Understanding Quantum Physics: Concepts and Applications
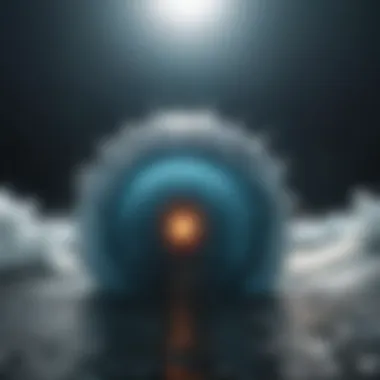
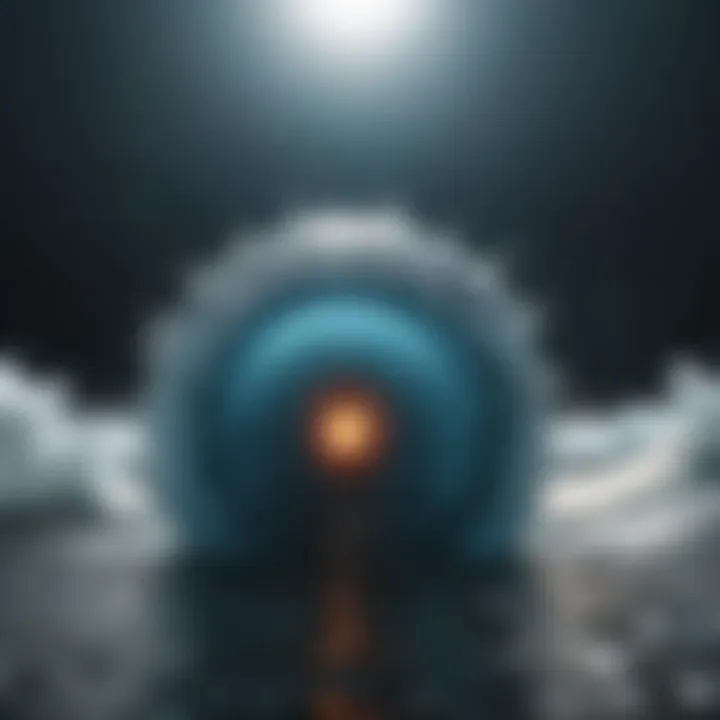
Intro
Quantum physics, often seen as a realm shrouded in complexities, offers insights that challenge conventional thinking. This domain is not just confined to the lab; its implications echo in our everyday lives and philosophical perspectives. To navigate the intricacies of quantum mechanics, it’s essential to begin with the groundwork—understanding foundational principles, historical milestones, and how they influence contemporary technologies.
Throughout this article, we will peel back the layers of quantum physics. We will dissect concepts like wave-particle duality and explore the enigmatic nature of quantum entanglement. As we journey through these topics, the ultimate aim is to translate dense theories into digestible explanations, making the world of quantum physics more approachable for students, researchers, educators, and professionals alike.
In essence, we are embarking on a quest for clarity in a field where uncertainty reigns supreme. By linking scientific principles to philosophical reflections, we seek to spotlight the relevance of quantum physics in our understanding of reality. The discussions here aim to illuminate not just how these quantum principles operate but also what they mean for the fabric of our universe.
Foreword to Quantum Physics
Quantum physics sits at the forefront of modern science, unraveling the mysteries of the universe's smallest constituents. As we step into the intricate world of subatomic particles and their behaviors, one realizes the significance of this field stretches beyond academic curiosity; it is foundational to much of today's technological advancements. From the workings of semiconductors in our smartphones to the principles behind emerging quantum computers, the implications are as vast as they are profound.
By diving into topics such as the particle-wave duality of electrons or the puzzling phenomenon of quantum entanglement, we see how traditional views of physics are challenged. Understanding quantum physics helps not just scientists but also the general audience appreciate how interconnected and complex our world really is.
Defining Quantum Physics
Quantum physics, in its essence, is the branch of physics that deals with the behavior of matter and energy at the smallest scales — the atomic and subatomic levels. Unlike classical physics, which describes a deterministic universe, quantum mechanics introduces a level of probability and uncertainty. It paves the way for understanding how particles can exist in multiple states at once and how their interactions can lead to outcomes that defy everyday logic.
Historical Context and Development
The journey of quantum physics is marked by a series of groundbreaking discoveries and theories that reshaped our conception of reality. Two pivotal figures in this historical narrative are Max Planck and Albert Einstein, whose work laid the groundwork for what would eventually become quantum mechanics.
Max Planck and Black-Body Radiation
Max Planck's work is often the cited turning point in physics. His introduction of the concept of quantization emerged from his studies of black-body radiation. Planck proposed that energy is not emitted or absorbed continuously but rather in discrete units, or 'quanta.' This idea was revolutionary and deviated sharply from the classical physics paradigm.
The significance of Planck's theory can’t be overstated. It illuminated the understanding of thermal radiation, which classical theories failed to fully explain. In this context, Planck's constant became a vital component of quantum theory, allowing for more precise descriptions of energy levels. It brought forth a wave of new ideas that eventually influenced not just physics but also chemistry, material science, and engineering.
Albert Einstein and the Photoelectric Effect
Another critical milestone came with Albert Einstein's exploration of the photoelectric effect. Einstein proposed that light could be thought of as both a wave and a particle, supporting Planck's initial ideas about quantization. He correctly argued that light incidents on a material can eject electrons only if the light's frequency exceeds a certain threshold, demonstrating duality in nature.
This insight validated the concept that energy comes in packets, further solidifying the foundation for quantum mechanics. Its implications ignited a fresh wave of inquiry into light behavior and contributed to the development of technologies such as lasers and solar cells.
In summary, by tracing the historical evolution from Planck’s quantization to Einstein’s interpretation of light, one can appreciate the depth and breadth of quantum physics as a nexus of exploration that has shaped modern scientific thought. The transition into comprehending quantum phenomena isn't just an academic undertaking; it shapes our everyday life, influencing various fields ranging from technology to philosophy and cognitive science.
Fundamental Concepts in Quantum Physics
Diving into the realm of quantum physics, one cannot overlook the significance of its foundational ideas. Understanding these basic concepts is not merely an academic exercise; it allows for a deeper appreciation of phenomena that underpin modern technology and scientific research. From the bizarre behavior of particles at the subatomic level to the intricate relationships established between them, each concept introduces a view into how our universe operates at its most fundamental layer.
Wave-Particle Duality
Concept Origin
The genesis of wave-particle duality traces back to early 20th-century physics. This duality essentially states that particles, such as electrons and photons, exhibit both wave-like and particle-like properties. A key moment in this narrative came with the work of Louis de Broglie, who posited that not only do waves exhibit behaviors associated with particles, but particles can also behave like waves. His proposition expanded the horizons of classical physics and opened up new avenues for inquiry.
One primary characteristic of this concept is the inherent flexibility of particles' identities. They can list in one moment as particles—a pinpoint of mass in space—and then, in another instant, blur into waves. This dual nature presents a profound implication on our grasp of reality, urging us to adjust our intuitive understanding of physical phenomena.
This concept is a beneficial choice for the article because it serves as a gateway to exploring more complex notions of quantum mechanics. However, its unique nature—simultaneously confusing and enlightening—can be tricky for those not steeped in the nuances of quantum theory.
Experimental Evidence
To substantiate wave-particle duality, rigorous experimental evidence has emerged over decades, with notable examples like the double-slit experiment, which highlighted how photons behave differently when observed. When not observed, they act as waves, creating interference patterns on a screen. When measured, they behave like particles, hitting the screen one at a time. This pivotal experiment elucidates not only wave-particle duality but also the overarching theme of observation in quantum mechanics.
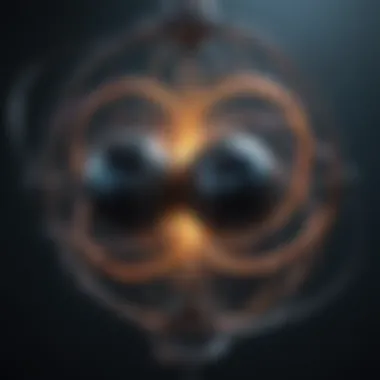
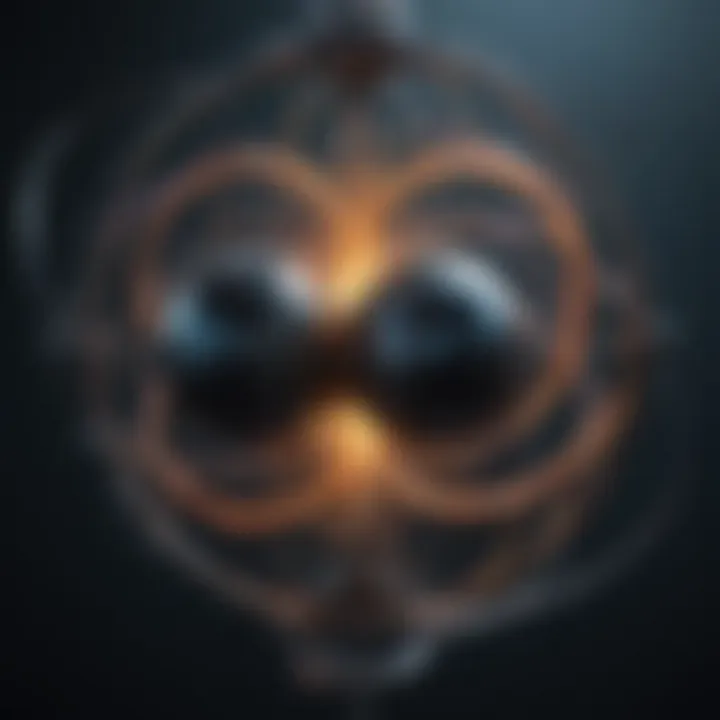
One could argue that the most striking aspect of this evidence is its ability to challenge everyday notions of determinism and reality. This concept's popularity within quantum physics makes it a key focal point for discussions about the nature of existence. Its uniqueness lies in how it compels scholars and students alike to rethink what they know about nature’s building blocks, revealing the intricacies of quantum behavior.
Quantum Superposition
Understanding State Vector
At the heart of quantum mechanics lies the principle of superposition—the idea that particles can exist in multiple states at once until a measurement is made. In a mathematical sense, this is represented by a state vector, encapsulating all potential states of a quantum system. The concept originated from the early investigations by physicists like Schrödinger, who famously described this phenomenon through thought experiments, such as the paradox of Schrödinger's cat.
A standout feature of superposition is its power to encapsulate the probabilities of all conceivable outcomes. This characteristic establishes it as a fundamental element in quantum mechanics, providing vital insight into the behavior of quantum systems. The ability to represent various states with one vector sets the groundwork for understanding complex interactions in multi-particle systems. The complexity of this concept can present a steep learning curve but ultimately enriches our grasp of quantum theory.
Practical Applications
The implications of superposition stretch far into the landscape of applied physics, particularly in quantum computing, where qubits leverage this very principle. Unlike classical bits that exist in states of either 0 or 1, qubits can simultaneously inhabit both states, drastically enhancing computational capabilities.
This is a popular aspect within this article as it bridges theoretical concepts with tangible applications in technology. The distinct feature of superposition in computing reveals how quantum mechanics can revolutionize industries, providing speed and efficiency previously thought unattainable. Its potential, however, faces practical hurdles, such as maintaining coherence in qubits over time, which remains a focal challenge in the field.
Quantum Entanglement
Definition and Examples
Quantum entanglement refers to a phenomenon where two or more particles become interlinked, such that the state of one particle instantaneously influences the state of another, regardless of distance. This concept shattered classical intuitions about separateness and locality, posing questions about how information can be shared instantaneously across vast distances.
The most famous illustration of entanglement involves pairs of particles generated in such a way that their properties are interconnected. For instance, if one particle is measured and found to spin in a particular orientation, the other particle's spin is immediately determined, regardless of how far apart they are. This instant connection serves as an important topic within quantum discourse and highlights the counterintuitive nature of quantum particles.
Phase Information and Non-locality
Diving deeper, the implications of entanglement touch upon phase information and non-locality, two concepts central to understanding interactions among entangled particles. Phase information relates to the position and momentum of particles, influencing outcomes in measurements. Non-locality, on the other hand, revolves around the idea that spatial separation does not prohibit influence between entangled particles.
The key characteristic of non-locality is how it challenges classical views of separateness, suggesting a deeper connection at work in the quantum realm. Quantum entanglement has practical significance, such as in quantum communication and cryptography, although the non-intuitive nature complicates its adoption as researchers endeavor to unravel its full potential.
"Particles are not consistently found; their existence is determined by interaction. This paradigm shift from the known to the unknown is what quantum physics is all about.”
In summary, the fundamental concepts in quantum physics serve as the backbone for understanding a universe that often operates contrary to our intuitive perceptions. Delving into wave-particle duality, superposition, and entanglement not only enriches academic discourse but also opens the door to technological marvels that intertwine with daily life.
Mathematical Framework of Quantum Mechanics
The mathematical framework underlying quantum mechanics serves as the backbone for the intricate theories and applications discussed throughout this article. It's essential, providing clarity and structure to the remarkable concepts that define quantum physics. This framework not only enables physicists to formulate predictions about the behavior of quantum systems but also lays the groundwork for numerous technological advancements.
Understanding the mathematics of quantum mechanics demystifies how particles interact at the quantum level. This level of comprehension is vital for researchers and enthusiasts alike. The prominence of mathematical descriptions in quantum theories allows for precise language and offers a robust platform for articulating complex phenomena.
Quantum States and Operators
In quantum mechanics, quantum states represent the information applicable to a quantum system. They can be visualized as vectors in a complex space. Operators, on the other hand, are mathematical entities that correlate to observable physical quantities, like position or momentum. The interplay between quantum states and operators is crucial, as it allows one to derive measurable predictions from the abstract constructs of quantum theory.
When studying quantum mechanics, grasping these elements is similar to learning a new language. It takes time and application, but once learned, it fosters deeper insight into the realm of quantum systems and aids in making sense of phenomena like superposition and entanglement. An understanding of how states evolve under different operators is fundamental for comprehending how systems behave during various processes.
The Schrödinger Equation
The Schrödinger equation stands as one of the most pivotal equations in quantum mechanics. It mathematically describes how the quantum state of a physical system changes over time, providing a direct linkage between mathematical formalism and observable physics.
Derivation and Interpretation
This part of the Schrödinger equation concerns itself with how the equation is constructed and the physical meanings assigned to its elements. Its derivation stems from fundamental principles of physics, embodying assumptions about wave functions and energy conservation. A key characteristic is its ability to transform complex problems into more manageable expressions. This characteristic makes the Schrödinger equation particularly appealing as it captures the essence of quantum behavior in a tractable mathematical form.
One unique feature of this derivation is the role of the wave function. Reflecting upon how it encodes all possible information about a quantum system makes it clear just how beneficial it is in predicting outcomes. Yet, this comes with its own set of challenges, as the interpretation of the wave function can lead to different philosophical stances, akin to chasing shadows in a fog.

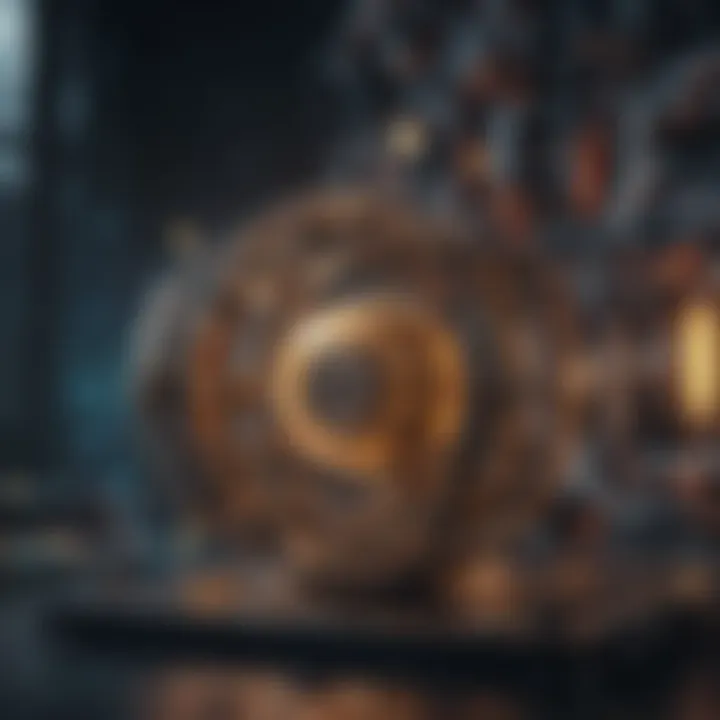
Applications in Quantum Systems
The practical aspects of the Schrödinger equation extend far beyond the theoretical realm; they play a vital role in various quantum systems. This section elucidates how the equation helps model a multitude of phenomena—ranging from semiconductor physics to the behavior of atoms in a laser.
The key characteristic here is applicability, as the equation forms the groundwork for understanding and predicting outcomes in diverse scenarios. What makes the Schrödinger equation so compelling is its inherent versatility, allowing researchers to tailor the equation to fit specific systems and obtain valuable insights. This multidimensional capability represents a significant advantage in both theoretical and experimental contexts.
Probability and Quantum Measurement
In quantum mechanics, the significance of probability cannot be overstated. As systems may only be predicted within a range of likelihoods rather than certainties, the fabric of quantum reality is woven with threads of chance. This leads to the compelling interaction between the crafts of probability theory and quantum measurement.
The Role of the Observer
A contentious yet fascinating subject in quantum mechanics lies in the observer's role. Essentially, it addresses how measurement affects the state of a quantum system. The essence of this concept can be likened to the philosophical debate of whether a tree falling in a forest makes a sound if no one is there to hear it. When an observer measures a quantum state, the act itself can alter the system, exemplifying a peculiar facet of quantum communication.
The key characteristic of this discussion is the idea that observation influences reality. This notion can be beneficial, as it generates new frameworks for understanding how to interpret outcomes based on observational context. Yet, it also poses certain disadvantages, as it blurs the boundary between objective reality and subjective experience, leading to ongoing debates among physicists and philosophers.
The Measurement Problem
The measurement problem evolves from this interplay between probability and observation, questioning how and when a quantum system transitions from a state of superposition to a definitive outcome. This problem encapsulates a central challenge in quantum theory: to understand it thoroughly while reconciling various interpretations.
The measurement problem's key characteristic is its ability to generate discussions that challenge prevailing notions of reality, opening doors to innovative perspectives within quantum physics. The unique feature of this problem rests in its ambiguity, which allows for a range of interpretations—be it the Copenhagen interpretation, many-worlds interpretation, or others. This peculiarity, while encouraging diverse theories, can complicate consensus in the scientific community.
In summary, the content above delves into the mathematical framework governing quantum mechanics, establishing a foundation that underpins profound implications for both scientific inquiry and philosophical discourse. By dissecting mathematical constructs and their applications, we pave the way for a richer understanding of the quantum world—illuminating the subtleties that continue to capture the imagination of scholars and enthusiasts alike.
Implications of Quantum Physics
The implications of quantum physics stretch far beyond the realm of theoretical discussions, permeating modern technology and philosophical thought. Understanding these implications is crucial for grasping the transformative effects quantum mechanics has on both our scientific paradigm and everyday life. This section explores breakthroughs in technology from quantum physics and dives deep into the philosophical reflections it incites, framing a clearer picture for educational and professional endeavors.
Technological Advancements
Quantum Computing
Quantum computing stands as a groundbreaking advancement, shifting the very fabric of computation. The unique characteristic of this technology is its ability to perform operations on multiple states simultaneously, thanks to quantum bits or qubits. Unlike classical bits that are either 0 or 1, qubits can exist in a state of superposition, allowing quantum computers to tackle complex problems at unprecedented speeds.
In this article, the exploration of quantum computing is particularly beneficial due to its potential to revolutionize fields such as cryptography, optimization problems, and drug discovery. The sheer processing power of quantum computers could overcome challenges that traditional computers find insurmountable.
However, quantum computing is not without its challenges. One major disadvantage lies in error rates, as qubits are notoriously delicate and can lose their quantum state through a phenomenon known as decoherence. Yet, researchers are vigorously working on error-correction methods to enhance stability and reliability, making quantum computers a thrilling area of study for the future.
Quantum Cryptography
Following closely behind quantum computing is quantum cryptography, which offers a new layer of security that classical cryptographic methods simply cannot match. Its main feature is leveraging the principles of quantum mechanics to develop secure communication channels. The most well-known application is Quantum Key Distribution (QKD), which ensures that any attempt to eavesdrop on the information can be immediately detected due to the nature of quantum states.
This article showcases quantum cryptography not only as a robust choice for privacy and security in digital communications but also as a fertile ground for ongoing research. The potential to create unbreakable codes is appealing in an era where data breaches and cyber threats are rampant.
However, like its computing counterpart, quantum cryptography comes with its own set of challenges. The practical implementation is often hampered by limitations in distance and the requirement for specialized equipment. Despite these hurdles, the field remains promising and a critical area of interest in the evolving technological landscape.
Philosophical Considerations
Interpretations of Quantum Mechanics
The interpretations of quantum mechanics stir intriguing philosophical debates that challenge our perceptions of reality. Different interpretations such as the Copenhagen interpretation, Many-Worlds, and pilot-wave theory offer varying insights into the nature of quantum phenomena. This conversation is pivotal as it invites not only physicists but also philosophers and thinkers to ponder profound questions about existence and the nature of knowledge.
A key characteristic of these interpretations is how they address the measurement problem—the question of how and when a quantum system transitions from a state of superposition to a single outcome. Some interpretations lean towards a deterministic view while others embrace the randomness inherent in quantum mechanics.
This article highlights the interpretative frameworks as beneficial lenses through which to examine the ontological implications of quantum mechanics. Understanding these perspectives enriches the discourse on consciousness, reality, and the very fabric of the universe. On the flip side, the diverse interpretations can lead to confusion and an overwhelming sense of ambiguity about scientific findings and truths.
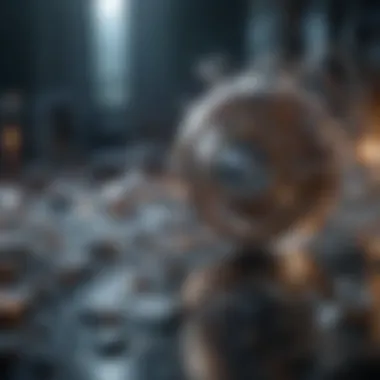
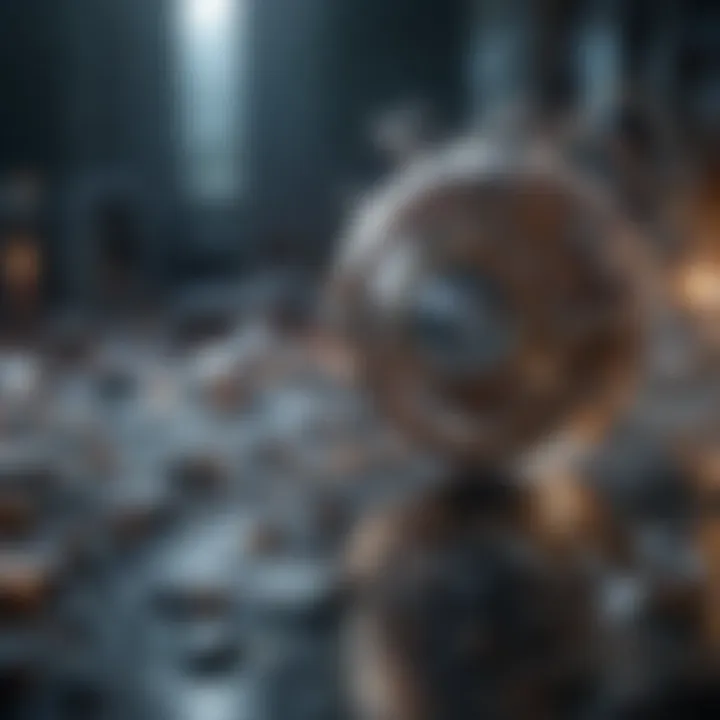
Determinism vs. Indeterminism
The debate between determinism and indeterminism is now more pertinent than ever in the context of quantum mechanics. Determinism suggests that all events are predetermined given prior states, while indeterminism acknowledges the intrinsic randomness that quantum mechanics introduces into our understanding of the universe. This discussion remains a cornerstone in philosophical circles and scientific discourse.
The attraction of this topic in this article lies in its expansive implication for science and morality. If the universe is indeed ruled by indeterminate events, this raises questions about free will and personal agency. Conversely, if determinism prevails, it leads to discussions about predestination and the predictability of behaviors and events.
Current Trends and Future Directions
In the ever-evolving realm of quantum physics, staying abreast of current trends is not just a fleeting desire but a necessity. As groundbreaking discoveries continue to emerge, the implications of these findings extend far beyond theoretical discussions; they hold the keys to unlocking technology's potential. Understanding current trends allows researchers and practitioners alike to navigate the bramble of ideas, tools, and applications that are shaping our scientific landscape. The trends under scrutiny in this section include exciting new frontiers like quantum biology and quantum networks, as well as the persistent challenges in error correction and scalability of quantum systems. These elements not only exemplify the advancement of quantum physics but also underscore its relevance in addressing real-world problems that require innovative solutions.
Emerging Research Areas
Quantum Biology
Quantum biology merges principles of quantum mechanics with biological phenomena, a field that's gaining traction rapidly. One of the most captivating contributions of quantum biology lies in its examination of processes like photosynthesis, where molecules exhibit quantum effects to achieve remarkable efficiencies. Research indicates that plants manage to transport energy through quantum coherence, a remarkable feat that suggests the universe might have finely tuned mechanisms at play within biological systems.
One of the key characteristics of quantum biology is its interdisciplinary nature—it draws on physics, biology, and chemistry, making it a melting pot of scientific inquiry. This appeal makes it a popular topic in this article, as many scholars see it as a way to explore fundamental questions about life itself. The unique feature of quantum biology is its focus on how quantum phenomena underpin complex biological processes. While exciting, the field faces its share of challenges, particularly when it comes to replicating these quantum effects in laboratory conditions. The advantages are clear, with the potential for renewable energy solutions and improved medical therapies; however, questions about feasibility and reproducibility remain.
Quantum Networks
Quantum networks represent another cutting-edge research area, a system that holds the promise of revolutionizing communication. These networks utilize the principles of quantum entanglement and superposition to establish secure communication channels that classical networks simply cannot match. The key characteristic here is their ability to leverage quantum bits or qubits that can exist in multiple states simultaneously, allowing for unparalleled data transmission capabilities.
This intriguing characteristic makes quantum networks a compelling choice for this article—security in data transmission is a significant concern in our digital age and quantum networks promise to alleviate many threats posed by classical encryption methods. Their unique feature lies in immediate, unbreakable security guaranteed by the laws of quantum physics itself. However, building usable quantum networks involves a steep learning curve and a range of technical obstacles. While the long-term benefit of secure communication is tremendous, current technology presents limitations such as distance and error rates that need addressing.
Challenges in Quantum Technologies
Error Correction in Quantum Computing
As quantum computing propels forward, one particular challenge stands out: error correction. Unlike classical computing, where bits are either zero or one, qubits can be in superpositions, making them susceptible to a host of errors caused by environmental noise. Error correction in quantum computing is a sophisticated system designed to detect and correct errors without disturbing the fragile quantum states. This aspect of quantum technology is one of the backbone necessities for ensuring reliable computation.
The complexity of error correction methods means they are crucial yet daunting, and they feature prominently in the ongoing development of robust quantum systems. Their unique feature is the need for redundancy—more qubits must be allocated to maintain a single logical qubit. While this may slow computations temporarily, the long-term benefits include the trustworthiness of results. So, while error correction is a tough nut to crack, its eventual success could open the floodgates for mass adoption of quantum computing.
Scalability of Quantum Systems
The scalability of quantum systems brings its own set of hurdles. Researchers are striving to build larger and more powerful quantum systems, moving from small prototypes to practical models that can handle real-world computations. One of the key characteristics of this challenge is the engineering of qubits themselves, which must be manipulated in ways that will sustain their delicate superposition states over time.
The unique feature here is the balance between increasing qubit numbers and maintaining coherence and minimal error rates. As exciting as scalable quantum systems are, they represent a painstaking journey filled with trade-offs. The advantages of scalability include enhanced computational power and broader applicability of quantum technologies across industries. However, it's a double-edged sword with the potential for increased complexity, costs, and the fundamental physics of maintaining qubit states intact.
The landscape of quantum physics is a tempest of challenges and innovations. Gaining clarity around these current trends is essential for any serious inquiry into this revolutionary field.
Epilogue
In the realm of science, the conclusion we draw from our exploration of quantum physics holds significant weight. This field, often viewed through a lens of enigma, actually reveals fundamental truths about the universe. The insights we garnered throughout this article provide a framework not just for understanding, but for appreciating the meticulous dance between theoretical constructs and practical applications.
Reflecting on Quantum Physics
The Importance of Quantum Mechanics
Quantum mechanics serves as the bedrock of modern physics, influencing diverse scientific domains from chemistry to material science. Its key characteristic is its ability to describe the behavior of matter and energy at astonishingly small scales. Unlike classical mechanics, quantum mechanics introduces concepts like wave-particle duality and uncertainty, reshaping how we understand existence itself. This is beneficial for our discourse as it underscores a shift from a deterministic viewpoint to one where probabilities reign supreme.
The unique feature of quantum mechanics lies in its probabilistic nature; instead of providing certainties, it offers a range of possibilities. This can be seen in practical applications like quantum computing, where information processing isn't just faster, but fundamentally different. However, this uniqueness presents challenges, especially in terms of interpretation and application in technology. For example, quantum states can be entangled in ways that defy classical explanations, yet harnessing this for everyday use in computing and encryption remains a puzzle.
Future Perspectives in Science
Anticipating future perspectives in science, particularly in quantum physics, we look towards the unfolding potential of quantum technologies. This aspect is vital since it may redefine our approach to computation, communication, and even understanding biological processes on a quantum level. A key characteristic here is the innovation driven by quantum research, making it a focal point for both academia and industry.
What sets the future of quantum science apart is its unique blend of theoretical exploration and cutting-edge technological advancements. Initiatives like quantum networks and quantum sensors are but the tip of the iceberg. The implications of these technologies are profound, promising enhancements in security and speed that are currently unfathomable. Yet, the intricacies of implementing reliable quantum systems present a challenge that calls for further research and collaboration across disciplines. This duality of promise and difficulty highlights both the opportunities that lie ahead and the hurdles that must be overcome as the field progresses.
Quantum mechanics reshapes our perception of reality, establishing a framework where certainty yields to potentiality.
Thus, as we reflect on quantum physics, we recognize its pivotal role not just in scientific discourse, but in the way we interact with and comprehend our universe. By engaging with these ideas, we prepare ourselves for futures that are as much constructed by our understanding of quantum phenomena as they are by our combined efforts to innovate within this captivating field.