Understanding Quantum Physics Simulation: A Detailed Overview
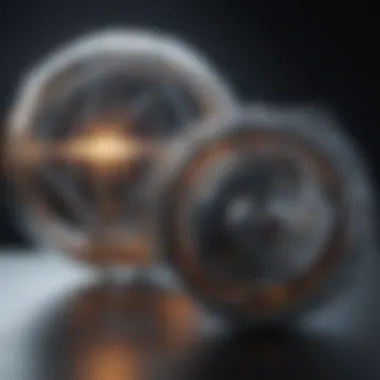
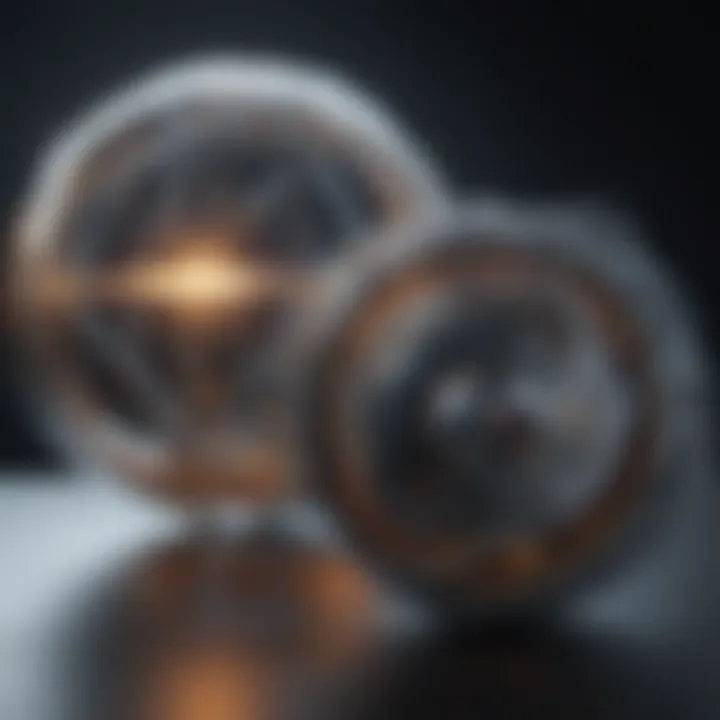
Intro
The realm of quantum physics simulation stands at the cutting edge of scientific exploration and technological innovation. As researchers and professionals increasingly turn to quantum simulations, they grapple with the nuances and complexities that these advanced methodologies encompass. This section aims to provide a detailed framework, setting the stage for deeper dives into essential themes such as key research findings, the breakdown of sophisticated concepts, and the broader implications of these technologies.
Quantum simulations are not merely theoretical exercises; they have practical applications that span various sectors including materials science, cryptography, and complex system analysis. The capacity to model quantum systems enables a more profound understanding of phenomena that classical computers struggle to compute accurately, thus catalyzing advancements in technology and scientific inquiry.
As we journey through this exploration, we will emphasize the crucial findings that have emerged, the methodologies used, and their anticipated impact on the future of diverse fields. By demystifying quantum physics simulations, we aim to elucidate how they contribute to a collective understanding of the universe and to the harnessing of quantum mechanics for practical use.
Key Research Findings
Overview of Recent Discoveries
Recent strides in quantum physics simulation have fundamentally altered our comprehension of matter and energy at microscopic levels. Notable advances include the development of sophisticated algorithms that enable simulations of quantum systems with increasing fidelity. For instance, breakthroughs in quantum Monte Carlo methods have improved the efficiency of simulating many-body problems, which are pivotal in the study of quantum phenomena.
In addition, the emergence of quantum computers has introduced new paradigms in simulation approaches. This technology allows for the modeling of intricate systems that were previously computationally prohibitive. Scientists are observing how quantum entanglement and superposition can be leveraged to solve complex equations that emerge in various scientific contexts.
Significance of Findings in the Field
The importance of these findings cannot be overstated. As quantum simulations enhance our ability to predict material properties and chemical reactions, industries from pharmaceuticals to renewable energy are beginning to harness these insights to innovate more effectively and sustainably. The results not only contribute to advancements in material design but also to breakthroughs in drug discovery.
"Quantum simulations represent a new frontier in the quest to unlock the mysteries of the universe and to build technologies that will shape our future."
Through ongoing research and technological refinement, the trajectory of quantum physics simulation is likely to yield even more transformative insights. Understanding these developments equips students, researchers, and professionals with the knowledge to engage with the forefront of modern science.
Breakdown of Complex Concepts
Simplification of Advanced Theories
Navigating the complexities of quantum physics can be daunting. However, breaking down advanced theories into understandable components makes this field more accessible. For example, quantum entanglementโoften viewed as esotericโis increasingly being dissected into tangible principles with direct applications in communication and computation.
Several core concepts can be simplified for clarity:
- Quantum Superposition: The principle that particles can exist in multiple states simultaneously until measured.
- Entanglement: A phenomenon where particles become interconnected, such that the state of one instantly influences the other, regardless of distance.
- Quantum Tunneling: The ability of particles to pass through barriers that classical physics would deem impassable.
Visual Aids and Infographics
Utilizing visual aids and infographics can significantly enhance comprehension. Diagrams that illustrate quantum states, entanglement connections, or simulation processes provide clarity to abstract ideas. They serve not only as learning tools but also as essential components in disseminating complex scientific concepts to a broader audience.
In summary, this narrative paves the way for a holistic understanding of quantum physics simulation, shedding light on both its theoretical underpinnings and practical implications. As we continue through this article, further sections will elaborate on specific technological advancements and applications that are poised to transform various fields.
Prelims to Quantum Physics Simulation
Quantum physics simulation is an essential academic and scientific field, bridging complex theories with practical application. The simulation enables researchers to explore quantum systems that are not easy to study in laboratories. In addition, quantum simulation allows for predictions about the behavior of particles at a quantum level, which can be critical in many areas such as materials science and drug development.
This section intends to lay the groundwork for understanding quantum physics simulation, elucidating its importance and elucidating key concepts. As the world of technology advances, simulations will increasingly play a role in solving real-world problems by providing insights that traditional methods cannot achieve. With the rapid pace of advancements in quantum computing, recognizing the significance of these simulations is crucial for anyone involved in scientific research.
What is Quantum Physics Simulation?
Quantum physics simulation refers to the replication of quantum systems through computational models. This involves using classical computing resources to understand how quantum systems behave under various conditions. Unlike traditional physics simulations that primarily deal with classical mechanics, quantum simulations account for principles unique to quantum theory, such as superposition and entanglement.
The goal of quantum simulation is to calculate properties of matter at the smallest scales. It allows researchers to model phenomena that occur in quantum systems such as electron interactions, excitations, and phase transitions, which otherwise cannot be observed directly. These simulations can be done using various computational algorithms, often adapted to run on classical computers.
Prominent approaches to quantum physics simulation include the use of quantum circuits and hybrid techniques that blend classical and quantum computations. The historical evolution of these techniques closely follows the development of quantum theory itself and parallels advancements in computer science. As quantum processors grow more sophisticated, the landscape of simulation will surely evolve as well.
Importance of Simulation in Quantum Physics
The role of quantum simulation in modern physics cannot be overstated. Several key aspects underscore its importance:
- Understanding Complex Systems: Many natural phenomena are inherently quantum mechanical, such as superconductivity and magnetism. Simulation facilitates the exploration of these systems beyond the capacity of direct experimentation.
- Accelerating Discovery: Quantum simulations can significantly speed up the process of exploring new materials and drugs. By simulating interactions at the quantum level, researchers can narrow down which compounds are worth studying in the lab.
- Validation of Theories: Simulations serve as a tool to confirm or challenge existing theories in quantum mechanics. Through rigorous testing of predictions, scientists can refine their models.
"Quantum simulation can elucidate complex phenomena that may take years of experimentation to understand, enabling revolutions in technology and materials."
- Impact on Technology Development: Quantum simulation paves the way for advancements in technologies such as quantum computing, cryptography, and nanotechnology. Each of these fields relies on our understanding of quantum mechanics.
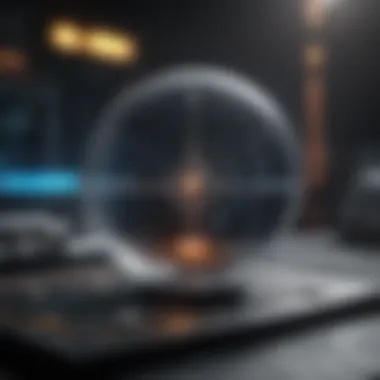
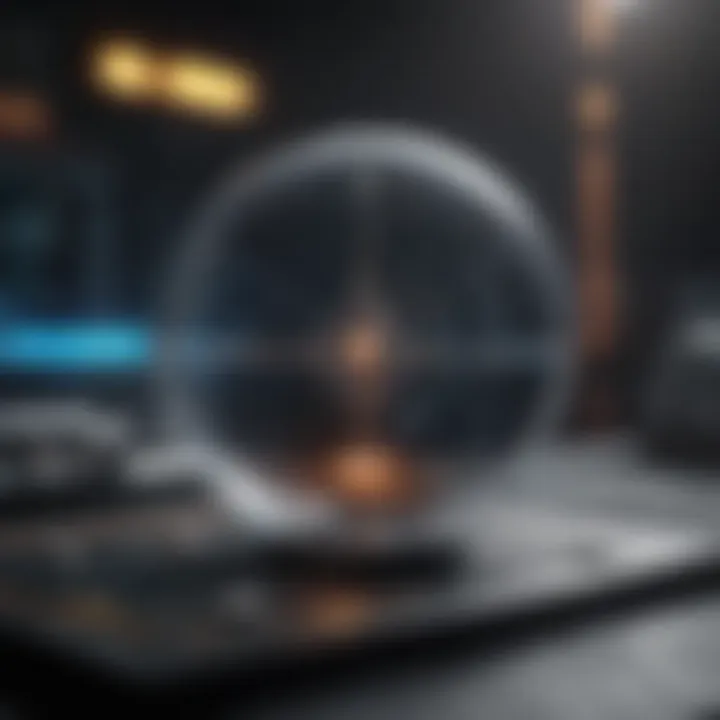
In summary, the significance of quantum physics simulation lies in its ability to transform theoretical concepts into practical applications, ultimately contributing to scientific advancement and technological innovation.
Historical Context
Understanding the historical context of quantum physics simulation is essential. This section provides insights into the foundational developments that shaped the field. By examining the origin and evolution of quantum theory and simulation techniques, one can appreciate the significance of advancements in computational physics. Historical milestones contribute to knowledge in the discipline, influencing current methodologies and future trajectories.
Origin of Quantum Theory
Quantum theory emerged in the early 20th century, fundamentally altering the understanding of physics. Max Planck's work in 1900 on blackbody radiation introduced the concept of quantized energy levels. This was a radical shift from classical physics, which treated energy as continuous. Albert Einstein expanded on these ideas in 1905, providing an explanation for the photoelectric effect via quantized light particles, or photons.
These developments led to further exploration by Niels Bohr, who in 1913 proposed a model of the atom that encompassed these quantum principles. By understanding how electrons occupy discrete energy levels, a greater comprehension of atomic behavior was achieved, which laid the groundwork for future simulations in quantum physics.
"Quantization is the key to understanding the very fabric of the universe, opening doors to new scientific horizons."
Evolution of Simulation Techniques
As quantum theory matured, so did the methodologies for simulating quantum systems. The mid-20th century saw the advent of computational techniques that utilized digital computers to model quantum phenomena. These methods initially focused on approximations due to the complexity of quantum systems.
With the development of algorithms, researchers improved the accuracy of simulations. Notable examples include the Hartree-Fock method, which approximated the behavior of electrons in atoms, and later, the Density Functional Theory (DFT), which allowed for large-scale simulations of molecular structures.
In recent years, the rise of quantum computing has revolutionized simulation techniques. Quantum computers show promise in processing complex quantum systems more efficiently than classical approaches. This progress draws directly from the historical evolution that began with initial quantum theories and has led to sophisticated computational frameworks used today.
The historical context is vital, as it demonstrates how theories transitioned into practical applications and sheds light on the significance of ongoing research in quantum simulation.
Fundamental Principles of Quantum Mechanics
The fundamental principles of quantum mechanics form the bedrock of modern physics. Understanding these principles is essential for grasping how quantum physics simulations work and their applications in various fields. This section focuses on key elements such as wave-particle duality, superposition, entanglement, and the uncertainty principle. Each of these concepts plays a crucial role in explaining quantum phenomena and influences simulation methodologies.
Wave-Particle Duality
Wave-particle duality is a cornerstone of quantum mechanics, revealing that particles like electrons and photons exhibit both wave-like and particle-like properties. This dual behavior challenges classical intuition, which sees light as a wave and matter as particles. The famous double-slit experiment starkly demonstrates this principle, where particles create an interference pattern when not observed, suggesting they behave as waves. However, upon observation, they act like particles.
Through quantum simulations, scientists can model systems that leverage this duality. Understanding wave-particle duality is essential in fields like optics and nano-technology, where the manipulation of light and matter is crucial. Researchers utilize simulation techniques to predict and analyze how systems behave under various conditions, greatly enhancing experimental designs.
Superposition and Entanglement
Superposition and entanglement are unique aspects of quantum mechanics that further complicate intuitive understanding. Superposition allows particles to exist in multiple states simultaneously until measured, leading to behavior that defies classical physics. For example, an electron in a quantum state can spin both up and down at the same time. This aspect is pivotal in quantum computing, where bits can process more information than classical bits.
Entanglement, on the other hand, describes how particles remain interconnected even when separated by large distances. The measurement of one particle instantly affects the state of its entangled partner, irrespective of the space between them. This phenomenon has profound implications for information transfer and security, especially in quantum cryptography.
In simulations, these principles are instrumental. They allow physicists to explore complex interactions in multi-particle systems and assess behaviors like quantum tunneling. Effective simulations can help optimize designs for quantum computers, greatly enhancing processing power and capabilities.
Uncertainty Principle
The uncertainty principle, articulated by Werner Heisenberg, posits that it is impossible to simultaneously know the exact position and momentum of a particle. This principle is not due to limitations in measurement techniques, but rather it is a fundamental property of quantum systems. The more precisely one property is measured, the less precise the other becomes. This principle has far-reaching consequences, particularly in how we interpret phenomena across physics.
In quantum simulations, accounting for the uncertainty principle is essential. It influences the design of algorithms and the representation of state spaces. By understanding this principle, researchers can make more accurate predictions and model systems that behave according to quantum laws, ultimately contributing to the development of new materials and technologies.
Understanding the fundamental principles of quantum mechanics is not just theoretical. It has real-world implications across technology, materials science, and more.
Thus, the fundamental principles of quantum mechanics are critical in driving advancements in quantum physics simulation. A thorough comprehension allows researchers to better model, analyze, and innovate across various disciplines.
Computational Techniques in Quantum Simulation
Computational techniques in quantum simulation are crucial for advancing our understanding of quantum systems. These methods encompass a range of approaches used to model and analyze quantum phenomena. Knowing these techniques is vital for students, researchers, and professionals as they navigate this complex field. The ability to perform simulations directly impacts research outputs, enabling the exploration of phenomena that are challenging or impossible to study experimentally.
Classical vs Quantum Simulation Methods
Classical simulation methods utilize traditional computing approaches. These methods can often be limited in accurately modeling quantum behaviors due to the inherent complexity of quantum states. However, classical simulation remains relevant for simpler systems or where quantum effects can be approximated.
On the other hand, quantum simulation methods exploit quantum mechanics to solve problems more naturally. Quantum algorithms can operate on quantum bits or qubits, helping to simulate systems at an exponentially reduced computational cost. This advantage allows for modeling complex quantum systems, which is essential in fields such as material science and quantum computing development.
Key differences between classical and quantum simulation methods include:
- Scalability: Quantum methods can scale better with complexity.
- Accuracy: Quantum simulations provide more precise results for quantum systems.
- Resource Consumption: Classical simulations may become infeasible on larger systems due to time and memory constraints.
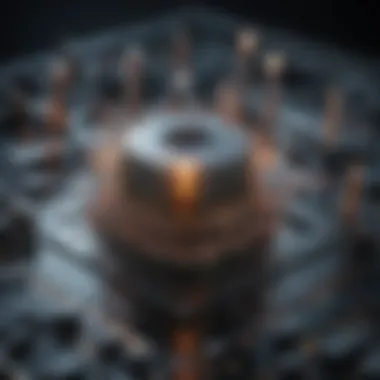
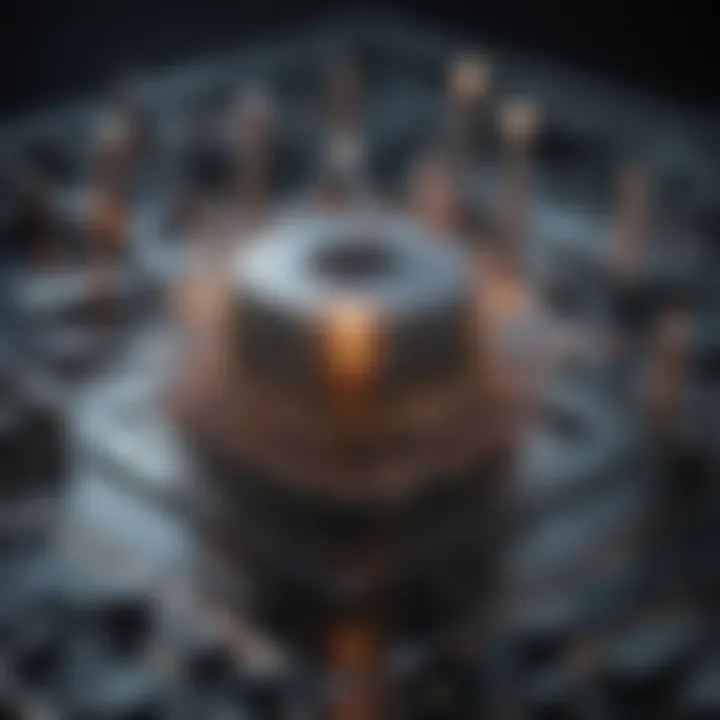
Algorithms for Quantum Simulation
Algorithms play a pivotal role in quantum simulation. Significant advancements have been made, particularly with the development of quantum algorithms like the Quantum Phase Estimation and the Variational Quantum Eigensolver. These algorithms are designed to solve specific problems efficiently on quantum computers.
Understanding these algorithms allows researchers to harness the potential of quantum hardware. For example, the Quantum Phase Estimation algorithm can precisely determine the eigenvalues of a Hamiltonian, which is crucial in various quantum applications.
Some notable quantum algorithms include:
- Quantum Phase Estimation
- Variational Quantum Eigensolver
- Quantum Monte Carlo Methods
Each of these algorithms is designed to tackle unique challenges in quantum simulations, revealing new insights into quantum systems.
Quantum Circuit Simulation
Quantum circuit simulation is an integral part of quantum computing. This involves modeling how quantum circuits process information using qubits. It allows for the experimental testing of quantum algorithms before implementation on actual quantum hardware.
Simulating quantum circuits can be done using classical computers with specialized software tools. Tools such as Qiskit and Cirq provide frameworks for building and running quantum circuits. They allow researchers to visualize quantum operations and evaluate the performance of quantum algorithms in a controlled environment.
Furthermore, understanding quantum circuit behavior is essential for optimizing quantum algorithms. By simulating circuits, researchers can identify potential issues with fidelity and error rates, which are critical for improving quantum computing technologies.
Quantum circuit simulation serves as a bridge between theory and practical applications, laying a foundation for breakthroughs in quantum technology.
Through these computational techniques, the realm of quantum physics simulation is not just theoretical but a functioning and developing field that contributes to scientific and technological innovation.
Applications of Quantum Physics Simulation
The applications of quantum physics simulation are vast and deeply interconnected with several scientific and technological advancements. Understanding these applications is crucial for grasping the importance of quantum simulations in addressing complex real-world challenges. This section delves into three key areas: material science, pharmaceutical research, and quantum computing development. Each of these fields benefits from the application of quantum simulations in unique ways, presenting new opportunities and potential innovations.
Material Science
Material science significantly benefits from quantum physics simulation. Researchers utilize these simulations to investigate the properties of materials at a quantum level. This approach allows for the accurate prediction of material behavior, leading to the discovery of new materials with desirable qualities. For instance, quantum simulations can reveal information about electronic structures, providing insights into conductivity, magnetism, and other important properties.
The ability to simulate these phenomena saves time and resources in material development. Traditional experimental methods can be expensive and time-consuming. By using quantum simulations, scientists can quickly evaluate various material candidates without physical prototypes. This efficiency is vital for sectors such as electronics and energy, where new materials can lead to groundbreaking technologies.
"Quantum simulations not only save resources but also push the boundaries of material discovery, potentially leading to innovations in energy storage and conversion."
Pharmaceutical Research
In the pharmaceutical sector, quantum physics simulation plays an essential role in drug discovery and development. The process of identifying potential drug candidates is often lengthy and expensive. With quantum simulations, researchers can model molecular interactions and predict how different compounds will behave when interacting with biological systems. This modeling helps in identifying promising drug candidates more quickly than traditional methods.
Quantum simulations allow for insights into the binding affinities and interaction dynamics between drugs and targets, which are fundamental in designing effective therapies. Moreover, this technology enables the optimization of lead compounds, enhancing efficacy while reducing side effects. As a result, quantum simulations can significantly accelerate the drug development timeline, improving the prospects for timely treatments for various diseases.
Quantum Computing Development
Quantum computing itself stands as a testament to the applicability of quantum physics simulation. The development of quantum computers relies heavily on simulating their complex operations and potential capabilities. Quantum simulations offer a platform for evaluating various algorithms and approaches that can be employed in quantum computing.
The ability to model quantum algorithms helps in understanding the computational advantages they can provide over classical computing methods. Researchers can test edge cases and optimize algorithm performance before deploying them on actual quantum systems. This predictive capability is crucial, as it can determine the future efficiency of quantum computers in solving complex problems that are currently beyond the reach of classical computers.
In summary, the applications of quantum physics simulation in material science, pharmaceutical research, and quantum computing development are just a few examples of how this field is shaping the future of science and technology. Each application brings about distinct benefits, fostering innovation and providing solutions to pressing global challenges. Understanding these applications is vital for anyone interested in the impactful role of quantum simulations in modern research.
Challenges in Quantum Simulation
The domain of quantum simulation presents a variety of challenges that need careful analysis and resolution. These obstacles are not just technicalities; they significantly impact the advancement and application of quantum physics. Understanding these challenges is crucial for researchers and practitioners who are navigating this complex landscape. Recent progress in quantum technology showcases the importance of addressing these issues properly. By doing so, the results from quantum simulations can greatly enhance scientific research and technological development.
Computational Limitations
One major challenge in quantum simulation lies in the computational limitations that arise from the complexity of quantum systems. The behavior of quantum particles can be incredibly intricate, exhibiting phenomena such as entanglement and superposition. Simulating these systems accurately requires immense computational resources, often exceeding conventional capabilities.
- Scalability: As the size of the quantum system increases, the resource requirements grow exponentially. Simulating a quantum system with just a few particles can be managed with classical computers; however, as more particles are added, the computations become infeasible quickly.
- Quantum State Representation: Representing quantum states efficiently is a critical bottleneck. Current classical approaches cannot accurately depict the superposition of states or the configurations in entangled systems without immense computational overhead.
- Algorithmic Limitations: The algorithms used in quantum simulations are still maturing. Many classical simulation techniques, like Monte Carlo methods, struggle with quantum properties, leading to potential inaccuracies in results.
These factors highlight the pressing need for innovations in both hardware and software to effectively advance quantum simulation capabilities.
Noise and Error Mitigation
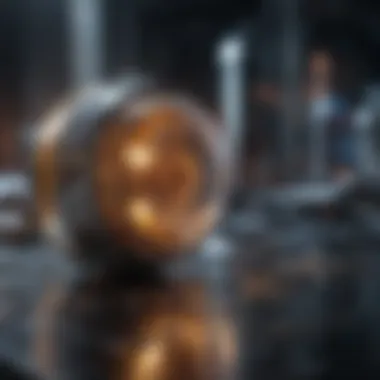
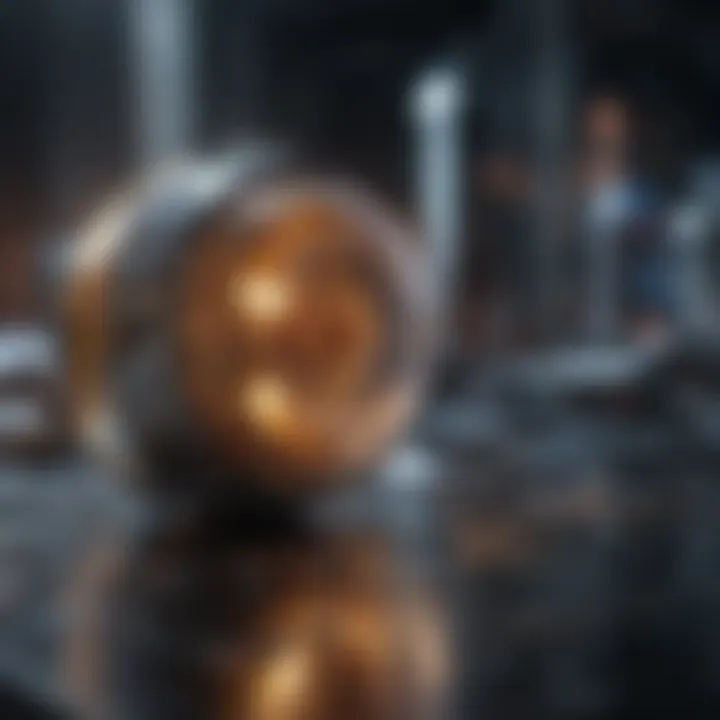
Another substantial challenge faced in quantum simulations is the issue of noise and error. Quantum systems are highly susceptible to environmental perturbations, which lead to decoherence. This is when quantum states lose their quantum characteristics due to interaction with the environment, and it fundamentally hinders accurate simulation.
- Error Rates: Quantum bits, or qubits, are prone to errors during operations. The fidelity of the quantum simulations can be greatly affected by these errors, necessitating error-correction strategies. High error rates pose obstacles in achieving reliable quantum results.
- Mitigation Strategies: Researchers are exploring various noise mitigation strategies. For example, techniques such as quantum error correction codes and dynamical decoupling are under investigation to enhance the robustness of quantum simulations.
- Stability In Algorithms: Developing algorithms that can maintain stability in the presence of noise is essential. Achieving stable algorithms will allow for better predictions and reliable outputs in simulations.
"Addressing noise and error in quantum simulations is not just about achieving accuracy; it is about enabling the technology to reach its full potential."
To sum up, overcoming challenges in quantum simulation is imperative. The obstacles of computational limitations and noise mitigation expose significant areas for research and development. As technology advances, the innovative approaches will bring forth better simulations, enabling new discoveries in both quantum physics and its applications.
Future Directions in Quantum Physics Simulation
The future directions in quantum physics simulation are critical for understanding how this field will evolve. As researchers continue to explore the boundaries of quantum mechanics, new opportunities arise for enhanced simulation techniques. These advancements are essential in maximizing the potential of quantum computations and ensuring that the results are practical and beneficial across various scientific fields.
Advancements in Quantum Hardware
Advancements in quantum hardware are pivotal to the development of quantum simulations. With new qubit technologies emerging, such as superconducting qubits and trapped ions, the efficiency and accuracy of quantum simulations are improving significantly. These technologies allow researchers to build more stable and scalable quantum systems.
Moreover, improvements in error correction techniques are helping to mitigate quantum noise, a common challenge faced in quantum simulations. As hardware becomes more robust, it leads to more reliable simulations of complex quantum systems. Researchers are also looking into integrated photonic systems, which offer the potential for high-speed quantum computations and enhanced communication protocols. This progress means that simulating intricate quantum phenomena may one day become routine.
Integration with Classical Systems
The integration of quantum and classical systems is another promising direction in quantum physics simulation. The interplay between these two realms can create hybrid models that leverage strengths from both sides. For instance, classical algorithms can be utilized for tasks that do not require quantum speedup, allowing quantum systems to focus on more complex challenges.
Incorporating artificial intelligence and machine learning tools into quantum simulations is paving the way for smarter algorithms that adapt and improve over time. This hybridization could lead to breakthroughs in computational efficiency, effectively solving problems that are currently intractable. The seamless integration of quantum and classical techniques can enhance simulation scope, leading to more comprehensive models in various applications, including materials science and drug discovery.
With these advancements, the future of quantum physics simulation seems optimistic. Researchers must remain aware of the ethical implications and ensure responsible usage of these powerful tools. "The advancements in quantum hardware and their integration with classical systems will likely transform how scientists approach simulations in the years to come."
Ultimately, the future directions of quantum physics simulation hold significant potential to drive scientific inquiry forward. Understanding the benefits and challenges inherent in these advancements will be crucial for students and professionals alike.
Ethical Considerations in Quantum Research
The exploration of quantum physics simulation has profound implications. As scientists dive deeper into this intricate field, the ethical considerations surrounding it become increasingly critical. These considerations shape the path of research and application, influencing how quantum technologies are developed and integrated into society. The importance of this topic lies in its potential to impact both scientific endeavors and societal well-being.
Key elements of ethical considerations include the responsible use of technology, implications for privacy, and the potential for misuse. As researchers advance quantum simulations, they must recognize their duty to engage with the ethical dimensions of their work. Here, we will examine two primary aspects: the impact on society and technology, alongside the scientific responsibility that stems from these innovations.
Impact on Society and Technology
Quantum physics simulation holds the promise of revolutionary advancements in numerous sectors, including computing, communication, and drug discovery. However, the ethical implications of these technologies cannot be overlooked. With great power comes great responsibility. The ability to manipulate quantum states can lead to innovations that enhance societal life, but it also presents risks that could disrupt social norms and individual rights.
- Privacy: As quantum technology develops, concerns arise regarding data privacy. Quantum computing has the potential to break existing encryption methods. This raises alarms around how data protection and individual privacy can be maintained in an era of powerful computational possibilities.
- Accessibility: There is also the question of access to quantum technologies. Unequal access can widen societal gaps, creating a divide where only a few benefit from advancements while others are left behind. Researchers must aim for inclusivity, ensuring that quantum advancements serve the greater good and are not restricted to wealthy individuals or corporations.
- Environmental Concerns: The environmental impact of deploying large-scale quantum systems can also be significant. Ethical research necessitates an assessment of sustainability practices, aiming to minimize the ecological footprint of these technologies.
"Ethics in quantum research is not just about compliance, but about fostering a responsible innovation culture."
Scientific Responsibility in Quantum Research
In addition to societal impacts, scientific responsibility plays a crucial role in the ethical landscape of quantum research. Researchers must adhere to high standards of integrity and accountability. This includes rigorous peer review and transparency in methodology and findings. The collaboration between scientists across various disciplines can help establish best practices that prioritize ethical considerations.
Moreover, the educational aspect of scientific responsibility is vital. Researchers should engage with the broader community, educating them on the implications of quantum technologies. This forms a dialogue that prepares society for changes that these technologies may bring. Additionally, fostering an environment of diverse perspectives, especially in governance discussions, can lead to more equitable outcomes.
- Developing Ethical Guidelines: Research institutions and regulatory bodies need to create clear ethical guidelines that govern quantum research and its applications. This will serve as a framework for navigating the complexities inherent in this field, ensuring careful consideration of the societal impacts.
- Engagement with Multidisciplinary Experts: By bringing in professionals from law, ethics, and sociology, the scientific community can adopt a more holistic approach to quantum research, addressing concerns that may not be rooted solely in scientific discourse.
- Open Dialogue: Establishing platforms for open dialogue between scientists and the public will help demystify quantum technologies and allow for public input in shaping policies that govern their use.
As we advance further into the realm of quantum physics simulation, a commitment to ethical reflection remains essential. The choices made today will shape the future landscape, impacting not only scientific advancement but also the very fabric of society.
Finale
The conclusion of this article serves a crucial role in synthesizing the intricate topics discussed throughout. It encapsulates the core insights into quantum physics simulation, building a bridge between theoretical concepts and their practical implications. Emphasizing the importance of quantum simulation facilitates a deeper understanding among students, researchers, educators, and professionals alike.
Summary of Key Insights
Quantum physics simulation is not merely a scientific endeavor, but a necessary tool in navigating the complexities of quantum mechanics. Several key insights arise from the exploration:
- Fundamental Principles: Understanding wave-particle duality, superposition, and entanglement is essential for anyone engaging with quantum simulations.
- Computational Techniques: The development of algorithms and computational methods illustrates an important transition from classical to quantum perspectives. The insights gained from these methods lie at the heart of modern innovations in technology.
- Real-World Applications: From materials science to pharmaceutical research and the burgeoning field of quantum computing, the implications of quantum simulation extend far beyond academic interest.
- Challenges Faced: Addressing computational limitations and mitigating noise in quantum systems remains a pressing concern, highlighting the necessity for ongoing research and development.
Overall, it is clear that quantum physics simulation represents a pivotal frontier in science and technology, driving advancements in numerous fields.
Final Remarks on Future Potential
Looking forward, the potential for quantum simulation continues to expand. As quantum hardware becomes more advanced, integrating quantum and classical systems will enhance simulation capabilities. Future research may also uncover new methodologies and applications, pushing the boundaries of our understanding.
The integration of quantum simulation techniques into various fields can revolutionize industries, create new technologies, and address global challenges.
In essence, the future of quantum physics simulation holds promise for significant advancements, making it a compelling area for inquiry and investment. Researchers and educators must continue to explore the dynamic and evolving landscape of quantum simulation, ensuring that they remain at the forefront of scientific discovery.