike other particles, quarks are never found in isolation; they are always bound together in groups, forming larger particles like protons and neutrons. This phenomenon presents an intriguing puzzle for physicists. Understanding quark confinement is essential, as it influences many aspects of particle physics and helps clarify the essential nature of matter itself.
Understanding Confinement
At its core, quark confinement is tied to the strong force, one of the four fundamental forces of nature. It explains how quarks interact with one another through the exchange of particles called gluons. Gluons essentially act as the glue holding quarks together, but there's a catch. As quarks try to move apart, the strong force actually becomes stronger, making it increasingly difficult for them to separate. The energy involved in this force leads to the creation of new quark-antiquark pairs rather than isolating a single quark.
This behavior can be likened to trying to stretch a rubber band: the further you pull, the more tension builds up, eventually snapping back. In terms of quarks, rather than being able to reach out freely, they remain tightly secured to one another within particles, creating a stable structure that ultimately composes atomic nuclei.
Implications for Particle Physics
The implications of quark confinement in particle physics are expansive. For one, it significantly influences the way physicists understand hadrons, the particles made up of quarks. Because quarks are confined within these hadrons, their properties can only be studied indirectly. Understanding how confinement works thus helps physicists interpret experimental data regarding matter at a subatomic level.
Also, the concept of confinement plays into theories concerning the early universe. In the moments after the Big Bang, it is theorized that quarks roamed freely in a quark-gluon plasma before becoming confined into protons and neutrons. This theory has profound implications, suggesting how the universe's matter evolved over time.
"Quark confinement is like the locked door in a club; once you cross the threshold, it's tough to leave without a ticket to get back in."
Furthermore, advancements in our understanding of confinement lead to possible future discoveries, such as new state of matter or even insights into dark matter. As researchers continue exploring this topic, the enigma of confinement could potentially unveil new realms of knowledge, shaping the future of quantum physics.
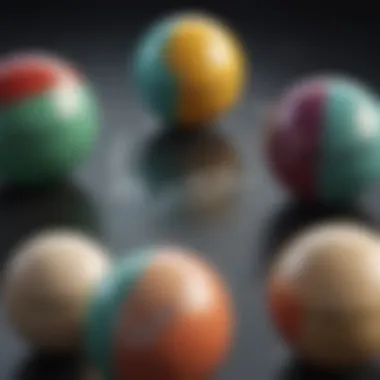
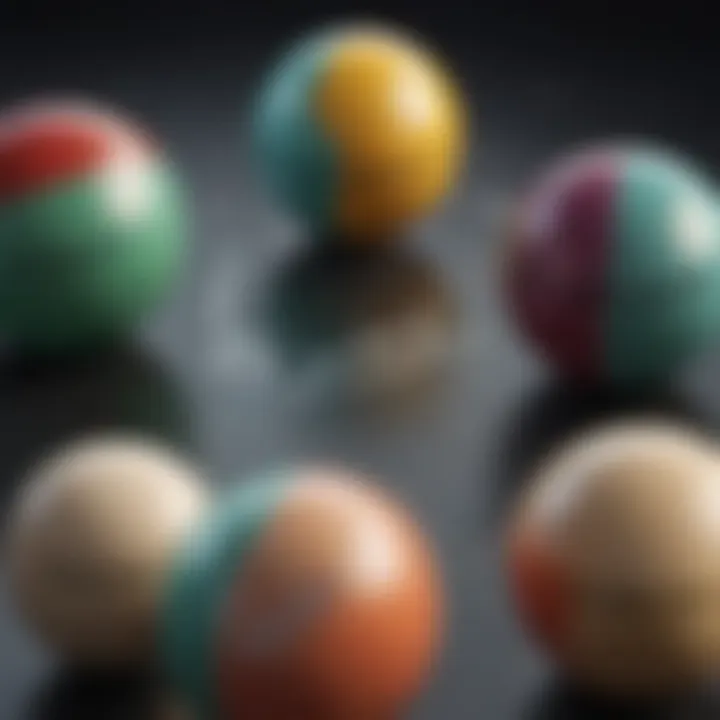
Quark Interactions
Quark interactions are pivotal in the realm of particle physics, revealing not only the intricate relationships among quarks but also how these interactions shape matter itself. Understanding quark interactions is crucial, as they govern the dynamics of protons and neutrons, which are the very building blocks of atomic nuclei. The interactions that quarks undergo play significant roles in defining properties like mass, stability, and decay processes. Broadly, these interactions arise from the strong force, also known as the strong nuclear force, and it is vital to grasp its nature to appreciate the full picture.
Strong Force Dynamics
The strong force has the ability to keep quarks tightly bound together, which is fundamentally different from other fundamental forces like electromagnetism or gravity. Its strength is what holds protons and neutrons together, despite the substantial repulsive electromagnetic forces between the positive charges of protons. This force operates at a very short range but is immensely powerful. Without this strong interaction, atomic structure as we know it would not exist.
Here are some key characteristics of the strong force:
- Short Range: The strong force acts effectively only over distances on the order of 1 femtometer (10^-15 meters). Beyond this distance, its influence rapidly decreases.
- Confinement: Quarks are never found in isolation; they are always bound within larger particles like protons and neutrons. This phenomenon is known as quark confinement, which is driven by the strong force.
- Exchange Particles: Gluons, the force carriers of the strong force, facilitate the interactions between quarks, constantly exchanging momentum and energy.
In quark interactions, these dynamics dictate the formation of larger structures, underlining their essential role in particle physics.
Role of Gluons
Gluons are the unsung heroes in the interactions between quarks. They serve as the actions of the strong force, analogous to how photons mediate electromagnetic interactions. Each gluon acts as a messenger that enables quarks to communicate and exchange forces within hadrons. This exchange leads to a binding effect that is truly remarkable.
Notably, gluons possess some unique characteristics:
- Self-Interaction: Unlike other force carriers, gluons can interact with each other. This self-interaction is a distinct feature of quantum chromodynamics and adds complexity to strong force dynamics.
- Color Charge: Gluons carry a property called color charge, which is essential for the strong force. This charge comes in three types—red, green, and blue—facilitating the interaction among quarks that also exhibit these color charges.
- Rich Dynamics: The interactions mediated by gluons contribute to the complexities of hadronic structures, influencing their mass and stability.
In summary, the interactions among quarks—governed by the strong force and facilitated by gluons—are the bedrock of the matter in the universe. Recognizing how crucial these dynamics are can lead to a deeper understanding of not only particle interactions but the fundamental nature of existence itself.
Understanding these interactions paves the way for further exploration in particle physics, establishing a foundation that extends into understanding mass, decay, and the very stability of matter. The relevance of studying quark dynamics cannot be understated, as it is a key to unraveling the mysteries of the universe.
Experimental Evidence for Quarks
Understanding quarks fundamentally transforms our grasp of matter. Without experimental evidence, theories about these tiny particles would remain mere conjecture. In this section, we will explore key methods utilized to validate the existence and behavior of quarks, as well as their implications in modern physics.
Deep Inelastic Scattering
One pivotal experiment that shed light on quarks is deep inelastic scattering. In this method, high-energy electrons are directed at protons or neutrons. By analyzing how these electrons scatter after colliding with nucleons, physicists gain insights into the internal structure of these particles. Essentially, deep inelastic scattering acts like probing an onion, with each layer revealing more about what lies within.
As the high-energy electrons interact with the quarks, what scientists observe has been nothing short of revolutionary. The results indicate that protons are not solid particles but are instead made of smaller constituents—quarks. This experimental approach confirmed the existence of quarks and their fractional electric charges, thereby solidifying the quark model of particle physics.
In a nutshell:
- Protons are comprised of three quarks—two up quarks and one down quark.
- The energy transferred during scattering validates predictions about quark sizes and behaviors.
Current Research Initiatives
The exploration of quarks doesn't end with deep inelastic scattering. There are numerous ongoing research initiatives aimed at uncovering further details about these elusive particles. Projects like the Large Hadron Collider in CERN aim to collide protons at unprecedented energies. Scientists analyze the resulting particle interactions to glean hints about the nature of quarks. Observations of jet formations, which are clusters of particles produced during these high-energy collisions, play a crucial role in supporting and refining our understanding of quark dynamics.
Furthermore, neutrino scattering experiments are gaining traction as researchers aim to study quark interactions in different settings. These initiatives focus on muon and electron neutrinos striking protons, enabling researchers to examine how these particles interact under conditions that depict the primordial universe—offering glimpses into quark behaviors that could never be replicated on Earth.
- Innovations in technology continue to push the boundaries of what can be studied.
- Collaborations across international research teams ensure a diverse range of ideas and methodologies.
"Collisions at the energy levels we monitor offer an unparalleled opportunity to observe rare processes involving quarks, leading to advancements in our foundational understanding of the universe."
Ongoing research not only enhances knowledge about quarks but also has broader implications for comprehending forces and particles at large, ultimately painting a clearer picture of how matter is structured at the most fundamental levels.
The Standard Model of Particle Physics
The Standard Model of particle physics serves as a cornerstone of contemporary physics, laying the groundwork for our comprehension of the universe at its most fundamental level. By delineating the types of particles known to exist and their interactions, this model has provided insights that continually shape our understanding of matter and its behaviors. Essentially, it's a framework that brings coherence to the chaotic world of subatomic particles, allowing researchers, educators, and students alike to find common ground in a complex web of interactions and foundational principles.
Integration of Quarks in the Standard Model
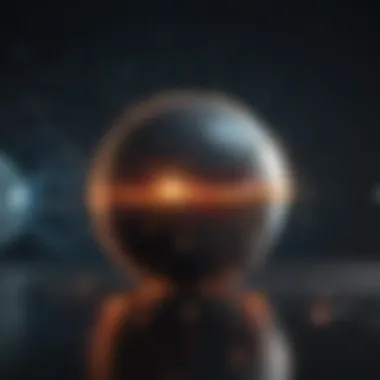
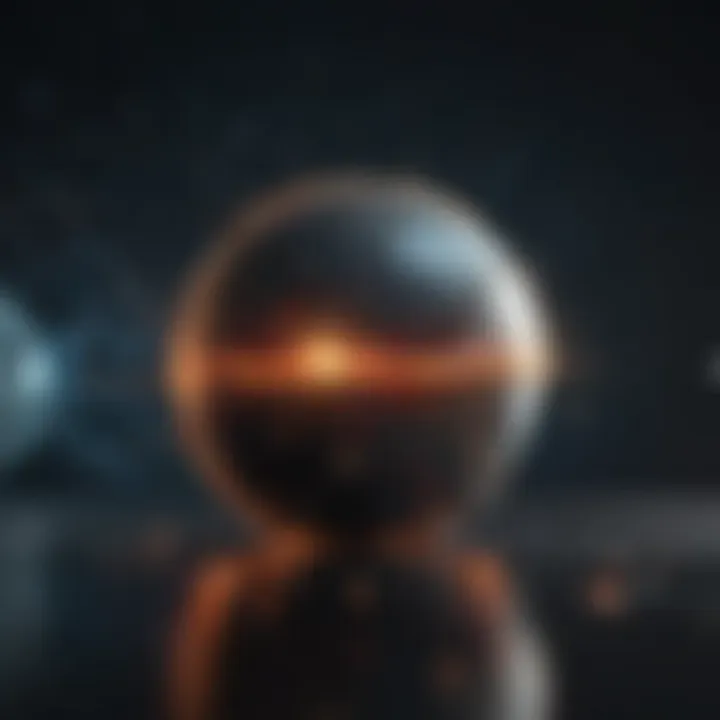
At the crux of the Standard Model lies the concept of quarks, which are indispensable to the makeup of protons and neutrons—collectively known as nucleons. Within this model, quarks are categorized into six distinct flavors: up, down, charm, strange, top, and bottom. Each of these flavors plays a crucial role in particle formation. For instance, protons are composed of two up quarks and one down quark, while neutrons consist of two down quarks and one up quark.
Moreover, the interactions between quarks are mediated by gluons, another key element of the Standard Model. These ‘glue’ particles ensure quarks remain bound together, highlighting how quarks maintain their integrity within nucleons. Not only does this speak to the profound interconnectedness of subatomic particles, but it also illustrates the elegance of the model itself, offering powerful predictions that have been experimentally validated. Without the integration of quarks and their dynamic interactions, the Standard Model would lose a significant portion of its explanatory power.
"The Standard Model is not just a theoretical construct; it's the most successful theory in physics, explaining a multitude of phenomena observed in nature."
Quarks vs. Leptons
To fully comprehend the landscape painted by the Standard Model, it’s essential to distinguish between its two primary constituents: quarks and leptons. While both categories are fundamental particles, they exhibit contrasting characteristics and functions.
- Quarks:
- Leptons:
- Constitute protons and neutrons.
- Are subject to the strong force, which is mediated by gluons.
- Come in six flavors and possess fractional electric charges (e.g., +2/3 for up quarks and -1/3 for down quarks).
- Include electrons, muons, taus, and their associated neutrinos.
- Do not experience the strong nuclear force, making them unaffected by gluons.
- Carry integer electric charges, with electrons being essential in electromagnetic interactions.
This dichotomy within the Standard Model not only illustrates the diverse types of matter particles but also emphasizes the various forces governing their interactions. While quarks bind together in complex ways to form baryons and mesons, leptons stand apart, contributing to different physical processes. This distinction enhances the richness of particle physics as a field of study, offering different avenues for exploration and understanding.
Challenges in Quark Research
The study of quarks presents a plethora of challenges that researchers in the field strive to address. These challenges are not just hurdles but gateways to deeper understanding in the realm of particle physics. Therefore, grasping the difficulties in quark research is essential; it opens both a window into existing knowledge gaps and a doorway to future inquiry.
To begin, one significant challenge lies in the precision of measurements. Quarks are elusive entities that can't be isolated in their free state due to confinement. This means experiments generally measure properties of larger particles, like protons and neutrons, which are themselves composites of quarks. This complicates the task of pinning down specific quark behaviors and makes modeling their interactions complex. The laws of Quantum Chromodynamics, which describe how quarks interact through the strong force, often rely on theoretical predictions rather than direct measurements, adding another layer of uncertainty.
As highlighted by physicists across the globe, "Quarks are like actors behind a glass wall; their roles are crucial, but seeing them up close is a whole different story."
Open Questions in Quantum Physics
In navigating the realm of quark research, various open questions remain. One of the most pressing is the nature of quark confinement. Researchers question what fundamentally causes quarks to remain bound within protons and neutrons, a phenomenon without a satisfactory theoretical explanation. Unlike other particles that can exist independently, quarks exhibit confinement, and understanding this could unlock new physics.
Another open question involves asymptotic freedom—the concept that quarks behave almost independently at extremely short distances while being strongly interacting at larger distances. The mechanics behind this transition remain a topic of active investigation and debate.
Lastly, there's much to unravel concerning the origin of mass—specifically, how quarks acquire mass through the Higgs mechanism. The incomplete nature of our understanding in these areas reiterates the complexity inherent in particle physics and the urgent need for further study.
Future Directions for Study
Looking ahead, future studies in quark research may take several directions. Advances in accelerator technology will likely lead to improved experimental setups capable of probing deeper into the behavior of quarks. Notably, high-energy particle colliders such as the Large Hadron Collider (LHC) will continue to offer invaluable data, which could reveal unforeseen aspects of quark interactions and properties.
In addition, researchers are exploring machine learning techniques to analyze vast datasets generated from quark interactions. By applying these advanced algorithms, they may uncover patterns and correlations that traditional analysis might overlook.
Importantly, theoretical physicists will need to refine quantum chromodynamics models to integrate new observations. These enhanced models could yield insights into not just quarks but the fundamental structure of matter itself.
The fusion of theory with cutting-edge technology paves the way for a more comprehensive understanding of the quark domain. As physicists continue to ask the hard questions and pursue innovative answers, the enigmatic world of quarks will inevitably reveal its secrets, one fragment at a time.
Closure
The concept of quarks has ushered in a new era in the understanding of matter and the universe itself. Their role as fundamental constituents of protons and neutrons is not merely a footnote in the annals of scientific discovery; it stands as a cornerstone of modern physics. Quark research serves not only to deepen our grasp of atomic structure but also to challenge and refine our understanding of the fundamental forces that govern the realm of subatomic particles.
The Significance of Quark Research
The significance of quark research extends well beyond theoretical constructs. As scientists delve into the complexities of quarks, they uncover the layers of reality that form the basis of everything around us. The exploration of quarks leads to several key benefits:
- Enhanced Understanding of Matter: Through quark interactions, researchers can elucidate how matter is constructed, revealing the intricate tapestry of particles and forces.
- Foundation for Particle Physics: The study of quarks is fundamental in particle physics, aiding in the development of the Standard Model and its ongoing evolution.
- Applications in Technology: Insights gained from quark research have far-reaching applications, potentially influencing fields like materials science and medical imaging.
Quark research not only answers questions but also raises new ones. Scientists ponder the implications of quark confinement and the dynamics of strong force interactions, leading to exciting inquiries into the underpinnings of the universe.
Implications for Future Physics Discoveries
Looking ahead, the implications of understanding quarks are vast. As knowledge advances, it is not just about grasping existing relationships; it's about opening doors to entirely new domains of inquiry. Some notable implications include:
- Discovering New Particles: Ongoing research could unveil new particles, further expanding the particle family.
- Testing Theories: Innovations in experimental methodologies might enable researchers to test existing theories like Quantum Chromodynamics more rigorously.
- Exploring Dark Matter: A deeper grasp of quarks may someday lead to breakthroughs in understanding dark matter, a component that remains elusive yet critical to the cosmos.
"Quarks are not just pieces of the puzzle; they are the very fabric that stitches the universe together."
Exploring these potentialities instills excitement in the scientific community. The drive to demystify quarks carries implications that stretch into the very core of physics, hinting at the discoveries that linger just beyond the horizon. Such exploration not only fulfills the quest for knowledge but also elevates our understanding of existence itself, paving the way for a deeper appreciation of the universe in which we reside.