Exploring the Fundamentals of Quantum Physics
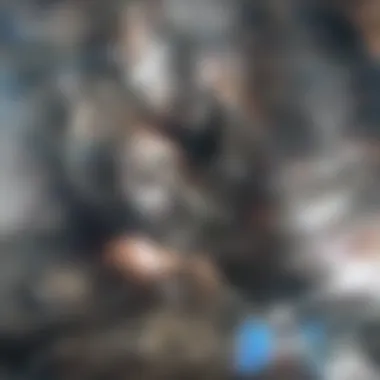
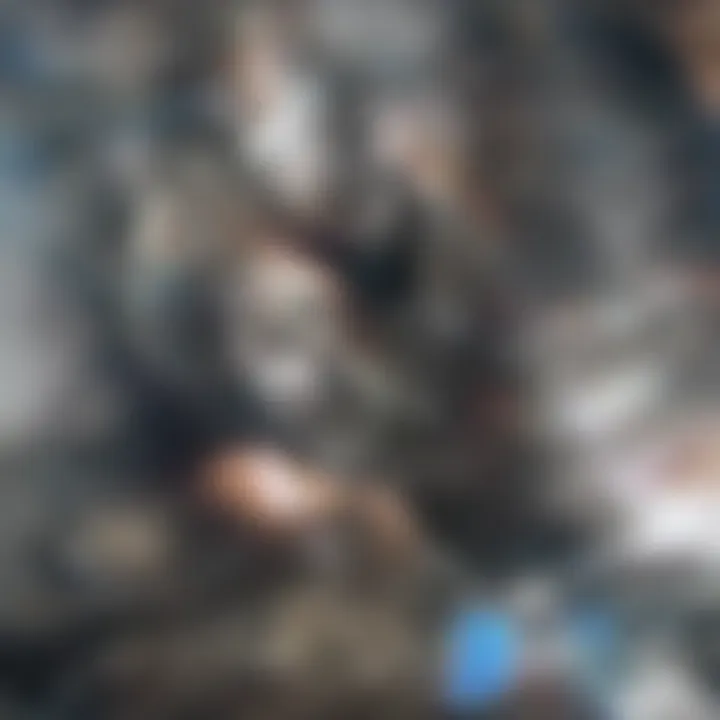
Intro
Quantum physics stands as a pivotal cornerstone of modern physics, characterized by its unique principles that govern the behavior of matter and energy at the smallest scales. Understanding quantum physics opens doors to intriguing possibilities that reshape our perception of reality.
As we delve into this topic, we will explore essential concepts such as superposition, entanglement, and wave-particle duality. The implications of these principles extend far beyond theoretical implications, affecting technology in ways previously thought unimaginable. This article aims to provide a structured overview that balances complexity with clarity, making it accessible for students, researchers, and professionals alike.
In the following sections, we will examine key research findings, breakdown complex concepts, and reflect on how these elements have contributed to the ongoing evolution of quantum theory.
Preamble to Quantum Physics
Quantum physics stands as a pivotal realm of study, a foundation that influences our comprehension of the natural world. This section outlines its significance and delves into fundamental aspects that motivate a deeper understanding of this complex field.
Understanding quantum physics is crucial for various reasons. First, it reshapes our perception of reality. At the atomic and subatomic levels, particles behave in ways that contradict everyday experiences. This deviation from classical physics principles prompts scientists and students alike to rethink longstanding concepts of matter and energy.
Moreover, quantum physics is integral to a slew of technologies that have transformed modern life. From semiconductors to medical imaging devices, its principles underpin many innovations. Grasping the basics of quantum theory can thus enhance appreciation for these advancements, encouraging deeper inquiry into science and technology.
Finally, highlighting the historical development of quantum theory provides critical insights into its evolution. Understanding how early 20th-century physicists like Max Planck and Albert Einstein contributed to the field helps contextualize current theories and experiments. The journey of quantum discovery enhances one's literary pursuit within this complex domain and stimulates further intellectual curiosity.
Historical Context
The origins of quantum physics can be traced back to the late 19th and early 20th centuries. During this time, scientists grappled with the limitations of classical physics. In 1900, Max Planck proposed the concept of quantization, suggesting that electromagnetic energy could only be emitted or absorbed in discrete units, or quanta. This was a radical departure from established paradigms.
Subsequent developments included Albert Einstein's work on the photoelectric effect in 1905. He proposed that light itself is quantized in packets called photons. This finding laid the groundwork for wave-particle duality, a fundamental principle of quantum mechanics.
The early comprehension of atomic structure further propelled the development of quantum physics. Niels Bohr's model of the hydrogen atom in 1913 presented a new framework, showing that electrons exist in quantized orbits. As the field evolved, contributions from physicists such as Werner Heisenberg and Erwin SchrΓΆdinger further deepened our understanding of quantum mechanics.
Defining Quantum Physics
Quantum physics, at its essence, is the study of matter and energy at the most fundamental level. It seeks to explain phenomena observed at atomic and subatomic scales, often challenging classical notions of physics. Key aspects of quantum theory include concepts such as quantization, superposition, and entanglement.
Quantization refers to the discrete nature of energy levels in atoms and subatomic particles. Unlike classical systems where energy can range continuously, quantum systems exhibit specific energy states, which can be described mathematically.
Superposition, another core principle, posits that quantum systems can exist in multiple states simultaneously until measured. This leads to unique outcomes upon observation, fundamentally differing from classical determinations.
Entanglement further exemplifies quantum oddities. It describes a scenario where particles become interconnected, such that the state of one particle immediately influences the state of another, regardless of distance. This concept has profound implications for quantum communication and information transfer.
Understanding these definitions is vital for grasping advanced topics in quantum mechanics and their applications in technology and research.
The Core Principles of Quantum Mechanics
The core principles of quantum mechanics provide a foundational framework for understanding the behavior of matter and energy at the smallest scales. These principles are both counterintuitive and enlightening, leading to revolutionary changes in physics and technology. In this section, we explore key concepts such as quantum states, superposition, entanglement, and wave-particle duality. Each of these ideas contributes to the overall structure and function of quantum mechanics, shedding light on phenomena that classical physics fails to explain.
Quantum States and Superposition
In quantum mechanics, a quantum state describes the condition of a quantum system. This state contains all pertinent information about the system, from energy levels to probable position. Quantum states embrace the concept of superposition, where a particle can exist in multiple states simultaneously until measured.
This idea challenges the classical view of definite states. For example, an electron can spin both up and down at the same time rather than being confined to one state alone. The mathematical representation of superposition is typically done using wave functions, which capture the probabilities of finding a system in particular states upon observation.
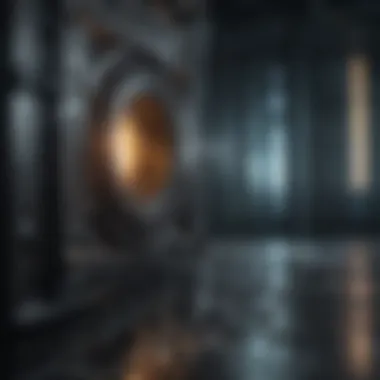
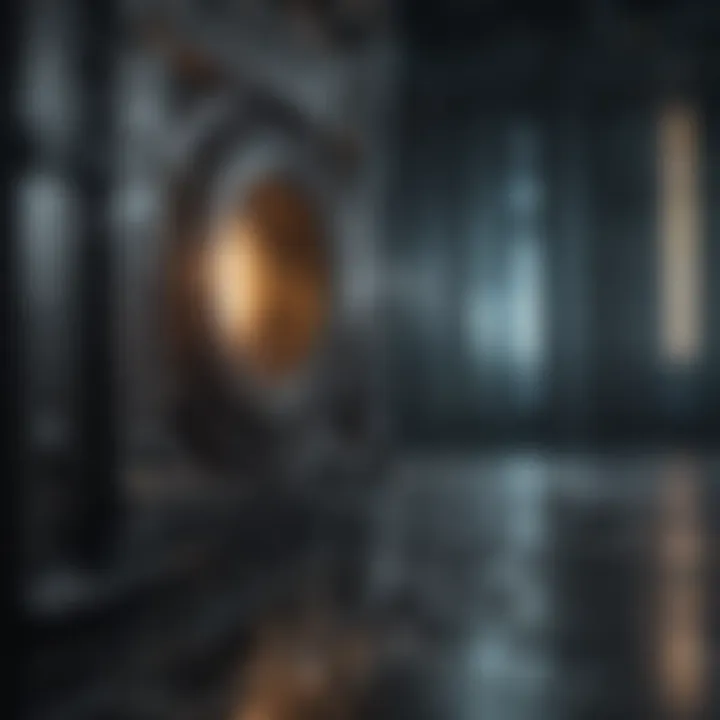
The superposition principle implies that, until observed, particles can exist in a vast array of states, making quantum systems inherently probabilistic rather than deterministic.
Quantum Entanglement
Quantum entanglement represents another cornerstone of quantum mechanics. When two or more particles become entangled, the state of one particle is directly correlated with the state of another, irrespective of the distance between them. This interconnection leads to fascinating implications about information and communication.
Entanglement poses questions about locality and the speed of information transfer. Albert Einstein famously referred to it as "spooky action at a distance" due to the instantaneous connection between entangled particles. The recent developments in quantum computing and cryptography heavily rely on entangled states, demonstrating their practical importance for technology.
Wave-Particle Duality
Wave-particle duality illustrates the dual nature of quantum objects, which exhibit both particle-like and wave-like properties. This characteristic is best exemplified in experiments such as the double-slit experiment, where light can behave as a wave creating interference patterns or as a particle when measured.
The implications of wave-particle duality extend into various fields including optics, quantum field theory, and even philosophy. Understanding this duality is critical for grasping quantum behavior and its applications in modern physics.
In summary, the core principles of quantum mechanicsβquantum states and superposition, entanglement, and wave-particle dualityβoffer crucial insights into the nature of reality. These concepts not only challenge traditional ways of thinking but also form the basis for innovations in technology and our understanding of the universe.
Mathematical Foundations of Quantum Physics
Mathematics forms the backbone of quantum physics. This discipline employs sophisticated mathematical structures to describe physical phenomena with remarkable precision. Understanding these mathematical foundations is essential for anyone delving into quantum mechanics.
The use of mathematics in quantum physics serves various purposes. First, it allows for the formulation of complex theories in a concise manner. Second, it provides the tools necessary for making predictions that can be experimentally tested. Key elements within this mathematical framework include wave functions, operators, and equations, which, together, outline the behavior of quantum systems.
Wave Functions
Wave functions, typically denoted by the Greek letter psi (Ξ¨), encapsulate the state of a quantum system. They carry essential information about the probabilities of finding a particle in various positions and states. The square of the wave function's absolute value indicates the likelihood of locating a particle within a particular region of space.
These wave functions play a crucial role in the calculation of other quantum properties. They allow scientists to glean insights into the behavior and interactions of subatomic particles. As such, understanding wave functions is fundamental to grasping quantum mechanics. The mathematical representation of a wave function can be complex, but its physical meaning is more straightforward: it reflects our knowledge and uncertainties about a quantum system.
Operators and Observables
In quantum mechanics, physical properties such as position, momentum, and energy are described by operators. An operator is a mathematical construct that acts on the wave function to extract information about an observable quantity. For instance, the position operator acts on a wave function to yield spatial properties of a particle.
The relationship between observables and operators is essential for interpreting measurements in quantum mechanics. When a measurement is made, the corresponding operator is applied to the wave function, producing eigenvalues that represent the possible outcomes of that measurement. This framework not only assures a structured approach to the mathematical foundations of quantum physics but also ensures consistency with experimental observations.
The SchrΓΆdinger Equation
The SchrΓΆdinger equation is a pivotal component of quantum mechanics. It describes how wave functions evolve over time. This equation is fundamentally a partial differential equation, which can seem daunting at first glance. However, it encapsulates the heart of quantum dynamics.
The time-dependent SchrΓΆdinger equation showcases how the state of a quantum system changes with time. On the other hand, the time-independent SchrΓΆdinger equation applies to systems in static states, allowing for the determination of energy levels. Each solution to the SchrΓΆdinger equation yields a wave function, furthering our understanding of quantum systems.
Significant Experiments in Quantum Physics
Significant experiments in quantum physics stand as pivotal milestones that shaped our understanding of the quantum realm. They validate theoretical principles, open avenues for technological advancements, and illuminate the perplexities that quantum mechanics introduces into our perception of reality. These experiments are not mere curiosities; they are foundational to the framework of quantum physics itself, serving to reinforce or challenge existing theories and assumptions.
The Double-Slit Experiment
The double-slit experiment is arguably one of the most famous experiments in quantum mechanics. First conducted by Thomas Young in the early 19th century, it dramatically reveals the strange nature of light and matter. In this experiment, a coherent source of light is shone at a barrier with two slits. On the other side, a detector screen records the pattern of light that emerges.
What is fascinating is that when both slits are open, an interference pattern appears on the screen, indicative of wave behavior. However, if one attempts to observe which slit a particle passes through, the interference pattern disappears. Instead, particles behave like particles would, showing two distinct bands corresponding to the two slits. This challenge to classical intuition raises questions about the nature of reality and observation in quantum physics.
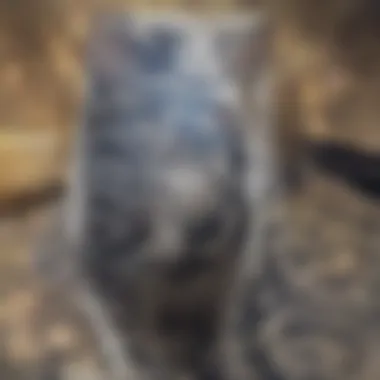
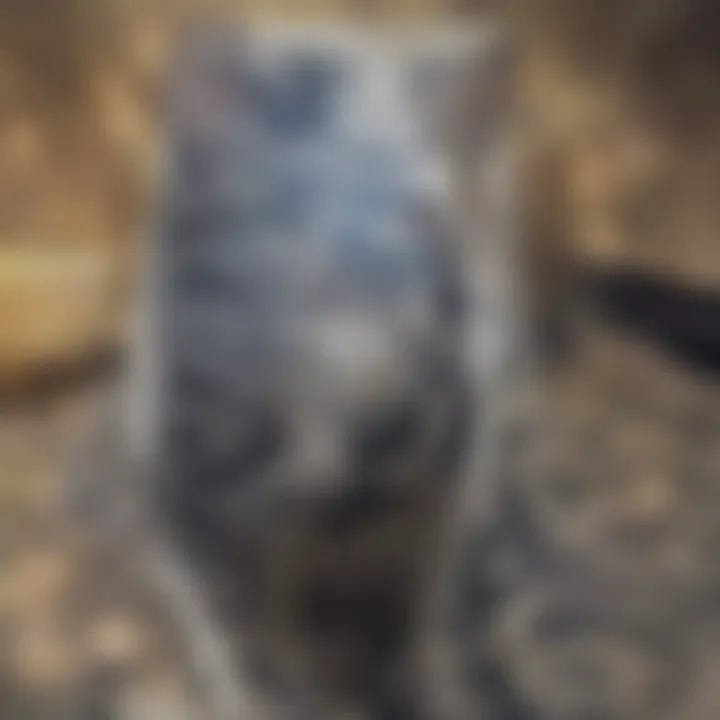
Quantum Tunneling
Quantum tunneling is another essential phenomenon that underscores the non-intuitive aspects of quantum mechanics. In classical physics, a particle like an electron requires sufficient energy to overcome a barrier. However, quantum mechanics introduces the concept that particles can pass through potential barriers, even if they lack the necessary energy to surmount them.
This occurs because particles do not have definite positions until they are measured. Their wave functions, which provide a probability distribution for their location, can extend into regions that are classically forbidden. Quantum tunneling has profound implications, not only in theoretical physics but also in practical applications, such as nuclear fusion and the functioning of devices like tunnel diodes and scanning tunneling microscopes.
Bell's Theorem and Experiments
Bell's theorem represents a significant theoretical advancement in quantum physics that leads to experiments testing the nature of entanglement. Proposed by physicist John Bell in the 1960s, it highlights the contradictions between quantum mechanics and local hidden variable theories.
Numerous experiments, notably those by Alain Aspect in the 1980s, have tested Bell's inequalities. The outcomes consistently support the predictions of quantum mechanics, suggesting that entangled particles can exhibit correlations that defy classical explanations, reinforcing the idea of non-locality inherent in quantum theory. This has deep implications for our understanding of causality, locality, and the nature of reality.
Quantum Teleportation
Quantum teleportation is a concept that stretches the boundaries of our understanding of communication. It is not about physically transporting matter but rather about transferring quantum information. This phenomenon relies on two key principles: quantum entanglement and the use of classical information channels.
In an experiment involving two entangled particles, the state of one particle can be measured, and this information can be used to reconstruct the state of the other particle. This has been demonstrated in various instances, sparking exciting discussions about the future of quantum communication and secure information transfer. Quantum teleportation has implications for the development of quantum computers and cryptographic systems that could redefine security in digital communication.
The experiments conducted in quantum physics challenge our understanding of reality and have vast implications in technology and philosophy.
Weaving together all these experiments, we see a rich tapestry that exemplifies the depth of quantum physics. Each experiment reveals the complexities and nuances of the quantum world, contributing not only to scientific knowledge but also to philosophical conversations regarding the nature of reality.
Quantum Physics and Technology
Quantum physics has become fundamental in reshaping modern technology. The principles derived from quantum mechanics now underlie various innovative fields, leading to groundbreaking developments that stretch the limits of current scientific understanding. With real-world applications emerging from theories previously thought to be purely academic, quantum physics bridges the gap between observation and advanced technological implementation.
One significant area of interest is quantum computing. Unlike classical computing, which relies on bits as the most basic unit of information, quantum computing utilizes quantum bits, or qubits. This shift enables computers to perform multiple calculations at once due to superposition, drastically enhancing computational power. Research suggests that quantum computers could solve complex problems, such as optimization and simulation of quantum systems, at speeds unattainable by traditional computers. The potential benefits of quantum computing include:
- Increased Processing Power: Tackling problems in fields ranging from cryptography to material science.
- Efficiency in Data Analytics: Providing new insights from vast sets of data.
- Revolutionized Machine Learning Techniques: Enabling algorithms that learn and adapt faster than classical methods.
Another crucial aspect is quantum cryptography, which leverages the principles of quantum mechanics to create security protocols. It addresses the inadequacies of conventional encryption methods. By using quantum key distribution (QKD), communication becomes nearly unhackable. The security of QKD stems from the fundamental laws of quantum physics, making eavesdropping detectable. Key points on quantum cryptography include:
- Privacy Assurance: Guarantees secure communication due to the principles of quantum mechanics.
- Future Security: Protecting sensitive data against potential quantum computing attacks in the future.
- Wide Applicability: Useful in finance, defense, and sensitive health records management.
Furthermore, quantum sensors present another layer of innovation. They utilize quantum states to achieve unprecedented levels of sensitivity and accuracy. This capacity makes them valuable in various domains, including
- Medical Imaging: Enhancing the quality and speed of diagnostic imaging.
- Navigation Systems: Providing precision beyond traditional GPS.
- Environmental Monitoring: Detecting changes in the environment at minute levels.
"The advent of quantum technologies is not just about scientific breakthroughs; it is shaping critical infrastructure for the future."
Philosophical Implications
Quantum physics extends beyond the confines of traditional physics, urging us to reassess our understanding of reality. The philosophical implications of this discipline are profound and multi-faceted, raising questions that have intrigued thinkers for generations. In this section, we will explore the significance of these implications, focusing on how they challenge our perception of existence and knowledge.
Interpretations of Quantum Mechanics
There are numerous interpretations of quantum mechanics, each attempting to explain the peculiarities of quantum phenomena. The most well-known are the Copenhagen interpretation, many-worlds interpretation, and pilot-wave theory. The Copenhagen interpretation posits that quantum particles exist in a state of probability until measured. This challenges classical determinism, suggesting that outcomes are not predetermined. In contrast, the many-worlds interpretation implies that every quantum decision leads to separate, branching realities, fundamentally altering the view of choice and consequence.
These interpretations do not simply offer different ways of understanding quantum mechanics; they also inform broader philosophical debates. For example, if realities branch into myriad outcomes, what does this mean for subjective experience and free will? As our grasp of quantum mechanics evolves, so too does our philosophical landscape, urging scholars to re-evaluate concepts that were once taken for granted.
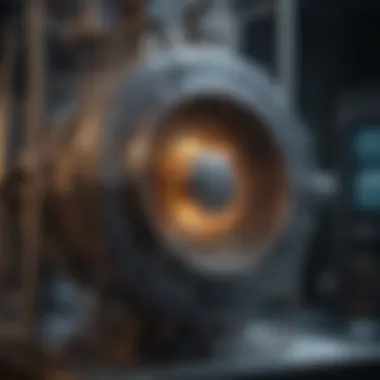
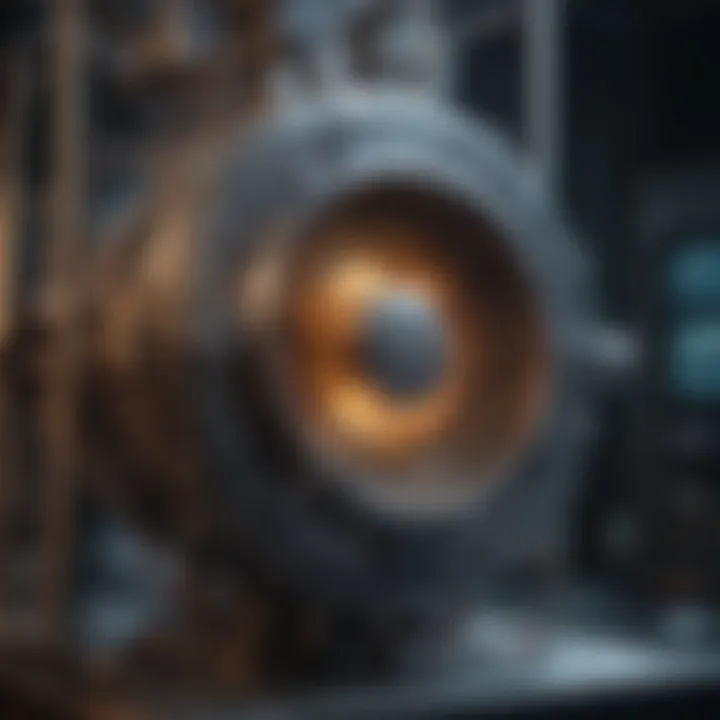
The Role of the Observer
In quantum mechanics, the observer plays a crucial role, prompting philosophic inquiry into the nature of observation itself. The act of measurement appears to influence the behavior of quantum particles, a phenomenon illustrated by the double-slit experiment. This raises the question: does reality exist independently of observation? The observer effect suggests that consciousness may shape physical reality, challenging the objective stance held by classical physics.
Philosophers and scientists alike have debated this aspect, leading to theories that argue for an inherent link between consciousness and the physical universe. This intertwining has implications for our understanding of human cognition and existence, suggesting that our consciousness may be more intimately connected to reality than previously thought.
Reality and Determinism
Quantum physics introduces a level of uncertainty and randomness that disrupts classical notions of determinism. Traditionally, many Western philosophies are rooted in the belief that the universe operates under fixed laws, leading to predictable outcomes. However, the probabilistic nature of quantum mechanics challenges this notion.
"If quantum mechanics hasn't profoundly shocked you, you haven't understood it yet." - Niels Bohr
This uncertainty raises essential philosophical questions: Is the universe inherently chaotic, or is our lack of understanding merely a limitation of our knowledge? The debate regarding determinism invites a crucial examination of concepts like causality, purpose, and fate. While some may argue that quantum randomness introduces chaos, others might see it as a gateway to a more complex and interconnected understanding of the fabric of reality.
In summary, the philosophical implications of quantum physics are vast. The interpretations of quantum mechanics push us to reconsider the framework of reality, while the role of the observer introduces an existential dimension to our understanding of consciousness. Finally, the shift from determinism to probability challenges the very foundations of how we view the universe. Engaging with these ideas can enrich not only our scientific perspectives but also our broader philosophical discussions.
Current Trends and Future Directions
Current trends in quantum physics reflect a dynamic phase of research and innovation. Understanding these trends is essential, as they shape the future landscape of both theoretical and applied physics. There are several key elements driving the advancement of quantum research, and recognizing them offers insight into how the field may evolve.
Emerging Research Areas
One prominent area of exploration is the field of quantum computing. Scientists are working on developing qubits, the building blocks of quantum algorithms. Various techniques, such as using superconducting circuits and trapped ions, show promise in creating functional quantum computers. This research is gaining momentum due to the potential for quantum computers to solve problems deemed intractable for classical computers.
Additionally, quantum information science is emerging as a critical research area. The ability to utilize quantum states for information processing and communication has vast implications. This includes advancements in quantum cryptography, where secure communication channels are established by leveraging quantum key distribution protocols. Researchers are increasingly interested in ensuring the security of information in an age where data breaches are commonplace.
Quantum Biology
Quantum biology is an interdisciplinary field that investigates the role of quantum mechanics in biological processes. This area gained attention due to observations suggesting that phenomena such as photosynthesis and bird navigation may be influenced by quantum coherence. Understanding these mechanisms can reveal how organisms leverage quantum behavior, which could lead to breakthroughs in bioenergy and even drug development. Furthermore, this intersection between biology and quantum mechanics opens new avenues in research, underscoring the need for a collaborative approach among scientists across various disciplines.
Interdisciplinary Applications
The integration of quantum physics with other scientific fields is another trend worth noting. This interdisciplinary approach fosters collaboration between physicists, chemists, engineers, and computer scientists to explore new applications of quantum mechanics. For instance, innovations in materials science are emerging by applying quantum mechanical principles to develop advanced materials with tailored properties. Researchers are investigating phenomena such as quantum tunneling to engineer materials with applications in various industries, including electronics and biotechnology.
Moreover, quantum sensors are gaining traction. These sensors utilize quantum states to achieve sensitivity levels far beyond classical sensors. Potential applications range from precise measurements in geological surveys to improved medical imaging techniques. As research continues to unfold, new ways to harness such sensors may revolutionize existing practices in various fields.
"The future of quantum physics is a collaboration of diverse fields, enhancing our understanding of natural processes and leading to transformative technologies."
Overall, the importance of current trends and future directions in quantum physics cannot be overstated. As research continues to unveil the mysteries of quantum mechanics, the implications for technology and interdisciplinary studies are profound. By investing in emerging research areas, the scientific community is not only expanding knowledge but also paving the way for innovations that may dramatically alter our understanding of the universe.
Closure
The conclusion serves as a critical juncture in the exploration of quantum physics. It synthesizes the main themes and insights presented throughout the article while reinforcing the significance of quantum principles in various domains. The careful articulation of key concepts enables readers to review their understanding and reflect on the implications of quantum mechanics.
Summarizing Key Concepts
In this article, we navigated essential tenets of quantum physics, from the nature of quantum states to the implications of entanglement. Various experiments paved the way for these concepts, such as the double-slit experiment, which vividly illustrates wave-particle duality. Moreover, we discussed mathematical tools like wave functions and the SchrΓΆdinger Equation that underpin quantum theory. This summary emphasizes that quantum physics is not merely a niche area of study; it forms the basis of many technological advancements today.
The Significance of Quantum Physics
The significance of quantum physics extends beyond academic interest. It transforms our understanding of reality, leading to practical innovations across technology and science. For instance, quantum computing promises unprecedented processing power, while quantum cryptography seeks to redefine data security. Furthermore, the philosophical implications challenge our perception of causality, urging debates on determinism and the observer's role in measurement. As such, the insights gathered from quantum physics are not only intellectually stimulating but also pivotal in shaping future advancements.
"Quantum mechanics is an essential framework for understanding the universe and continues to provoke critical thoughts about many facets of existence."
In summary, recognizing the essential concepts and the broader significance of quantum physics ensures that we grasp its fundamental role in the modern scientific landscape. With ongoing research and interdisciplinary applications emerging, the relevance of quantum physics only continues to grow, making it crucial to remain informed and engaged in this fascinating field.