Understanding Quantum Physics: A Comprehensive Exploration
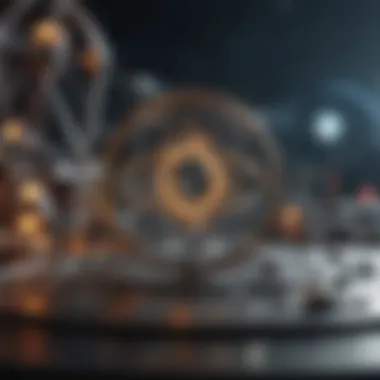
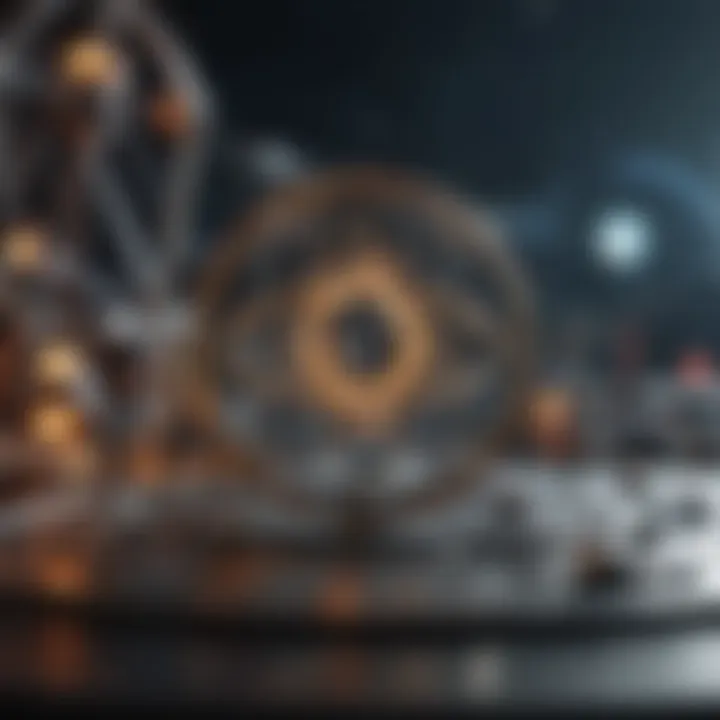
Intro
Quantum physics is a fascinating field that invites inquiry into the very nature of reality. This branch of physics challenges our classical notions of matter, energy, and the interactions between them. By exploring the behavior of particles at a quantum level, we gain insight into complex phenomena that often seem counterintuitive. Understanding quantum physics is not only crucial for physicists and researchers but also for anyone interested in the underlying principles that govern the universe.
In this article, we aim to unpack the foundational ideas, delve into the historical context, and explore the practical implications of quantum theory. This journey will cover significant discoveries, breaking down complex concepts into manageable parts. We will also examine how quantum physics impacts technology, philosophy, and broader societal perspectives.
By painting a comprehensive picture, this exploration will cater to students, educators, and science enthusiasts seeking a deeper appreciation of quantum physics.
Prelude to Quantum Physics
Quantum physics is not just another field of science; it fundamentally changes our understanding of the universe. It delves into the behavior of matter and energy at the smallest scales, uncovering principles that seem counterintuitive but are consistently validated through experiments. Understanding quantum physics is crucial as it informs various contemporary technologies and philosophical discussions regarding the nature of reality itself.
To grasp quantum physics, one must first define its core aspects and contextualize its development through history. The pursuit of quantum knowledge allows us to achieve measurable advancements in fields like computing and cryptography, as well as reshape philosophical debates about determinism and reality.
Definition and Scope
Quantum physics, or quantum mechanics, is the branch of physics that addresses phenomena occurring at atomic and subatomic levels. Unlike classical physics, which adheres to deterministic principles, quantum physics integrates the concepts of probability and uncertainty. This has broad implications for our understanding of physical laws, information theory, and the fundamental fabric of reality.
The scope of quantum physics extends beyond simple particle interactions. It encapsulates phenomena like wave-particle duality, entanglement, and superposition. Each of these concepts challenges conventional wisdom and invites us to rethink how we perceive the universe.
Historical Context
The Birth of Quantum Theory
The birth of quantum theory can be traced back to the early 20th century, sparked by a series of experiments that defied classical theories. One critical moment was Max Planckβs introduction of quanta, which revolutionized energy emission and absorption concepts. This idea led to the formulation of the photoelectric effect, later explained by Albert Einstein. The introduction of quantized states of energy was a quantum leap forward, allowing scientists to describe atomic behavior with unprecedented accuracy. This shift paved the way for new research avenues and technological advancements, such as lasers and semiconductors.
Key Figures and Milestones
Key figures in the development of quantum physics, including Niels Bohr, Werner Heisenberg, and Erwin SchrΓΆdinger, were instrumental in establishing its foundational principles. Bohr's model of the atom, Heisenberg's uncertainty principle, and SchrΓΆdinger's wave mechanics all contributed richly to the quantum lexicon. Each milestone not only enhanced scientific knowledge but also impacted technology and philosophy profoundly.
These developments underscore the collaborative nature of scientific inquiry, demonstrating how individual contributions culminate in a broader understanding of quantum realities. Their unique perspectives highlighted the interplay between particle behavior and measurement, driving innovations in both theory and application.
Importance in Contemporary Science
The importance of quantum physics in contemporary science cannot be overstated. Its principles are embedded in technologies we utilize daily, such as lasers, MRI machines, and modern electronics. Furthermore, quantum physics has spurred the development of quantum computing and cryptography, promising unprecedented processing capabilities and secure communication lines.
In the philosophical realm, quantum physics forces us to grapple with questions about determinism, reality, and our understanding of consciousness. The implications reach far beyond physical theories, influencing how we perceive our existence in the universe. Its impact is a reminder that science is not just a collection of facts; it shapes our understanding of existence itself.
Fundamental Concepts of Quantum Physics
In the study of quantum physics, understanding its fundamental concepts is pivotal. These concepts form the basis for much of modern physics and have implications that reach far beyond theoretical discussions. They guide research in various fields, including material science, electronics, and even philosophy.
Grasping these ideas helps students and researchers appreciate the complexities of nature at a microscopic level. This section will delve into three fundamental concepts: wave-particle duality, quantum superposition, and quantum entanglement. Each of these concepts not only illustrates the peculiar nature of quantum phenomena but also highlights how they influence the technological advancements of our time.
Wave-Particle Duality
Wave-particle duality is a cornerstone of quantum mechanics. This principle asserts that every particle or quantum entity can exhibit both wave-like and particle-like properties. For instance, light behaves as a wave when it diffracts and creates interference patterns. Conversely, it exhibits particle-like behaviors when it is absorbed or emitted in discrete packets called photons.
This duality is critical for understanding phenomena such as the photoelectric effect, where light striking a metal surface can eject electrons. Albert Einstein explained this effect, demonstrating that light has dual characteristics.
The significance lies in how this concept challenges traditional views of physics, forcing a reevaluation of how we perceive particles and waves. It's not just an academic topic; this principle underlies modern technologies like lasers and semiconductors.
Quantum Superposition
Quantum superposition is another vital concept in quantum physics. It refers to the ability of a quantum system to exist in multiple states simultaneously until it is measured. This phenomenon is often illustrated through the thought experiment known as SchrΓΆdinger's cat, where a cat in a sealed box is both alive and dead until someone opens the box to observe it.
The implications of superposition are profound. They challenge classical intuition and suggest that reality is far more complex than it appears. Quantum superposition enables technologies such as quantum computing, where qubits can represent multiple states at once. This characteristic offers vast potential for processing power and efficiency compared to traditional binary systems.
Quantum Entanglement
Quantum entanglement is perhaps the most striking concept in quantum physics. It describes a situation where two or more particles become interconnected in such a way that the state of one particle instantly influences the state of another, regardless of the distance separating them. This phenomenon puzzled even Einstein, who famously referred to it as "spooky action at a distance."
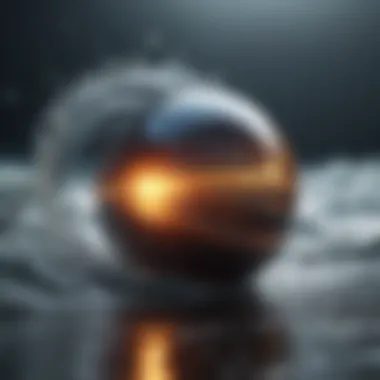
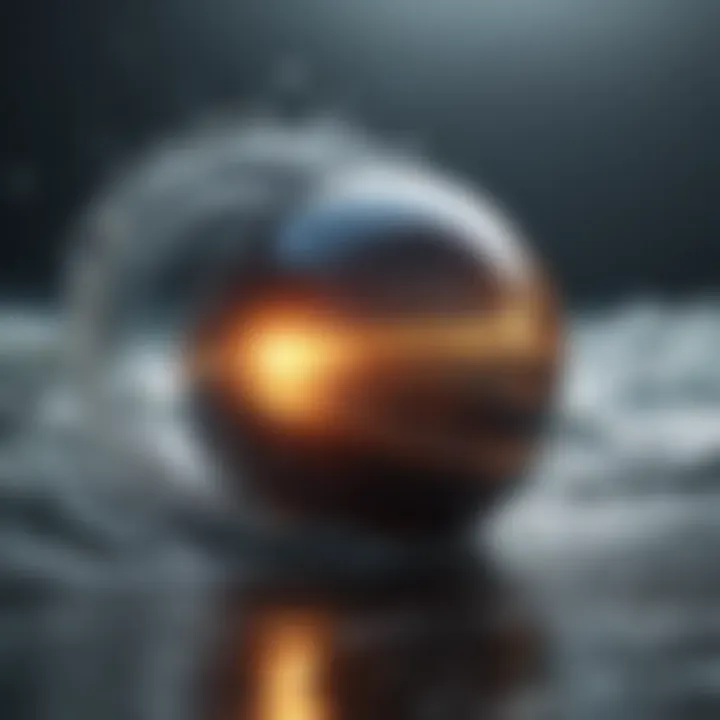
Entanglement plays a crucial role in various applications, particularly in quantum cryptography. It allows for secure communication that is theoretically immune to eavesdropping. The implications for quantum networks are immense, leading to advancements in secure data transmission across vast distances.
In summary, the fundamental concepts of quantum physics reveal a complex and fascinating view of reality. Understanding wave-particle duality, quantum superposition, and quantum entanglement is essential not only for academic exploration but also for their applications in technology and our daily lives. As research progresses, these concepts continue to shape our understanding of the universe.
Key Principles Governing Quantum Physics
The exploration of quantum physics hinges upon several key principles that reveal the underlying nature of the microscopic world. These principles do not only challenge traditional notions of physics but also foster innovative technologies and broaden our understanding of reality. The significance of these principles lies in their ability to articulate phenomena that are central to quantum mechanics. A nuanced grasp of these principles equips students, researchers, educators, and professionals with the critical insights needed to navigate the complexities of quantum theory.
Heisenberg Uncertainty Principle
The Heisenberg Uncertainty Principle asserts a fundamental limit to the precision with which certain pairs of physical properties, such as position and momentum, can be simultaneously known. This principle is key in quantum physics because it introduces the notion that at the quantum scale, measuring one quantity will inherently disturb the other. In other words, the act of observation affects what is being observed.
Mathematically, the principle can be expressed as:
[ \Delta x \cdot \Delta p \geq \frac\hbar2 ]
Where (\Delta x) is the uncertainty in position, (\Delta p) is the uncertainty in momentum, and (\hbar) is the reduced Planck's constant.
This inherent uncertainty leads to several philosophical implications, including debates on determinism and the nature of reality. The principle also affects technologies like quantum computing, where uncertainty in states can enable more complex computations.
Quantum Tunneling
Quantum tunneling refers to the phenomenon where a particle can pass through a potential energy barrier despite not having enough energy to overcome it classically. This principle is crucial in explaining processes such as nuclear fusion and electron behavior in semiconductors.
Tunneling is applied in various technologies, including:
- Tunnel Diodes: These are semiconductor devices that utilize tunneling to achieve high-speed performance.
- Scanning Tunneling Microscopes: These allow imaging of surfaces at the atomic level by measuring tunneling current.
The implications of quantum tunneling extend beyond mere theoretical interest. They play a substantive role in the development of quantum technologies, including quantum computing and advanced materials.
Pauli Exclusion Principle
The Pauli Exclusion Principle states that no two fermions (such as electrons) can occupy the same quantum state within a quantum system simultaneously. This principle is foundational to understanding the structure of atoms and the behavior of matter.
Consequences of the Pauli Exclusion Principle include:
- The arrangement of electrons in atoms, determining chemical properties.
- The stability of matter, preventing atoms from collapsing under their own gravitational pull.
- The formation of complex structures like solid materials, as it explains why electrons fill orbitals in a specific order.
Understanding the Pauli Exclusion Principle is essential for exploring various fields, from chemistry to material science. Consequently, the principle forms a bedrock for comprehending the behavior of particles in condensed matter and significantly influences research and technology development.
The key principles of quantum physics not only elucidate the mechanics of atomic and subatomic particles but also provide a groundwork for revolutionary technologies that shape our future.
In summary, the principles governing quantum physics reveal profound insights. They challenge conventional wisdom, inspire future technology, and necessitate a re-evaluation of our understanding of reality. Embracing these principles allows us to explore new frontiers in both science and philosophy.
Mathematics of Quantum Physics
The field of quantum physics heavily relies on mathematical frameworks to describe and predict the behavior of quantum systems. Mathematics serves as the language through which the principles of quantum mechanics are articulated. This section emphasizes the critical role that mathematics plays in understanding quantum physics, focusing on key areas such as state representation, operators, and the synthesis of quantum mechanics with linear algebra.
Quantum State Representation
Wave Functions
Wave functions are fundamental in quantum mechanics. They provide a description of the quantum state of a particle or system. The wave function is typically represented by the Greek letter psi (( \psi )), and it contains all the information about a system's properties, such as position and momentum. The key characteristic of wave functions is their probabilistic nature, allowing for the calculation of the likelihood of finding a system in a specific state at a given time. This probabilistic approach is crucial for comprehending the inherent uncertainties present in quantum systems.
One of the unique features of wave functions is their ability to represent complex systems, which allows scientists to predict the behavior of particles at a subatomic level. The advantages of employing wave functions include their adaptability in handling various scenarios and providing a comprehensive overview of a system's dynamics. However, a disadvantage is their abstract nature, which may limit intuitive understanding for those not well-versed in advanced mathematics.
Quantum Numbers
Quantum numbers further enrich the representation of quantum states. They serve as sets of numerical values that define the quantum states of electrons in an atom. Quantum numbers describe particular properties, such as energy levels, angular momentum, and magnetic orientation. A key characteristic of quantum numbers is their discrete nature, implying that certain properties can only exist in specific quantized states.
The primary advantage of quantum numbers is their utility in organizing and classifying quantum states, making it easier to understand atomic and subatomic phenomena. However, the unique feature of these numbers lies in their specific nature; for instance, each electron in an atom is described by a unique set of quantum numbers. This can lead to complexities when dealing with multi-electron systems, where interactions can yield unforeseen behaviors.
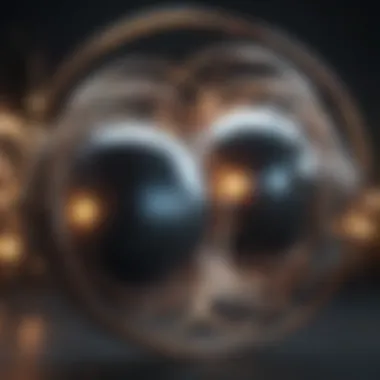
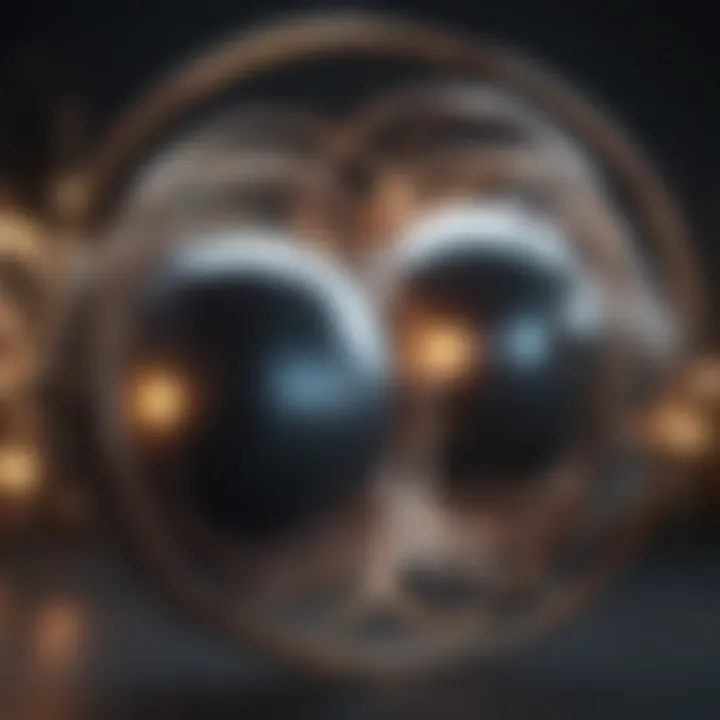
Operators and Observables
Operators in quantum physics are mathematical constructs used to represent observable physical quantities. Each observable, such as position, momentum, or energy, is associated with a corresponding operator. The application of these operators to wave functions reveals the expected values of the observables. For example, the momentum operator acts on a wave function to yield information about the momentum of the particle. Understanding operators is essential, as they are fundamental to making predictions and interpreting measurements in quantum mechanics.
Operators illustrate how measurements influence quantum states, highlighting the importance of precise mathematical interpretation in quantum experiments.
Quantum Mechanics and Linear Algebra
The relationship between quantum mechanics and linear algebra cannot be overstated. Quantum states can be represented as vectors in a complex vector space, while operators can be conceived as matrices acting on these vectors. This linear algebra foundation allows for the application of familiar mathematical techniques, which can simplify complex problems and provide insights into quantum behavior. The use of eigenvectors and eigenvalues in this context is particularly noteworthy, as they enable the analysis of measurable quantities and their respective outcomes in quantum systems. Through these mathematical structures, quantum mechanics reveals its intricate nature and strengthens its connection to applied mathematics.
Applications of Quantum Physics
The applications of quantum physics manifest in many fields, reflecting its profound influence on modern technology, security, and scientific inquiry. The implications of these applications cannot be overstated. Understanding them is crucial not only for researchers but also for individuals keen on how quantum science shapes their daily lives. The core elements of quantum physics introduced earlier find their real-world expressions in several groundbreaking technologies.
In essence, the key areas of application can be categorized into three main categories: quantum computing, quantum cryptography, and quantum sensing. Each application harnesses the unique properties of quantum mechanics to solve complex problems that classical techniques cannot effectively address.
Quantum Computing
Quantum computing signifies a paradigm shift in information technology. Unlike classical computers that use bits as the basic unit of information, quantum computers utilize qubits. These qubits can exist in multiple states simultaneously due to quantum superposition. This ability to represent more complex data enables quantum computers to perform calculations far more efficiently than their classical counterparts.
The potential benefits of quantum computing are immense. They include:
- Enhanced Computational Power: Quantum computers can tackle complex problems in fields like cryptography, chemistry, and logistics that would take classical computers thousands of years.
- Optimization: Businesses can leverage quantum algorithms for optimization problems, leading to better resource allocation and decision-making.
However, significant challenges remain. Building stable quantum computers is technically demanding. Issues like decoherenceβwhere quantum states lose their coherence due to environmental interactionsβare ongoing subjects of research.
Quantum Cryptography
Quantum cryptography presents a revolutionary approach to secure communication. It relies on principles of quantum mechanics to protect data. Notably, Quantum Key Distribution (QKD) allows parties to share encryption keys securely. The famous BB84 protocol exemplifies this, ensuring that any eavesdropping attempts can be detected.
Key points about quantum cryptography include:
- Absolute Security: The laws of physics ensure that the act of observing a quantum system changes it. This principle provides a security measure that classical cryptography cannot offer.
- Practical Challenges: While the theory is robust, practical implementation is still unfolding. Infrastructure for widespread adoption is being developed but is not yet universally accessible.
Quantum Sensing
Quantum sensing utilizes quantum mechanics to achieve measurement precision beyond classical limits. Quantum sensors operate by exploiting phenomena such as entanglement and superposition. These sensors can measure gravitational waves or magnetic fields with unprecedented accuracy.
Important aspects of quantum sensing are:
- Medical Applications: Tools like quantum magnetometers may improve medical imaging techniques, enhancing diagnosis accuracy.
- Environmental Monitoring: Quantum sensors can detect minute changes in the environment, aiding in climate change research and disaster preparedness.
Philosophical Implications of Quantum Physics
The philosophical implications of quantum physics are profound and significant. This field challenges our traditional notions of reality, observation, and determinism. Understanding these implications contributes to a broader comprehension of how quantum physics interacts with our perception of existence. Here, we discuss two critical sub-components: reality and observation, and the debate between determinism and probability.
Reality and Observation
In quantum physics, the act of observation plays a pivotal role. The famous thought experiment known as SchrΓΆdinger's cat illustrates this concept well. In this scenario, a cat is both alive and dead until someone opens the box to observe its state. This leads us to ponder the nature of reality itself. Is something real only when it is observed? This question forces us to reconsider how we define existence.
Quantum mechanics suggests that particles exist in a state of superposition, a blend of all possible states, until observed. This inherent uncertainty opens the door to critical reflections on epistemology, which is the study of knowledge and belief. A few essential points emerge from this discussion:
- Dependent Reality: Reality might depend on the observer's presence.
- Collapse of States: Observation collapses the probabilistic wave function into a definite state, redefining what we consider 'real.'
- Subjective Influence: An observer's choices could influence outcomes, suggesting a deep connection between consciousness and matter.
"The act of observation fundamentally alters the reality of what is observed."
Determinism vs. Probability
The clash between determinism and probability forms another philosophical cornerstone in quantum physics. Classical physics operates on deterministic principles, asserting that if we know the current state of a system, we can predict its future state accurately. However, quantum mechanics introduces an inherent probabilistic nature to events. Key ideas include:
- Heisenberg Uncertainty Principle: This principle asserts that certain pairs of physical properties cannot be simultaneously known to arbitrary precision. For example, knowing an electron's position means we cannot know its momentum exactly.
- Randomness of Events: At the quantum level, outcomes can only be predicted in terms of probabilities. This stands in stark contrast to classical views of a predictable universe.
- Philosophical Consequences: These uncertainties point towards a universe that is not fully deterministic, raising questions about free will and the nature of causality.
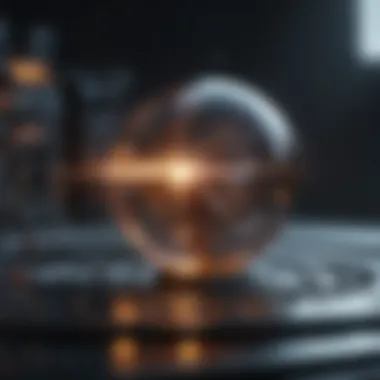
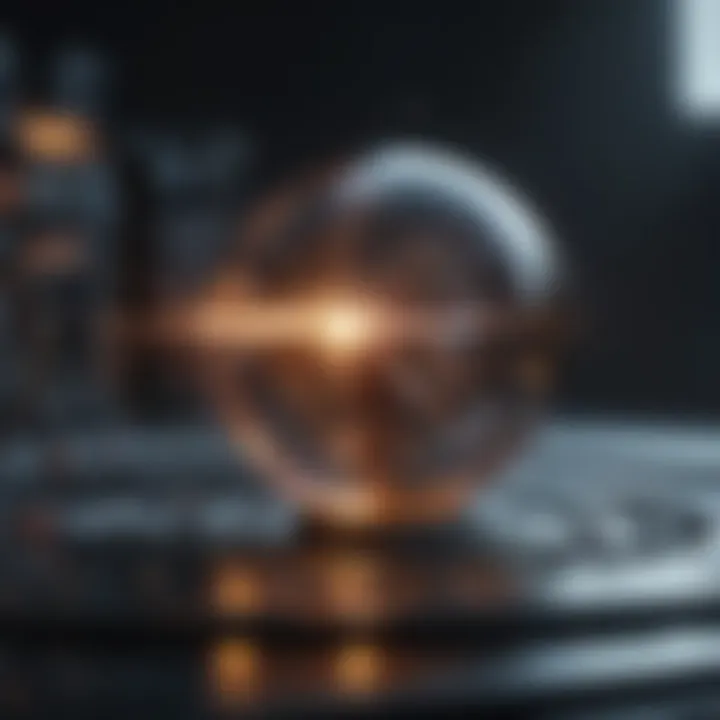
Current Research in Quantum Physics
Current research in quantum physics serves as a crucial avenue for understanding and expanding the frontiers of modern science. This area of study is not static; it evolves significantly thanks to new discoveries and theoretical advancements. By delving into current research, one can gain insights into both the potential and limitations of quantum theories. This section details the key topics in this ongoing exploration, particularly focusing on emerging theories and the challenges that accompany them.
Emerging Theories
In the realm of quantum physics, several emerging theories are capturing the attention of researchers. Among them, quantum gravity stands out as a pivotal undertaking aimed at unifying general relativity and quantum mechanics. Researchers are trying to understand how these two leading theories of physics can be reconciled. Another area of interest is quantum information theory, which encompasses the study of information processing within quantum systems. This intersection of quantum mechanics and information theory opens avenues for groundbreaking advancements in computing and cryptography.
Furthermore, string theory is yet another speculative yet intriguing approach. It posits that the fundamental particles of the universe are not point-like dots but rather tiny vibrating strings. Each vibration corresponds to a particle's mass and charge.
- The implications of such theories often extend beyond physics, touching on topics in philosophy, mathematics, and even cosmology.
Researchers are also exploring many-worlds interpretation, which suggests that all possible outcomes of quantum events are realized in separate, parallel realities or universes. These new theories generate excitement but also require rigorous scrutiny to identify their validity and implications for our conceptual understanding of reality.
Challenges and Controversies
As intriguing as these new theories can be, they are not without their challenges and controversies. One major issue is the lack of experimental validation for many emerging theories. For example, string theory's reliance on higher dimensions has made it difficult to derive testable predictions. This lack of empirical evidence can lead to skepticism among the scientific community.
Moreover, the interpretation of quantum mechanics itself continues to be a contentious subject. The question of whether wave functions represent actual reality or merely our knowledge of systems remains unresolved. This debate complicates the roadmap for future experiments and theoretical work.
- Ethical implications also arise. As quantum technologies develop, issues related to privacy, security, and economic impact emerge. These factors add another layer of complexity that researchers must navigate.
Ultimately, the pursuit of knowledge in quantum physics is not just about uncovering truths; it involves grappling with the profound implications these truths might bring to society. As researchers continue to explore these challenges and theories, they contribute to the framing of future scientific paradigms.
"Theories are not just a logical construction; they are profoundly tied to our understanding of the universe."
In summary, ongoing research in quantum physics provides a vast landscape ripe for exploration. Emerging theories present exciting possibilities but also confront us with significant challenges. Understanding and addressing these aspects is essential for any meaningful progression in the field.
Future Prospects of Quantum Physics
The future prospects of quantum physics represent a fascinating frontier within contemporary science. This intricate field holds the potential to transform technology, alter perceptions of reality, and challenge our existing frameworks of understanding. As researchers delve deeper into the principles guiding quantum mechanics, they unlock new opportunities that extend beyond traditional applications. This section discusses technological innovations emerging from quantum physics and the broader societal impacts these advancements might entail.
Technological Innovations
Technological advancements within quantum physics are paving the way for a new era of innovation. Here are some critical areas where quantum physics is making strides:
- Quantum Computing: Quantum computers utilize the principles of quantum mechanics to process information in ways classic computers cannot. They promise to perform complex calculations at unprecedented speeds and with greater efficiency. Google and IBM are leading projects in this quest, presenting significant milestones for quantum supremacy, where a quantum computer outperforms the most powerful classical computers.
- Quantum Communication: Security in communication is crucial in today's digital world. Quantum cryptography offers groundbreaking methods for secure data transmission. The uniqueness of quantum states can ensure that any attempt to eavesdrop on communication will interfere with the data itself, making unauthorized access detectable. Companies such as ID Quantique are already trialing these technologies.
- Quantum Sensors: These devices employ quantum phenomena to measure physical quantities with a higher degree of precision than their classical counterparts. Developments in fields like gravitational wave detection and timekeeping devices demonstrate the potential of quantum sensors for scientific advances.
Technological innovations in quantum physics not only enhance existing technologies but also cross-pollinate various fields such as materials science, pharmaceuticals, and energy. This rapid evolution signifies that the practical applications of quantum mechanics are just beginning to be explored.
Broader Impacts on Society
The societal implications of advancing quantum physics are profound. As quantum technologies permeate everyday life, they will disrupt industries, influence economic structures, and shape global interactions. Here are several areas affected by these changes:
- Healthcare: Quantum techniques can lead to revolutionary breakthroughs in medical imaging and drug discovery. The ability to analyze complex biological systems at a quantum level may yield better diagnostic tools and targeted therapies, hence improving patient care.
- Economy: Quantum computing is expected to create industries that do not exist today. Startups focusing on quantum applications will grow, leading to new job markets and transforming existing sectors like finance and telecommunications. Greater efficiency in computations will also enhance decision-making processes in business.
- Ethical Considerations: With powerful tools come responsibilities. As quantum technologies become mainstream, ethical concerns will arise regarding privacy, data security, and the potential for misuse. Societies will need to actively engage in discussions about the regulations and limitations surrounding these advanced technologies.
"The ongoing evolution of quantum physics will challenge existing paradigms, not just scientifically, but also ethically and socially."
Through understanding and addressing these broader impacts, societies can better prepare for the transition into a quantum-enabled future. This proactive approach will be crucial in maximizing benefits while mitigating risks associated with these transformative technologies.
Culmination
The conclusion of this article synthesizes the essential themes and findings discussed in the previous sections about quantum physics. It serves to reinforce the significance of quantum mechanics not only as a field of study but as a framework that underpins much of modern science and technology. This section offers a concise recap of core concepts, coupled with reflections on the ongoing research that continues to evolve within the discipline.
Recap of Key Concepts
At the heart of quantum physics lie several foundational ideas, each contributing to our understanding of the microscopic world. Important concepts such as wave-particle duality, which denotes that particles like electrons exhibit both wave- and particle-like properties, reveal the complexity of nature at quantum scales. Additionally, quantum superposition suggests that systems can exist in multiple states simultaneously until measured, fundamentally challenging classical notions of individuality and certainty. The entanglement phenomenon further complicates our understanding of separability and locality. All these principles illustrate how quantum physics diverges from intuitive perception.
Furthermore, several essential principles, including the Heisenberg Uncertainty Principle, emphasize the intrinsic limits of measurement and observation. Such principles form a core part of quantum mechanics and contribute to discussions surrounding determinism and causality. Thus, the recap not only highlights the intricate nature of physical phenomena but also underscores the fundamental shifts in our perception of reality prompted by quantum theories.
The Ongoing Nature of Quantum Research
The realm of quantum physics is far from static. Continuous research opens up new avenues for exploration and a deeper comprehension of the universe. Current endeavors aim to address burgeoning challenges and controversies within the field. Studies are ongoing into emerging theories, such as quantum gravity and interpretations of quantum mechanics that could further clarify our understanding of reality itself.
Quantum computation and cryptography represent prominent fields where research is intensely focused, promising technological innovations that could reshape many aspects of our daily lives. As institutions and collaborations increase in number, the implications of these advancements will only broaden, engaging various sectors in society.
In summary, the conclusion emphasizes that quantum physics is more than a theoretical framework; it is a dynamic and evolving field. As research progresses, its implications will reverberate throughout technology, philosophy, and our understanding of reality. The continuous exploration in quantum physics not only illuminates the nature of existence but also challenges students, researchers, and professionals to ponder the fabric of the universe.