Understanding Quantum Physics: Mechanisms and Implications
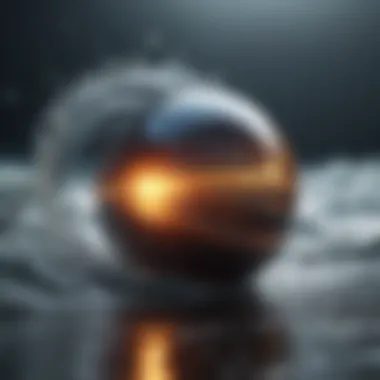
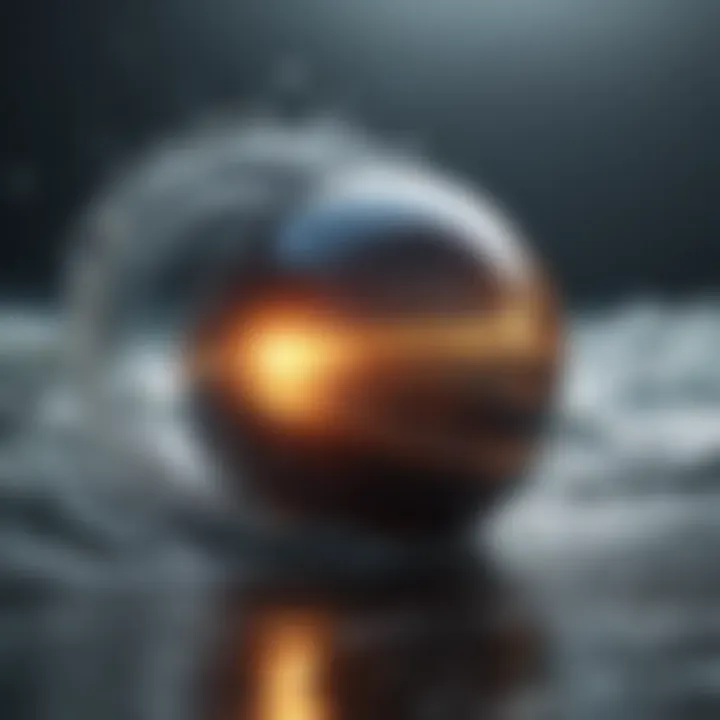
Intro
Quantum physics represents a revolutionary branch of science that explores the behavior of matter and energy at the smallest scales. At its core, it seeks to unravel the complexities of particles, waves, and fundamental forces that govern our universe. Given its intricate nature, quantum physics often challenges our conventional understanding of reality. This article aims to break down the fundamental principles of quantum physics, making it accessible to a wider audience while maintaining the depth essential for a proper comprehension.
By delving into topics such as wave-particle duality, the uncertainty principle, and quantum entanglement, readers will be equipped with the knowledge needed to explore how these concepts influence modern technology and scientific advancements. Whether you are a student, educator, researcher, or a professional in the field, this guide will enhance your grasp of the fascinating and often counterintuitive landscapes of quantum mechanics.
Key Research Findings
Overview of Recent Discoveries
Recent advancements in quantum physics have led to groundbreaking discoveries. Notably, experiments using photons have illustrated wave-particle duality in real-time, allowing scientists to observe particles behaving both as waves and discrete entities. Such findings have profound implications for the understanding of light and matter.
Moreover, developments in quantum computing hold the potential to revolutionize fields like cryptography, optimization, and machine learning. Researchers at IBM and Google continue to push the boundaries of practical quantum computing through algorithms designed to harness entanglement and superposition.
Significance of Findings in the Field
The significance of these findings lies not only in theoretical exploration but also in practical applications. Quantum technologies are no longer confined to laboratories; they are becoming integral to industries. For example, quantum sensors promise increased precision in measuring environmental changes. Such inventions could transform fields ranging from meteorology to medical imaging.
"Quantum physics is no longer just theoretical; it shapes the very technology we use daily."
As we continue to explore these findings, we realize their implications extend far beyond academia. The merging of quantum principles with everyday technology indicates a shift towards a future in which quantum mechanics could become a cornerstone of technological advancement.
Breakdown of Complex Concepts
Simplification of Advanced Theories
Dismantling complex theories in quantum physics is essential for clarity. For example, wave-particle duality suggests that particles can exhibit properties of both waves and particles, depending on the context of observation. This concept can be challenging to apprehend but can be simplified as follows:
- Wave Behavior: Particles can spread out and interfere with each other, similar to how water waves do.
- Particle Behavior: Particles can be detected as individual points, like small bullets, when measured.
The uncertainty principle, proposed by Werner Heisenberg, states that it is impossible to precisely know both the position and momentum of a particle. The more accurately one is measured, the less accurate the other becomes. This principle highlights fundamental limitations in our understanding of quantum systems.
Visual Aids and Infographics
Visual aids can significantly enhance the learning experience by illustrating intricate concepts. For instance, graphical representations of wave functions can depict how particles exist in overlapping states. Diagrams showing entangled states can clarify how particles remain interconnected across distances, defying classical intuitions of locality. Such resources can transform abstract theories into more tangible ideas.
In summary, quantum physics offers a fascinating glimpse into the building blocks of our universe. As we advance through this article, we will continue to unravel these theories and examine their applications in modern technology, reinforcing the relevance of quantum mechanics in today's scientific landscape.
Foreword to Quantum Physics
The realm of quantum physics represents a profound shift in understanding the workings of the universe at the smallest scales. It is not merely a collection of abstract concepts, but a body of knowledge essential for anyone engaged in modern scientific inquiry. As technology advances, the relevance of quantum physics continues to resound in various fields, from computing to healthcare.
By grasping the principles of quantum physics, one not only appreciates its theoretical aspects but also its practical applications. For example, innovations born from quantum mechanics have led to the development of advanced materials, quantum computing, and secure communication systems such as quantum cryptography. This article will explore these fundamental principles, providing a guidance to the intricacies of this fascinating field.
Defining Quantum Physics
Quantum physics, often referred to as quantum mechanics, studies matter and energy at atomic and subatomic levels. At this scale, classical physics fails to explain observed phenomena, necessitating a new framework. Throughout its history, quantum physics has defined critical concepts that contradict intuition, such as wave-particle duality and uncertainty.
Fundamentally, quantum physics introduces several essential elements. The notion of a quantum state represents a physical system's condition, encapsulated in terms of probabilities rather than certainties. This reflects a significant shift away from deterministic views prevalent in classical physics.
Historical Background
The historical development of quantum physics began in the early 20th century with significant contributions from numerous scientists. Max Planck's introduction of quanta in 1900 was a pivotal moment. His work sparked a reassessment of energy exchange principles and laid the groundwork for future discoveries.
Soon after, Albert Einstein expanded upon Planck's ideas, explaining the photoelectric effect. This contributed to the understanding of light as both a particle and a wave. Niels Bohr further refined atomic theory, proposing the quantized nature of electron orbits.
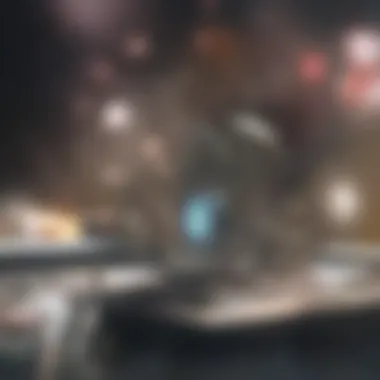
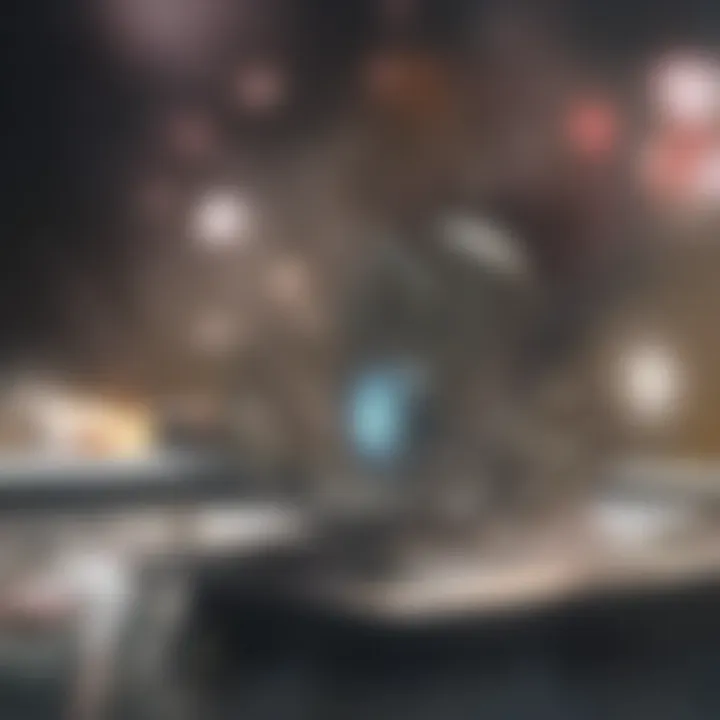
These milestones ushered in a new scientific paradigm, fundamentally changing how scientists perceive the universe. The development of quantum theory represented not only a scientific breakthrough but also profound philosophical implications regarding reality itself. These historical insights serve as a foundation for the subsequent exploration of quantum principles and their implications.
"Quantum mechanics provides a mathematical description of much of the dual particle-like and wave-like behavior and interactions of energy and matter."
Understanding quantum physics is not merely an academic exercise. It embodies the essence of our attempt to comprehend the intricate tapestry of reality, making it a crucial aspect of scientific discourse.
Core Principles of Quantum Physics
The core principles of quantum physics form the foundation of this fascinating field. Understanding these principles is crucial for grasping the complex behaviors observed at the quantum level. They challenge traditional perspectives on physics and introduce concepts that defy common sense. By examining these principles, readers can better appreciate the underlying mechanisms driving modern technologies such as quantum computing and cryptography.
Wave-Particle Duality
Wave-particle duality is one of the most intriguing concepts in quantum physics. It suggests that subatomic particles, like electrons and photons, exhibit both wave-like and particle-like properties. This duality can be seen in experiments, such as the famous double-slit experiment, where light behaves as a wave when not observed, yet acts like a particle when its path is being measured.
The significance of wave-particle duality extends beyond the laboratory. It impacts how we perceive light and matter, allowing for advancements in optics and quantum technologies. Understanding this duality is essential for anyone studying quantum mechanics, as it frames the interaction between energy and matter.
Quantum State and Superposition
A quantum state represents the complete information about a quantum system. Unlike classical states, which can exist in specific configurations, a quantum state can exist in multiple configurations simultaneously, a phenomenon known as superposition. This principle allows particles to occupy various states until they are measured, fundamentally altering how we understand particles and their behaviors.
Superposition has significant implications for quantum computing, where bits can exist in multiple states at once, dramatically increasing processing power. Researchers are still exploring its full potential, revealing possibilities for more efficient algorithms and solving complex problems that classical computers struggle with.
The Uncertainty Principle
The uncertainty principle, primarily formulated by Werner Heisenberg, states that certain pairs of physical properties, like position and momentum, cannot both be precisely known simultaneously. This principle disrupts the notion of determinism found in classical mechanics, suggesting instead that there is an intrinsic limitation to measurement at the quantum level.
This has profound implications that challenge our conventional views of reality. It leads to questions about the nature of existence and the reliability of observations within quantum systems. In practice, the uncertainty principle influences the development of technologies like electron microscopy and has sparked debates in both physics and philosophy, reshaping our approach to understanding the universe.
Quantum Theory and Its Components
Quantum theory serves as the cornerstone of our understanding of the micro world. Its components shape how we perceive fundamental concepts such as energy, matter, and the interrelations between them. Recognizing these elements is essential for grasping the deeper implications quantum physics holds for technology and philosophy.
In quantum physics, theory often shifts foundational ideas about determinism and certainty, embracing instead a conceptual framework that incorporates probability and complexity. This article section delineates the components that characterize quantum theory, laying the groundwork for comprehending more intricate discussions that follow.
Quantum Mechanics: An Overview
Quantum mechanics presents a scientific methodology for investigating the behavior of particles at the atomic level. It diverges significantly from classical physics, drastically altering our grasp of physical phenomena. Unlike classical mechanics, where objects follow predictable paths, quantum mechanics introduces elements of randomness and uncertainty.
In simpler terms, quantum mechanics suggests that particles, like electrons, do not have fixed positions or velocities but exist in a state of potential possibilities. When a measurement is conducted, the system 'collapses' to a particular state. This process highlights the importance of measurement in quantum physics, a theme we will explore further.
The Role of Probability in Quantum Mechanics
Probability is not just a tool in quantum mechanics; it is integral to the framework itself. Unlike classical systems, where outcomes can be predicted with certainty, quantum mechanics relies on probability distributions to describe the behavior of particles. The famous wave function, denoted by Ψ, encapsulates this probabilistic nature, representing a superposition of potential states.
- Complexity of Outcomes: Each measurement may yield a different result, making predictions challenging and fundamentally probabilistic.
- Interpreting Probability: The nature of probability in quantum mechanics leads to various interpretations, such as the Copenhagen interpretation or Many-Worlds interpretation. Each provides different insights into how to understand the probabilistic outcomes that emerge.
This reliance on probability challenges traditional views of determinism, fundamentally altering how scientists and theorists think about the universe.
Measurement Problems
The act of measurement in quantum mechanics raises a plethora of philosophical and practical questions. Measurement problems arise from the paradox of observation—how does observing a quantum system change its state? This question is central to quantum mechanics and introduces the concept of observer effect.
One major issue is:
- Wave Function Collapse: The concept suggests that upon measurement, a particle’s wave function collapses to a definite state, but exactly what constitutes a measurement remains vague.
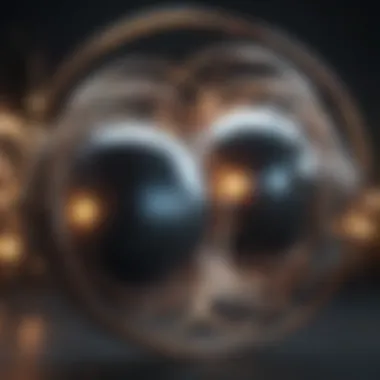
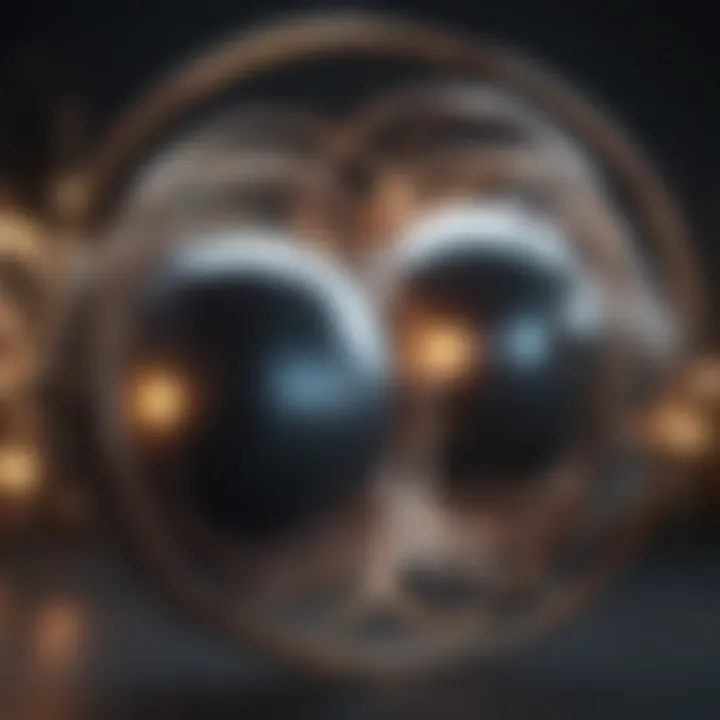
Furthermore, different interpretations of quantum mechanics offer various resolutions to these measurement problems, highlighting the field's ongoing debates.
"The measurement problem is not merely a technical issue but touches on the fabric of reality itself."
This complexity reveals not only the limits of our understanding but also marks a thrilling frontier for future research.
In summary, the components of quantum theory provide essential insights into the microscopic realm. From the overview of quantum mechanics to the pivotal role of probability and the intricacies of measurement issues, an appreciation for these concepts is vital for those engaging with quantum physics, be it from a scientific or philosophical standpoint.
Entanglement and Non-locality
Quantum entanglement and non-locality represent some of the most fascinating aspects of quantum physics. These concepts have significant implications for understanding the universe and the fundamental nature of reality. They challenge classical notions of separability and locality, opening new avenues of exploration in both theoretical and practical applications in modern science and technology.
Entanglement occurs when pairs or groups of particles interact in such a way that the state of one particle is directly related to the state of another, even when separated by vast distances. This relationship persists regardless of the space separating the particles, leading to what Einstein famously termed "spooky action at a distance." The intertwined states mean measuring one particle will instantaneously determine the state of its entangled partner, no matter how far apart they are.
Understanding Quantum Entanglement
At its core, quantum entanglement defies classical intuition. To grasp this concept, it is essential to understand that quantum states are not just simple properties but reflect a complex web of interconnections. When two particles are entangled, they form a singular quantum system despite being spatially separated. This unity suggests a holistic view of the universe.
Key features of quantum entanglement include:
- Instantaneous correlation: Changes in one particle's status have an immediate effect on the other.
- Non-locality: The entangled particles do not conform to the limitations of classical communication; they act as if they share a common state.
- Measurement effects: Observing one particle influences its partner, embedding the observer firmly within the quantum framework.
Entanglement has profound implications in fields such as quantum computing and quantum cryptography, where it serves as the backbone for many advanced processes. Understanding the mechanisms of entanglement leads to better comprehension of quantum systems at macroscopic scales and introduces opportunities for revolutionary technologies.
Implications for Communication and Computing
The implications of quantum entanglement extend beyond theoretical physics. It presents novel possibilities for communication systems and computational methods.
- Quantum Communication: Entangled particles can facilitate ultra-secure communication. In quantum key distribution, for instance, the principles of entanglement ensure that intercepted communications are detectable by the parties involved, thereby providing robust security.
- Quantum Computing: Entanglement is crucial for the operation of quantum computers, which rely on qubits that can represent multiple states simultaneously. Entangled qubits can perform complex calculations at speeds unattainable by classical computers, potentially solving problems that were previously considered intractable.
- Distributed Computing: With entangled systems, tasks can be distributed across multiple nodes without loss of coherence, enhancing the efficiency and capability of computational networks.
Entanglement is not merely a peculiar peculiarity of quantum mechanics. It plays a pivotal role in redefining our approach toward technology and information management, heralding an era where the principles of quantum mechanics transform practical applications, from enhanced security measures in communication to groundbreaking advancements in computational algorithms.
"Quantum entanglement deepens our understanding of the universe, revealing a complex interplay of connections that challenge our perception of reality and locality."
Applications of Quantum Physics
The influence of quantum physics extends beyond theoretical boundaries and finds substantial applications in various fields of technology and science. This section elaborates on the significance of these applications, discussing the mechanisms they introduce, the benefits they offer, and the considerations they entail. By bridging fundamental physics with practical use, quantum physics has the potential to revolutionize multiple industries.
Quantum Computing
Quantum computing represents one of the most profound applications of quantum physics. Unlike classical computers that use bits to process information, quantum computers leverage quantum bits, or qubits, which can exist in multiple states simultaneously due to superposition. This characteristic allows quantum computers to perform complex calculations at speeds unattainable by traditional computers.
The implications of quantum computing are vast. Here are a few noteworthy points:
- Increased Processing Power: Quantum computers can solve problems such as factorizing large numbers and optimizing complex systems more efficiently.
- Advanced Algorithms: Algorithms designed for quantum systems, like the Grover's algorithm, can significantly reduce the time taken to search large databases.
- Artificial Intelligence Enhancement: The complexity of machine learning algorithms can be tackled with quantum computing, leading to breakthroughs in various AI applications.
Despite these advantages, there are challenges. Developing stable qubits and maintaining coherence is an ongoing research priority. The error rates in quantum systems currently limit usability in real-world applications.
Quantum Cryptography
Quantum cryptography adds a new layer of security, primarily through a method known as quantum key distribution (QKD). This system uses quantum mechanics to secure data transmission. It guarantees that any attempt to eavesdrop will alter the system and alert the parties involved.
Key elements of quantum cryptography include:
- Unbreakable Security: The laws of quantum mechanics provide a secure way to transmit information, as any interference changes the quantum state and is detectable.
- Data Integrity: The encryption keys generated are unique to each session and cannot be reused, ensuring privacy for each communication exchange.
- Future Applications in Secure Communications: Quantum cryptography can protect sensitive information, such as financial transactions and personal data in communication networks.
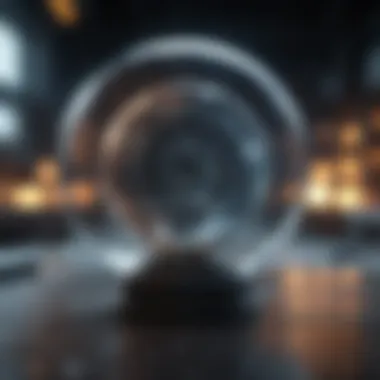
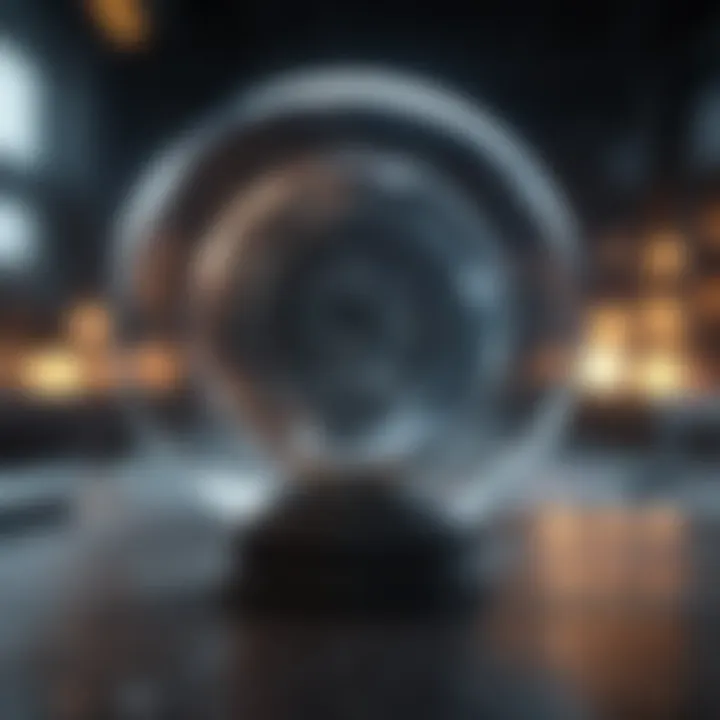
Nonetheless, the technology still faces scalability issues. Current QKD systems require special infrastructure, which can limit widespread adoption.
Medical Applications
Quantum physics plays a vital role in advancing the medical领域 through quantum imaging and quantum sensors. One primary application is in diagnostic imaging techniques. By utilizing quantum sensors, healthcare professionals can achieve improved sensitivity and resolution.
Key advantages include:
- Enhanced Medical Imaging: Techniques like magnetic resonance imaging (MRI) can become more precise, allowing for earlier detection of diseases.
- Targeted Drug Delivery: Quantum dots can be used to deliver drugs to specific areas in the body, minimizing side effects and increasing treatment effectiveness.
- Clinical Diagnostics: Quantum sensors can be used for detecting diseases with greater accuracy, supporting timely intervention.
The integration of quantum technology in medicine also raises ethical considerations. Discussions around data privacy and the implications of new medical technologies are ongoing.
The future of applications in quantum physics shines with potential. Each application emphasizes that understanding these principles is key to unlocking innovative solutions in diverse fields.
Challenges and Debates in Quantum Physics
The journey through quantum physics is not without its twists and turns. Understanding the challenges and debates surrounding this field is crucial for anyone delving into its mechanisms and implications. These challenges stem from the fundamental nature of quantum physics itself, which often contradicts our classical intuitions. Hence, the significance lies in how these issues lead to vibrant discussions and an ongoing quest for deeper insights.
Philosophical Implications
At the core of quantum physics lies a treasure trove of philosophical implications. These implications arise from the nature of reality as revealed by quantum phenomena. Concepts such as superposition and entanglement challenge conventional notions of causality and locality. The famous double-slit experiment illustrates this vividly. In it, particles display wave-like behavior, suggesting that they exist in multiple states simultaneously until measured. This raises a fundamental question: What is the role of the observer in defining reality?
Researchers and philosophers debate whether quantum mechanics implies that reality is inherently probabilistic or whether it merely reflects our limited understanding.
Key philosophical questions include:
- What does it mean for a particle to exist in multiple states?
- Is reality independent of observation?
- How do we reconcile quantum indeterminacy with a deterministic universe?
These questions invite scholars from various fields to explore the implications of quantum mechanics in ethics, metaphysics, and even the philosophy of science.
Ongoing Research and Discovery
Research in quantum physics is a continuously evolving landscape. Scientists are constantly exploring the boundaries of current understanding. The ongoing debates often lead to new lines of inquiry and experimental testing of quantum theories. Recent advancements in quantum technologies have sparked renewed interest in fundamental questions. For instance, quantum computing is not just an application of quantum mechanics; it also creates new challenges for theorists trying to understand its implications.
Ongoing research focuses on several areas, including:
- Quantum Information Theory: Understanding how information behaves at the quantum level.
- Quantum Gravity: Investigating how quantum mechanics interacts with gravitational forces.
- Interpretations of Quantum Mechanics: Exploring alternative views, such as Many-Worlds or Copenhagen interpretations, offers different insights into reality.
Ongoing discoveries are reshaping our understanding, leading to breakthroughs that could redefine technology and its impact on society. As new experiments yield data, they often fuel further debate, challenging previous assumptions and unearthing new questions.
In the realm of quantum physics, every answered question opens doors to new inquiries. Each discovery reignites discussions that challenge our grasp of the universe.
In summation, the challenges and debates in quantum physics highlight its intricate nature and the necessity for continued research. By examining these elements, we find not only a deeper understanding of quantum mechanics but also a reflection of the very essence of scientific inquiry.
Ending
The concluding section of this article serves a crucial role in synthesizing the vast array of insights presented throughout. Reflecting on the key concepts discussed, it reaffirms the profound significance of quantum physics in our understanding of the universe. In this section, we will recap the major principles and highlight the ongoing relevance in both theoretical and practical applications.
Recap of Major Concepts
Quantum physics entails several groundbreaking ideas that challenge traditional perceptions of reality. These ideas include:
- Wave-Particle Duality: This principle suggests that particles can act both as particles and waves. The behavior of light, as seen with photons, exemplifies this duality, impacting fields ranging from optics to quantum computing.
- Quantum State and Superposition: The idea of superposition allows particles to exist in multiple states simultaneously until measured. This concept is essential in quantum computing as it underlines how quantum bits, or qubits, function.
- The Uncertainty Principle: Formulated by Werner Heisenberg, this principle indicates that certain pairs of physical properties, like position and momentum, cannot be simultaneously known to arbitrary precision. It has deep implications for measurements and predictions in quantum systems.
- Quantum Entanglement: This phenomenon illustrates a connection between particles such that the state of one immediately influences the state of another, regardless of the distance separating them. The potential for using entanglement in secure communications is a topic of active exploration.
These concepts provide a framework through which we understand various quantum phenomena and their implications for technology and philosophy.
Future Directions in Quantum Research
Quantum physics is an ever-evolving field. The future will likely reveal even deeper insights into the mechanisms governing the quantum world. Notable areas for future research include:
- Quantum Computing Advances: Continued development in quantum theories promises to revolutionize computing capabilities. Researchers are working on creating more stable qubits and scalable quantum systems.
- Quantum Cryptography: There is significant ongoing work in developing secure communication methods through quantum principles, such as quantum key distribution. This may enhance digital security in multiple applications.
- Exploring the Foundations of Quantum Mechanics: Philosophical inquiries into the meanings and implications of quantum mechanics continue to challenge scientists and thinkers. Understanding the nature of reality and information through the lens of quantum theories remains an intriguing endeavor.
Ultimately, the exploration of quantum physics not only fuels technological advancements but also broadens human understanding of nature itself. As we push further into this complex landscape, the dialogue between science and philosophy will play a fundamental role in shaping future hypotheses and discoveries.
"Quantum physics is not just a theory; it is the foundation of the modern scientific era."
In summary, the journey through quantum mechanics elucidates not only its theories but its profound influence across various fields, prompting us to continually reassess our understanding of the universe.