Understanding Quantum Information Theory and Its Impacts
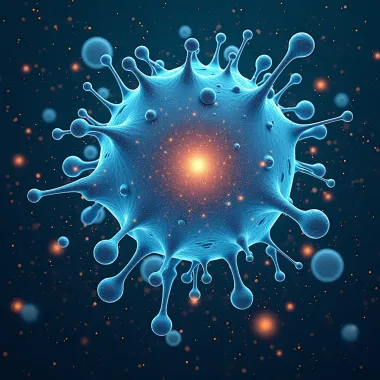
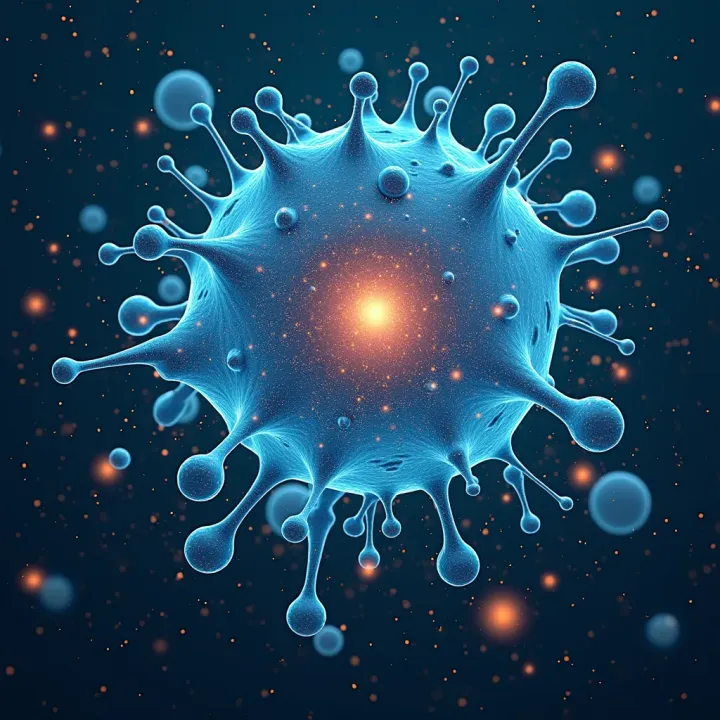
Intro
Quantum information is a field that combines the principles of quantum mechanics and information theory. It offers a distinct approach to understanding how information can be represented, processed, and transmitted using quantum states. As technology progresses, the significance of quantum information has grown, influencing various fields, such as cryptography, communication, and computing.
With the advent of quantum computing, traditional understandings of information processing are being challenged and redefined. Thus, grasping the fundamentals of quantum information is crucial for students, researchers, educators, and professionals eager to navigate this evolving landscape.
Key Research Findings
Overview of Recent Discoveries
Recent research in quantum information has led to several key discoveries. One of the most notable advances is the concept of quantum entanglement, where particles become linked, allowing for instantaneous communication regardless of distance. This property raises important questions about the nature of reality and the limits of classical information transfer.
Another significant finding is the development of quantum algorithms, such as Shor's algorithm for factoring large integers. This algorithm demonstrates that quantum computers could outperform classical computers in specific tasks, thereby transforming fields like cryptography.
Some additional discoveries include the exploration of quantum teleportation and quantum key distribution, both of which have profound implications for secure communications.
Significance of Findings in the Field
The findings in quantum information research hold transformative potential. They challenge the established paradigms of both quantum mechanics and information theory, suggesting new applications and systems that could enhance data security and computing speed. The implications for cryptography are especially profound, as these discoveries may render currently used encryption methods obsolete.
"The development of quantum information theory opens pathways to enhance security in communication systems, setting a new standard for data protection."
Supporting Concepts and Theories
Understanding these findings requires familiarity with certain concepts in quantum mechanics and information theory. Here are some fundamental ideas:
- Quantum Superposition: This principle states that a quantum system can exist in multiple states at once until observed.
- Quantum Measurement: Measurement in the quantum realm collapses a superposition into a single state, altering the information contained within.
- No-Cloning Theorem: This theorem asserts that it is impossible to create an identical copy of an arbitrary unknown quantum state, which influences security in quantum communications.
By unpacking these concepts, one can appreciate the innovative nature of quantum information and the depth of research being conducted in the area.
Breakdown of Complex Concepts
Simplification of Advanced Theories
The advanced theories behind quantum information can be quite intricate. Simplifying these concepts is essential for broader understanding. Here are some techniques effective in facilitating this:
- Use of Analogies: Applying relatable comparisons to everyday scenarios can help digest challenging ideas.
- Incremental Learning: Mastering basic principles first then gradually tackling more complex theories aids comprehension.
Visual Aids and Infographics
Visual aids are also valuable tools for breaking down complex ideas. One effective method is through infographics that visualize the relationships and functions of quantum states.
For example, an infographic illustrating quantum entanglement could showcase how two particles interact despite distance, making the abstract concept more tangible.
Prolusion to Quantum Information Theory
The intersection of quantum mechanics and information theory has become a focal point of scientific exploration. Understanding quantum information theory is crucial, particularly as we delve into the nuances of how information operates within quantum systems. This field not only challenges our classic views of information but also provides innovative solutions and technologies that could redefine computing, cryptography, and communication.
Defining Quantum Information
Quantum information can be viewed as the information that is held within the state of a quantum system. Unlike classical information, which encompasses bits that can be either 0 or 1, quantum information utilizes quantum bits, or qubits. These qubits can exist in multiple states simultaneously due to the principle of superposition. This capability drastically increases the computational power and efficiency of quantum systems.
Defining quantum information leads to critical insights into how data is processed and transmitted at a fundamental level. The implications resonate broadly across different fields such as computer science, physics, and engineering. This evolving narrative compels researchers to reconsider traditional concepts and adapt to new paradigms in information processing and storage.
Historical Context and Development
The evolution of quantum information theory has a rich historical context. It originated from thought experiments and theoretical contemplation in the early 20th century. Pioneers like Niels Bohr and Albert Einstein laid the groundwork by grappling with the quirks of quantum mechanics. However, it was not until the 1980s that significant breakthroughs began to emerge. Researchers like Richard Feynman proposed that quantum systems could simulate physical processes more efficiently than classical computers.
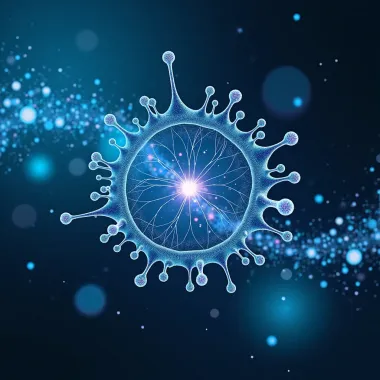
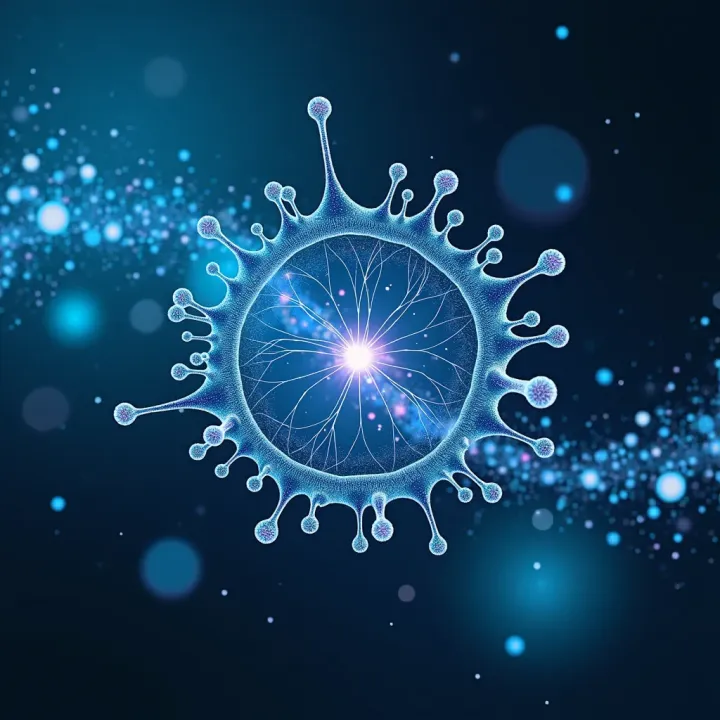
In the subsequent years, figures such as David Deutsch and Peter Shor advanced the field further. Deutsch introduced the concept of a universal quantum computer, while Shor developed an algorithm that could factor large numbers exponentially faster than classical methods. This discovery ignited discussions among experts about the potential and risks associated with quantum computing, particularly in terms of security and cryptography.
Today, quantum information theory continues to develop. The relativity of information in quantum mechanics has prompted questions about locality, causality, and the nature of reality itself. This journey illustrates the dynamic relationship between theoretical investigations and practical applications, establishing a framework that guides current research and technological innovations.
"Quantum information theory is reshaping our understanding of what information is and how it can be manipulated."
In summary, the importance of understanding quantum information theory cannot be overstated. It not only informs current research but also impacts future innovations across various domains.
Fundamental Concepts of Quantum Mechanics
Fundamental concepts of quantum mechanics serve as the backbone for understanding quantum information. This area of physics introduces principles that defy classical mechanics, expanding our comprehension of the universe at a microscopic scale. Embracing these concepts is essential not only for scientists but also for those engaged in the fields of computer science, cryptography, and communication.
The interaction between quantum mechanics and information theory unveils a new dimension of how we perceive, process, and transmit data. Without grasping the intricacies of quantum states, superposition, entanglement, and measurement, one cannot fully appreciate the power and potential of quantum information.
Quantum States and Superposition
Quantum states represent the unique condition of a quantum system, encapsulating all possible information about its properties. These states can be indicated in various forms, such as pure states and mixed states, depending on the level of knowledge about the system.
Superposition is a crucial principle where a quantum system can exist in multiple states simultaneously. This idea considerably contrasts with classical binary systems, where a bit is either 0 or 1. In quantum mechanics, a qubit can be both 0 and 1 at the same time, illustrated by the expression:
[|\psi\rangle = \alpha |0\rangle + \beta |1\rangle]
where ( \alpha ) and ( \beta ) are complex numbers satisfying the normalization condition. This property of superposition allows quantum computers to perform many calculations at once, providing a significant advantage over classical computing methods.
Entanglement and Nonlocality
Entanglement is a phenomenon in which two or more quantum particles become interlinked. Once entangled, the state of one particle instantly influences the state of another, regardless of the distance separating them. This concept leads to what Einstein famously termed "spooky action at a distance."
Entangled particles exhibit correlations that classical physics cannot explain. These correlations can be utilized in applications ranging from quantum cryptography to quantum teleportation. Entanglement essentially raises questions about the nature of information and communication, offering new pathways in the exploration of quantum technologies.
Measurement and Quantum Collapse
Measurement in quantum mechanics refers to the process of acquiring information about a quantum system. When a measurement is made, the quantum system transitions from a superposition of states to a single outcome. This process is known as quantum collapse.
The act of measuring is not merely passive; it alters the state of the system. This raises essential considerations concerning information retrieval and uncertainty. Notably, the Heisenberg Uncertainty Principle states that certain pairs of properties, like position and momentum, cannot be precisely known simultaneously.
It is crucial to understand that measurements have intrinsic limitations and implications. This understanding leads to ongoing discussions within the scientific community concerning the foundational aspects of quantum mechanics and its relation to information.
"The study of quantum mechanics and its implications on information is reshaping our understanding of both fields and will undoubtedly alter the technological landscape in the years to come."
In summary, grasping the fundamental concepts of quantum mechanics is vital for delving into quantum information theory. Recognizing the complexities of quantum states, superposition, entanglement, and measurement offers a clearer perspective on how these principles inform advancements in areas such as quantum computing and cryptography.
The Mathematical Framework of Quantum Information
The mathematical framework of quantum information serves as the backbone for understanding how information behaves in quantum systems. This framework provides a systematic approach to describe quantum states, transformations, and the processing of information at its most fundamental level. A solid grasp of this framework is essential for anyone looking to delve into the intricate world of quantum information, as it lays the groundwork for innovations in quantum computing and cryptography.
Qubits and Quantum Gates
Qubits, or quantum bits, represent the basic unit of quantum information. Unlike classical bits, which can exist as either 0 or 1, qubits can exist simultaneously in multiple states due to the principle of superposition. This unique property allows quantum systems to perform many calculations at once, vastly increasing their computational power.
Quantum gates, which are the quantum equivalent of classical logic gates, manipulate qubits to perform operations. These gates can change the state of a qubit or a group of qubits using unitary transformations. For instance, a Hadamard gate creates superposition, while a CNOT (Controlled NOT) gate introduces entanglement between qubits. The arrangement and combination of these gates form quantum circuits, enabling complex computations.
In practice, the ability to manipulate qubits through quantum gates leads to the potential for vast improvements in algorithmic efficiency and speed. This capability is crucial for developing algorithms that can solve problems beyond the reach of classical computers.
Density Matrices and Mixed States
While pure states describe an idealized qubit system, real-world systems often involve mixed states. These are statistical mixtures of pure states, which can occur due to decoherence and interaction with the environment. To handle these situations, density matrices provide a more comprehensive mathematical framework than wave functions alone.
A density matrix encapsulates all possible information about the quantum state of a system, including probabilities of different outcomes during measurements. This allows for the description of entangled systems, where individual measurements on parts of the system do not provide complete information about the whole. Understanding density matrices is essential for researchers who explore complex quantum systems as they account for reality's inherent uncertainties.
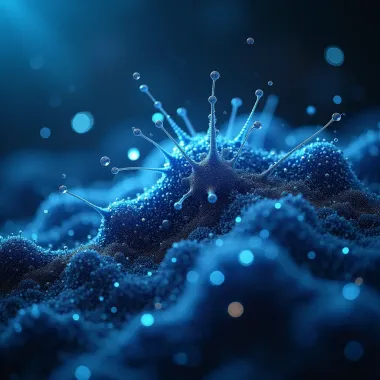
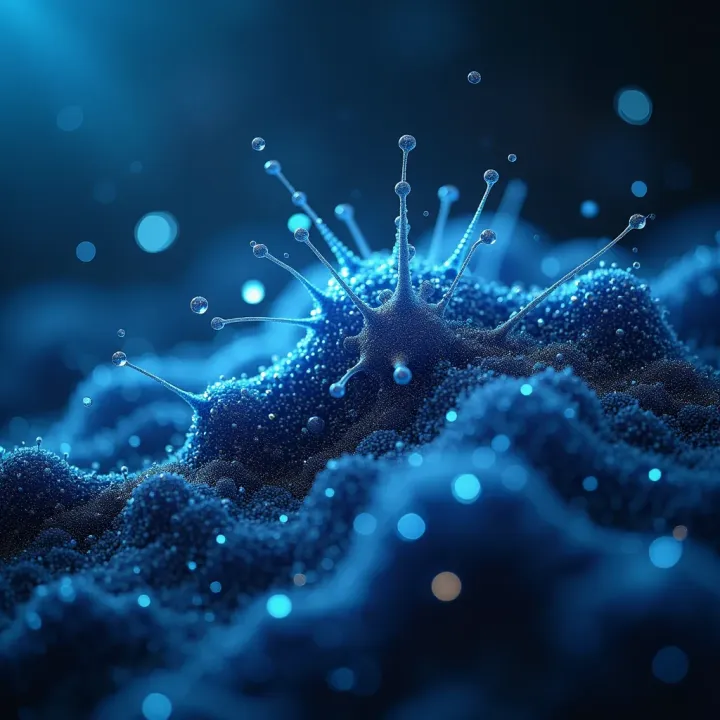
"In quantum information, recognizing the nuances of mixed states is as important as understanding idealized models."
Quantum Algorithms and Computational Complexity
Quantum algorithms leverage the principles of quantum mechanics to solve problems in ways that classical algorithms cannot. For example, Shor's algorithm enables efficient factorization of large integers, which poses a significant challenge for classical computers. In contrast, Grover's algorithm provides a quadratic speedup for unstructured search problems.
The computational complexity of quantum algorithms hinges on the nature of quantum superposition and entanglement. This results in a completely different framework for analyzing algorithm efficiency. Open questions remain in the field regarding the limits of quantum computing capabilities compared to classical models.
As quantum technologies evolve, understanding the mathematical foundations becomes increasingly relevant. The mathematical framework of quantum information is not just theoretical; it is the cornerstone of practical advancements in many scientific fields.
This framework not only enhances our computational abilities but also reshapes our understanding of information itself in the quantum realm. Its importance extends beyond mere calculations, influencing how we conceptualize data transmission, encryption, and computational processes in broader scientific applications.
Applications of Quantum Information Theory
The applications of quantum information theory are numerous and significant, shaping a diverse array of fields and industries. This segment will elucidate the vital role quantum information plays in various sectors, including computing, cryptography, and communication. Understanding these applications highlights not just theoretical advancements but also practical benefits and real-world implications.
Quantum Computing Innovations
Quantum computing represents a groundbreaking leap in computational capabilities. By utilizing quantum bits, or qubits, quantum computers can process information in ways classical computers cannot. This advantage allows for vastly improved problem-solving efficiencies, especially in complex fields such as optimization, material science, and machine learning.
Recent innovations in quantum algorithms, like Shorβs algorithm for factoring large integers and Groverβs algorithm for searching unsorted databases, showcase the potential of quantum computing. These advancements can dramatically reduce execution time for tasks that would take classical computers impractically long periods to solve. Organizations such as IBM and Google are leading in this area, with initiatives like IBM's Quantum Experience, which allows users to run experiments on real quantum processors. This makes innovative quantum computing more accessible to researchers and enthusiasts alike, driving exploration and discovery.
Advancements in Quantum Cryptography
In the realm of security, quantum cryptography lifts the standard of encryption techniques. Traditional encryption methods can be vulnerable to hacking, especially with the advent of more powerful computing technologies. Quantum key distribution (QKD) addresses these weaknesses by utilizing the principles of quantum mechanics. This offers secure communication channels that are provably immune to eavesdropping.
The most notable protocol in this area is BB84, developed by Charles Bennett and Gilles Brassard. It enables two parties to generate a shared, secret random key. Any interception attempt would disturb the quantum states, thus alerting the communicating parties of potential breaches. Numerous institutions and startups, such as ID Quantique and Quantum Xchange, have begun implementing quantum cryptography in various applications, from financial transactions to government communications, ensuring a heightened level of security in an increasingly digital world.
Quantum Communication Protocols
Quantum communication extends beyond cryptography; it encompasses protocols that facilitate the transfer of information using quantum systems. Techniques like quantum teleportation and superdense coding represent innovative strides in information transmission capabilities.
Quantum teleportation allows for the transfer of quantum states between two distant qubits. Although it would not teleport physical matter, it is groundbreaking in moving fragile quantum states without measuring them directly. On the other hand, superdense coding enables the transmission of two classical bits of information by sending only one qubit. These protocols showcase the fundamental shift in how we can perceive and handle information.
"Quantum communication can redefine how data is shared globally, promising new levels of efficiency and security."
Through advances in these areas, researchers are grappling with the potential of quantum repeaters, which could be essential for long-distance quantum communication systems. The global quantum internet becomes a tangible vision as these protocols and technologies evolve.
Theoretical Foundations and Philosophical Implications
The study of quantum information is not only about computations and algorithms; it bridges the gap between physics and information theory. This intersection raises deep theoretical questions and philosophical implications. Understanding these foundations is crucial as they help to define the principles governing how quantum information functions. This section unpacks the theme of quantum information, revealing its importance and exploring its broader impact on our understanding of the universe.
Information as a Physical Entity
In classical physics, information is often viewed as an abstract concept that exists independently of physical systems. Quantum mechanics, however, challenges this notion. Information in quantum systems is tied to the physical state, making it a tangible entity. This perspective leads to significant implications for how we understand measurement, states, and the nature of reality itself. The realization that information can have physical representation, such as qubits, fundamentally alters our approach to various scientific fields.
Why does this matter?
- It redefines the boundaries of thermodynamics and computation.
- It suggests that the laws of physics can be interpreted through the lens of information.
- It encourages new ways to explore the universe, integrating physics and information.
The Role of Information in Quantum Mechanics
Quantum mechanics relies heavily on the manipulation and transfer of information. Information operates in a different concept, contrasting classical bits that assume definite values of 0 and 1. In quantum mechanics, due to superposition, a qubit can represent multiple values at once. This leads to new behaviors and phenomena, such as quantum entanglement, where the state of one qubit can depend instantaneously on the state of another, regardless of distance.
This role has several benefits:
- Enables the development of quantum algorithms which can outperform classical counterparts.
- Facilitates advancements in secure communication through quantum cryptography.
- Provides a deeper understanding of reality at a fundamental level.
Implications for Classical Concepts of Reality
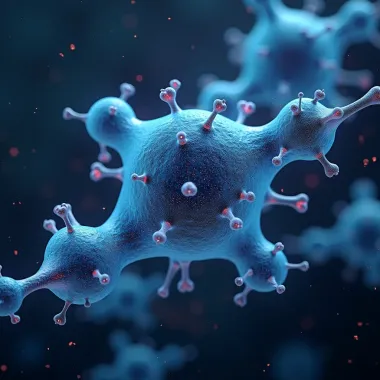
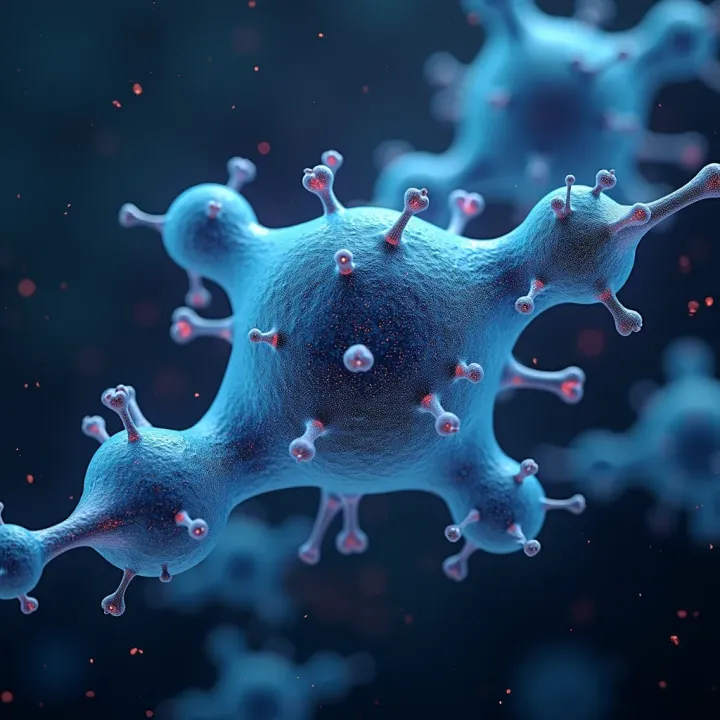
The intersection of quantum information theory and classical reality raises profound questions. Traditional concepts of determinism are challenged by the probabilistic nature of quantum mechanics. Reality, once perceived as a fixed structure, becomes fluid and interconnected. Quantum theory suggests that the observer plays a crucial role in measurement and thus reality is not entirely objective.
βThe universe is not only queerer than we suppose, but queerer than we can suppose.β
βJ.B.S. Haldane
These implications call for a reevaluation of established theories and philosophical inquiries. Concepts of causality, time, and locality may need to be revisited in light of quantum information theory. This blending of philosophy and science can unlock new pathways for research and understanding, emphasizing the need for interdisciplinary dialogue.
Challenges and Future Directions
The landscape of quantum information theory is vast and intricate, populated with both exciting potential and notable challenges. As researchers and practitioners seek to enhance our understanding of quantum systems, this section will outline the specific hurdles present in the field, advancements we hope to achieve, and the ethical considerations that underpin our inquiries. Addressing these aspects is crucial for both practical applications and theoretical exploration, as they shape the future of technology and our comprehension of quantum nature.
Technical Limitations in Quantum Technologies
One of the primary barriers in the advancement of quantum technologies is related to the physical implementations of quantum systems. Current quantum computers, such as those developed by IBM and Google, often grapple with issues of coherence and fault tolerance.
When qubits interact with their environment, they can lose the delicate quantum states that are necessary for computation. This phenomenon is known as decoherence, and minimizing its impact is essential for effective quantum computing.
Moreover, the fabrication of reliable quantum bits remains difficult.
- Qubits can be susceptible to error induced by environmental noise.
- Error-correcting codes need to be developed to help mitigate such flaws in computation.
Further, scaling quantum systems poses significant engineering challenges. As systems grow larger, maintaining control and ensuring performance require sophisticated approaches. Advances in error correction and qubit connectivity are vital steps towards realizing more robust quantum technologies.
The Quest for Quantum Standards and Protocols
Developing universal quantum standards and protocols is indispensable for establishing consistent frameworks within the field. Unlike classical systems that often have established benchmarks, quantum information systems still need guidelines to ensure interoperability and security. This lack of standards can hinder progress and application in various industries.
Creating protocols for quantum communication is an immediate necessity, particularly in areas such as quantum cryptography. For instance, methods like Quantum Key Distribution, which allows two parties to share cryptographic keys securely, demand rigorous standards to ensure they are both practical and secure.
A few key points include:
- The establishment of uniform quantum protocols will facilitate better communication between different quantum systems.
- Research must continue to enhance security measures against potential vulnerabilities in the quantum realm.
Additionally, intergovernmental cooperation will be crucial in setting these standards, allowing nations to come together for collective benefits in quantum advancements while also establishing trust frameworks across borders.
Ethical Considerations in Quantum Research
The advance of quantum technologies brings not only technical challenges but also ethical dilemmas. As we harness power from quantum information, implications about its misuse or potential for harm must be addressed. Consideration of these aspects is essential for responsible exploration of the technology.
- The use of quantum computers in breaking current encryption methods could have ramifications across sectors, from finance to personal privacy. This calls for a dialogue on regulations and limitations surrounding quantum computing.
- Researchers must consider how advancements can affect employment landscapes, given the disruptive nature of technologies such as quantum computing and artificial intelligence.
"As we proceed into the quantum world, it is imperative that we keep ethical implications in context, fueling discussions that foster responsible advancements."
In summary, addressing challenges in quantum information theory, ranging from technical limitations to ethical concerns, will greatly influence our future directions in the field. Each of these factors intertwines, shaping the trajectory of quantum technology as we strive for practical, advanced applications.
End and Summary
In concluding this exploration of quantum information theory, it is essential to appreciate its significance in both theoretical and practical dimensions. The field is not merely an academic pursuit; it possesses far-reaching implications that could transform multiple industries. Quantum information theory bridges the gap between physics and information science, thus unlocking new capabilities in computing, cryptography, and communication.
Recapitulating Key Concepts
As we recapitulate the key concepts, we first acknowledge the foundational principles. Quantum states and the superposition principle mark a significant departure from classical information theory. Superposition allows quantum bits, or qubits, to exist in multiple states simultaneously. Coupled with the phenomenon of entanglement, the interplay between quantum systems enables protocols such as quantum key distribution, which offers unprecedented security in communication.
Additionally, the mathematical framework, involving density matrices, provides a robust approach to managing quantum uncertainty. This framework supports advanced quantum algorithms that enhance computational processing, illustrating the potency of quantum information theory over classical paradigms.
"Quantum information is transforming our approach to secure communication, enabling levels of privacy previously considered unattainable."
In summary, the key concepts we discussed serve as building blocks for understanding the larger picture of quantum information theory and its applications.
The Continual Evolution of Quantum Information Theory
The field of quantum information theory is far from static; it is in a continual state of evolution. New discoveries emerge, often challenging existing paradigms. Core practices in quantum computing and cryptography are shifting rapidly as technology advances. Researchers are exploring innovative solutions to existing hurdles, such as technical limitations and the need for universally accepted standards and protocols.
Moreover, ethical discussions are becoming increasingly pertinent. As we push the boundaries of technology, we face critical considerations about its implications for privacy and security. Each evolution brings new responsibilities and questions about the impact on society.
Through diligent research and collaboration, the potential for quantum information theory continues to unfold, revealing insights that promise significant advancements, both conceptual and technical.