Understanding Quantum Computing: A Deep Dive
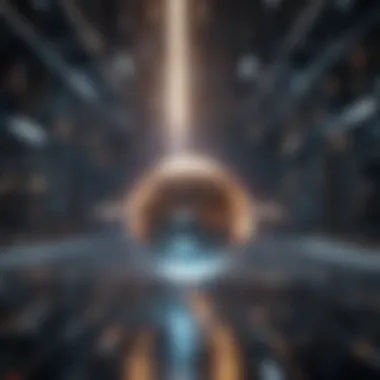
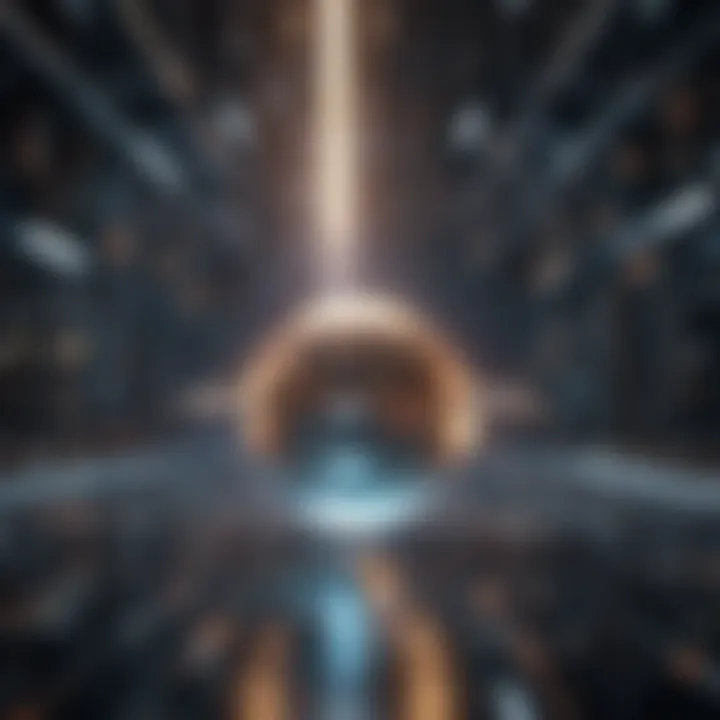
Key Research Findings
Quantum computing, an innovative domain of computer science, is propelling us toward unprecedented challenges and solutions in computation. Recent years have seen an accelerated pace of research and practical application of quantum technologies.
Overview of Recent Discoveries
In the last few years, several key advancements have emerged in quantum computing. Breakthroughs include the development of superconducting qubits, which are critical for creating scalable quantum circuits. Googleβs 2019 experiment achieving quantum supremacy demonstrated the ability of quantum processors to solve specific problems faster than classical computers. Researchers at IBM have also made progress in developing quantum volume, a measure of the capabilities of their quantum processors. Studies are revealing new algorithms that harness the potential of quantum speedup for problem-solving in logistics and cryptography.
Significantly, the exploration into quantum error correction codes is advancing. These codes are essential as quantum systems are notably prone to errors due to decoherence. Therefore, the refinement of error correction strategies is vital for reliable quantum computing.
Significance of Findings in the Field
The implications of these findings are profound. By leveraging the principles of quantum mechanics, these technologies promise to revolutionize industries, enhance data security, and enable complex simulations that were hitherto impossible. Industries such as pharmaceuticals are already using quantum simulations to develop new drugs more efficiently. Additionally, improvements in the computational power of quantum systems can dramatically change processes such as optimization, which is critical in logistics and resource management.
"Quantum computing offers a new paradigm that redefines the limits of computability and efficiency, with potential implications across diverse sectors."
Breakdown of Complex Concepts
Quantum computing employs principles that may seem alien to those accustomed to classical computation. To demystify this domain, we can break down some of its essential elements.
Simplification of Advanced Theories
At the core of quantum computing lie the concepts of qubits and superposition. Unlike classical bits, which can represent a 0 or a 1, qubits can exist in a state of superposition. This allows quantum computers to process vast amounts of information more efficiently. Another critical principle is entanglement, where qubits become interdependent, such that the state of one qubit can instantly influence the state of another, regardless of the distance separating them.
Visual Aids and Infographics
Illustrating these concepts assists in their comprehension. Diagrams can showcase quantum circuits, the behavior of qubits, and the distinctions between classical and quantum algorithms. Such visual tools aid in visualizing complex interactions and enhance understanding of their practical applications.
To facilitate this exploration, additional resources such as Wikipedia provide further insights into these complex topics, while platforms like Reddit host discussions and insights from the quantum computing community.
Prologue to Quantum Computing
Quantum computing stands as a pivotal development in the domain of information technology. Its importance cannot be overstated, as it promises to transform computation in ways that classical computers cannot achieve. From cryptography to materials science, the implications of quantum computing extend into numerous fields, asserting its relevance in both theoretical and practical applications.
The concept of quantum computing intertwines deeply with the principles of quantum mechanics, which govern the behavior of matter at an atomic level. This intersection creates a rich terrain for exploration, making it a critical topic for students, educators, and professionals alike. Understanding this arena can illuminate many complexities about computational capacity and efficiency, particularly in dealing with problems that were previously deemed infeasible.
One of the primary benefits of embracing quantum computing is its potential to solve complex problems at unprecedented speeds. Tasks such as factoring large numbers, simulating quantum systems, or optimizing intricate processes are areas where quantum algorithms may excel. As we traverse through this comprehensive exploration, it is essential to grasp these intricate concepts not just as theoretical musings but as pathways toward real-world advancements.
Understanding quantum computing is not merely an academic pursuit; it reflects on future technological evolution. By dissecting its core components, we can appreciate the opportunities and challenges it presents, enabling informed discussions about its broader impacts on society. This article aims to bridge the gap between advanced scientific concepts and a broad audience, offering insights and encouraging critical thought regarding the transformative power of quantum computing.
Defining Quantum Computing
Quantum computing refers to the use of quantum-mechanical phenomena, such as superposition and entanglement, to perform computational tasks. Unlike classical computers, which use bits as the smallest unit of information, quantum computers utilize quantum bits, or qubits. A qubit can represent and store data in states of 0, 1, or both simultaneously, allowing for parallel processing of information. This unique characteristic underscores how quantum computing can surpass classical methods by exploring multiple possibilities at once, thereby reducing computation time dramatically in suitable applications.
In essence, the quantum computer operates on principles that defy our classical understanding of physics. While a standard computer performs calculations in a linear fashion, a quantum computer can process vast amounts of data far more rapidly, making it suitable for tasks that rely on the manipulation of complex information.
Importance in Modern Science
The emergence of quantum computing holds significant importance within the landscape of modern science. It provides a framework for addressing questions that classical computers struggle with. For example, in fields like cryptography, quantum computers can achieve levels of security impossible with traditional systems, utilizing algorithms specifically designed to exploit their unique capabilities.
Furthermore, quantum computing facilitates breakthroughs in drug discovery and materials science by allowing the simulation of molecular interactions at a granular level. This precision could lead to the development of new materials and medications tailored to specific needs.
Moreover, the implications of quantum technology extend into artificial intelligence, optimization problems, and beyond. As quantum computing evolves, it will likely redefine efficiency and capabilities across diverse sectors, marking a paradigm shift in how we approach problem-solving and innovation.
"Quantum computing isn't merely a new way of computing; it is set to challenge our very understanding of computation itself."
In summary, the foundational understanding of quantum computing serves as a vital piece in grasping the potential and challenges of future technology. By engaging with these concepts, one can appreciate their transformative impacts on modern science and technology.
Historical Perspectives
The exploration of quantum computing represents a paradigm shift in how we understand processing and computation. Historical perspectives shed light on the evolution of this field. Understanding the journey that led to the current state of quantum computing allows us to appreciate the complexity and potential that it holds for the future. It creates a context for the advancements made in technology and theory, illustrating how past research laid the groundwork for what is now a rapidly developing discipline.
Early Theoretical Foundations
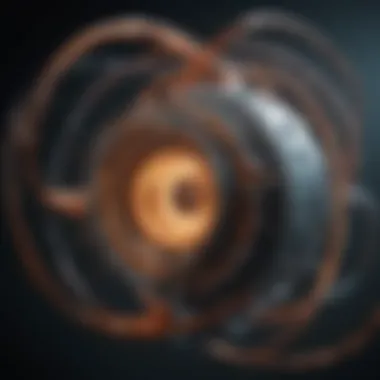
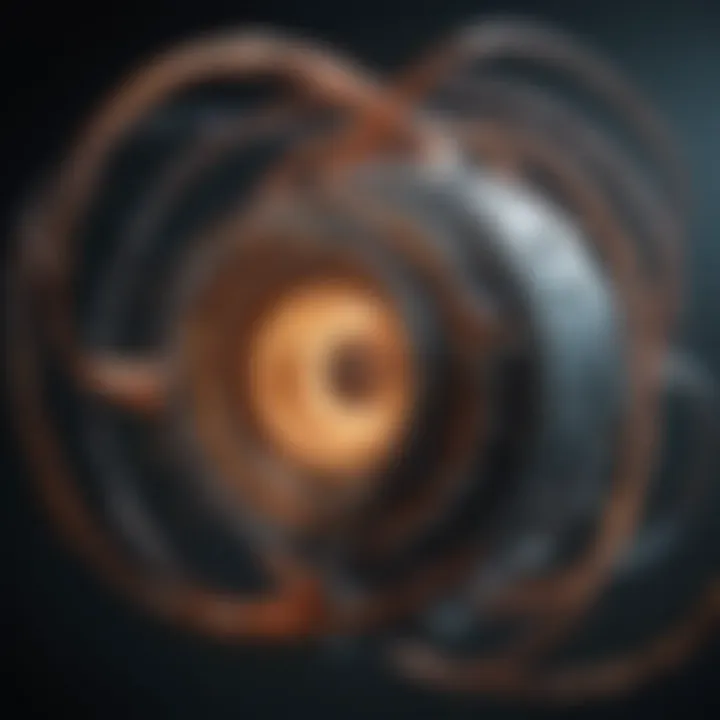
The origins of quantum computing can be traced back to the early 20th century when physicists began to explore the peculiar behavior of subatomic particles. Quantum mechanics emerged as a way to describe phenomena that classical physics could not account for. Figures like Max Planck and Albert Einstein were integral during this period, introducing concepts such as quantization of energy.
For instance, Planck's quantization theory, initially proposed to resolve black-body radiation issues, was a significant stepping stone. Further work by Niels Bohr on atomic models also contributed to this nascent field.
By the late 1980s, the idea of using quantum systems for computation started to gain traction. The work by David Deutsch in 1985 introduced the concept of a universal quantum computer. It proposed a model that applies quantum mechanics to computational processes, suggesting the capability of performing tasks impossible for classical computers.
This theoretical groundwork not only informed subsequent research but also inspired a generation of computer scientists and physicists to make further inquiries into the capabilities of quantum systems. The interplay of physics and computer science became increasingly important as researchers identified that quantum phenomena could indeed be harnessed for computational purposes.
Key Milestones in Development
The path from theory to practice in quantum computing has been marked by several key milestones which helped propel the field forward. In 1994, Peter Shor developed an algorithm that could factor large numbers exponentially faster than the best-known classical algorithms. This was not only groundbreaking for cryptography but also validated the concept that quantum computers could solve problems deemed intractable for classical systems.
In the following years, there were additional important advancements. Lov Grover's algorithm, presented in 1996, promised a significant speedup for unstructured search problems, further showcasing the power of quantum computation. These algorithms served as very tangible proof of concept for the theoretical foundations laid earlier.
As the years progressed, experimental efforts also gained momentum. The creation of several small-scale quantum computers began around the 2000s. These developments marked the transition from theoretical models to practical systems capable of executing quantum algorithms. Companies like IBM and Google entered the fray, focusing on developing and commercializing quantum computing technology.
The announcement of Google's supremacy experiment in 2019 further solidified the field's momentum, as it demonstrated quantum computing's potential to perform tasks quicker than classical counterparts. This ongoing journey of milestones highlights an evolving understanding of quantum principles and their application, setting the stage for future breakthroughs.
Quantum computing is at the intersection of physics and computer science, driven by milestones that validate its potential and theory.
The historical perspectives on quantum computing are vital. They illustrate how ideas can crystallize over time into practical technology, hinting at the future landscape of computation.
Fundamental Principles of Quantum Mechanics
The principles of quantum mechanics form the backbone of quantum computing. Understanding these fundamental ideas is critical because they distinguish quantum systems from classical ones. As we navigate this complex realm, we uncover not only how quantum computers operate but also the unique properties that enable them to solve problems that are practically insurmountable for classical computers.
Quantum mechanics introduces several essential concepts. Key among them are superposition, entanglement, and quantum interference. Together, these principles change how we perceive information and computation. They also offer significant benefits over traditional computational methods. For instance, a quantum computer's superposition allows it to consider multiple states simultaneously, vastly improving efficiency for certain calculations. This efficiency is pivotal in various applicationsβfrom cryptography to complex simulations in physical sciences.
Consideration of these principles also leads to challenges in development. Quantum stability and error rates arise directly from the foundational concepts. Thus, a profound understanding of these principles is not only beneficial but necessary to navigate the challenges ahead.
"Quantum mechanics does not simply provide a new theory; it offers a fundamentally different perspective on reality itself."
Superposition
Superposition is a phenomenon that allows quantum bits, or qubits, to exist in multiple states at once. Unlike classical bits, which can be either 0 or 1, qubits can represent both 0 and 1 simultaneously. This capacity is what grants quantum computers their exceptional power. By leveraging superposition, a quantum computer can perform numerous calculations in parallel, which accelerates solving complex problems significantly.
The implications of superposition extend beyond speed. They also enhance computational capacity. When a quantum system is in superposition, it can be thought of as performing multiple operations at once. Thus, it fundamentally alters the landscape of algorithm design. For example, Shor's Algorithm benefits from superposition to factor large numbers efficiently, a task that would require extensive time on classical systems.
Entanglement
Entanglement is another cornerstone of quantum mechanics. When qubits become entangled, the state of one qubit is directly related to the state of another, regardless of the distance separating them. This correlation can lead to faster communication and data processing capabilities. The entangled state means that measuring one qubit immediately affects its counterpart, which can enable quicker computations and more robust information transfer.
The application potential of entanglement in quantum computing is vast. For instance, it is integral to the implementation of algorithms for quantum teleportation and in developing quantum networks. These applications expand beyond theory and into practical solutions for enhancing current technologies.
Quantum Interference
Quantum interference describes the phenomenon where the probability amplitudes of quantum states can add up or cancel each other out. This principle is crucial in optimizing quantum algorithms. By precisely manipulating the phases of quantum states, one can enhance the outcomes of a computation while minimizing errors.
Interference plays a significant role in various quantum algorithms. It enables the formation of constructive interference patterns, which boost the likelihood of correct answers emerging more prominently than incorrect ones. This aspect is essential in quantum Fourier transforms and Grover's Algorithm, which relies on interference to speed up search processes.
Quantum Bits: Qubits
Quantum bits, or qubits, represent a fundamental building block of quantum computing. Understanding qubits is essential to grasp how quantum computers operate differently compared to classical computers. The concept of qubits challenges the traditional notions of information processing and storage, revealing a more complex interplay of states and possibilities.
Definition and Characteristics
A qubit is the quantum equivalent of a classical bit. Where a classical bit can exist in one of two states, namely 0 or 1, a qubit can exist in a state of 0, 1, or both simultaneously, due to a property called superposition. This unique characteristic allows quantum computers to process vast amounts of information at much higher speeds than classical computers.
Moreover, a qubit can be implemented through various physical systems, such as photons, electrons, or atoms. Each system offers distinct advantages in terms of manipulation and measurement. For instance, superconducting qubits are widely used in several quantum processors today due to their relatively high coherence times.
A major characteristic of qubits is entanglement. When qubits become entangled, the state of one qubit becomes dependent on the state of another, regardless of the distance separating them. This can lead to significant enhancements in computational power and communication security.
Comparison to Classical Bits
In the realm of traditional computing, classical bits either take the form of 0s or 1s. Quantum bits, however, function quite differently:
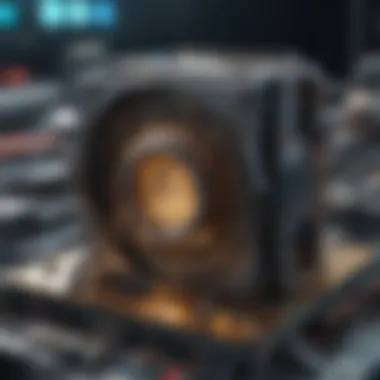
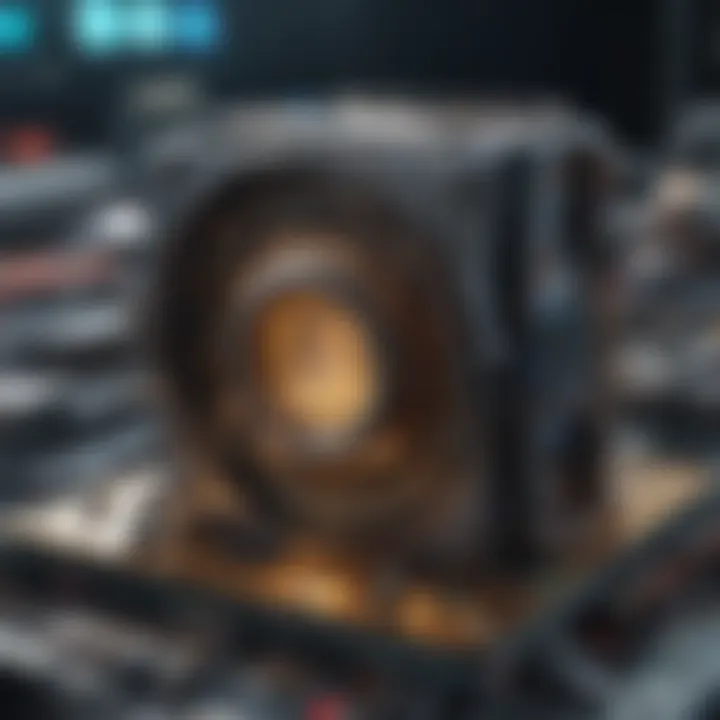
- State Representation: Classical bits represent information in a binary system, while qubits can represent multiple states simultaneously due to superposition.
- Processing Capability: A quantum computer can simultaneously perform computations on multiple combinations of qubits. Classical computers, in contrast, must execute each combination sequentially.
- Entanglement: Classical bits do not maintain relationships over distance in the same manner as qubits. The entanglement of qubits allows for correlations that can enhance processing speed and capability.
"The key to quantum computing lies in how we utilize qubits to understand the complex landscape of computation beyond classical limits."
Understanding these concepts about qubits is vital for anyone entering the field of quantum computing, as they highlight how and why this new technology stands to redefine the future of computation.
Quantum Algorithms
Quantum algorithms are pivotal in the realm of quantum computing. They harness the unique properties of quantum mechanics to perform computations that traditional algorithms struggle with. This section will delve into several key quantum algorithms, discussing their implications and the advantages they bring to various domains.
Shor's Algorithm
Shor's Algorithm represents a breakthrough in the realm of factorization of large integers. Developed by Peter Shor in 1994, this algorithm can factor numbers exponentially faster than the best-known classical algorithms. This characteristic makes it of great interest, particularly in the field of cryptography.
Traditional encryption methods, such as RSA, which relies on the difficulty of factoring large numbers, would be rendered obsolete if a functional quantum computer can implement Shor's Algorithm efficiently. The implications reach far into data security, banking systems, and online communications. As such, understanding Shor's Algorithm is essential for grasping the potential threats and changes in cybersecurity frameworks as quantum computing advances.
Grover's Algorithm
Grover's Algorithm is another significant quantum algorithm that optimizes search processes. Designed by Lov Grover in 1996, it offers a quadratic speedup for unstructured search problems, which implies finding a specific item in an unsorted database. This contrasts with classical approaches necessitating linear search time.
The ability to cut down search time has real-world applications that reach into various fields, such as database search, cryptography, and even machine learning. For example, Grover's Algorithm can make brute force attacks on encryption significantly faster, highlighting the necessity for new cryptographic methods as quantum computing evolves. The potential applications warrant careful consideration and proactive measures.
Quantum Simulation Techniques
Quantum simulation techniques leverage quantum computers to simulate physical systems, a task notoriously complex for classical computers. By utilizing quantum bits, which exist in superpositions, these simulation techniques can model quantum phenomena efficiently. This capability is crucial in fields such as materials science, where understanding molecular interactions is vital to creating new materials and drugs.
Simulating quantum systems provides researchers with insights that would be otherwise unattainable. For example, it can help predict the behavior of superconductors or elucidate reaction dynamics in chemical processes. These techniques open new avenues for exploration in scientific research and innovation.
Quantum algorithms are more than just theoretical constructs; they are practical tools that may transform industries and scientific research.
Current State of Quantum Computing Technology
The current state of quantum computing technology represents a dynamic and rapidly evolving frontier in the broader field of computation. As the knowledge of quantum mechanics translates into practical applications, researchers and companies alike have invested significant resources into developing viable quantum systems. These advancements challenge traditional paradigms of computing and open up unexplored possibilities that could affect various industries. Understanding this state is crucial for comprehending the future trajectory of technology and its potential impact on both society and scientific progress.
Leading Quantum Computing Firms
Several companies are at the forefront of quantum computing research and development. Among these, Google, IBM, and Rigetti Computing have made substantial strides in creating quantum processors and software that utilizes quantum principles effectively.
- Google has focused on achieving quantum supremacy, demonstrating that quantum computers can solve problems unfeasible for classical machines.
- IBM has developed the IBM Q platform, which provides access to its quantum processors through cloud technology, enabling wider access for researchers.
- Rigetti Computing emphasizes quantum cloud computing, focusing on integrating quantum processors with classical systems for hybrid computing solutions.
Other noteworthy players include D-Wave Systems, whose systems are known for quantum annealing, and Xanadu, which explores photonic quantum computing.
Technological Advancements
Technological advancements in quantum computing have mostly centered around improving qubit coherence times and error rates. These technical improvements enhance the reliability and scalability of quantum systems.
Moreover, breakthroughs in quantum error correction are also pivotal, as they directly impact the performance of quantum algorithms. Machine learning integration into quantum systems, enabling better data analysis methods, has become another area of research. These advancements are crucial, as they lay the groundwork for more practical applications of quantum computing in the real world.
Quantum Hardware Types
Quantum hardware can be broadly categorized into a few types based on the physical implementation of qubits. Each type presents unique advantages and challenges. The primary categories include:
- Superconducting Qubits: These are widely used by companies like IBM and Google. They allow fast gate operations and significant scalability but are sensitive to external interference.
- Trapped Ions: IonQ and Honeywell are key players here. This method provides high fidelity in operations but poses challenges in scalability.
- Topological Qubits: Microsoft's approach centers on these qubits. They promise robustness against certain types of errors, although they are still largely theoretical at this stage.
- Photonic Qubits: Used in Xanaduβs systems, these qubits exploit light particles for computation, allowing for integration into existing telecommunication systems.
The understanding of these various hardware types is essential for identifying which technologies may prevail as quantum computing moves toward more practical applications.
"The journey in quantum computing needs not only innovation in technology but also collaboration among researchers and industries to solve existing challenges and reach its full potential."
Applications of Quantum Computing
Quantum computing holds profound promise across various sectors. Its capability to process information in radically new ways leads to potential breakthroughs in traditional areas. This section delves into three crucial applications: cryptography, drug discovery, and optimization problems. Each application highlights the transformative impact of quantum computing, showcasing its potential to solve complex problems that current classical systems cannot efficiently address.
Cryptography
Cryptography is a fundamental aspect of data security. Traditional methods often rely on the complexity of mathematical problems, such as factoring large integers. Quantum computing can fundamentally change this landscape.
Shor's Algorithm indicates that, with sufficient qubits, quantum computers can factor these massive numbers exponentially quicker than classical computers. This capability poses a challenge to current encryption methods since many public key systems hinge on this difficulty. The implications are significant.
Organizations must consider transitioning to quantum-resistant algorithms to secure sensitive data from future quantum threats. The development of new cryptographic techniques like lattice-based and hash-based systems aims to anticipate this shift.
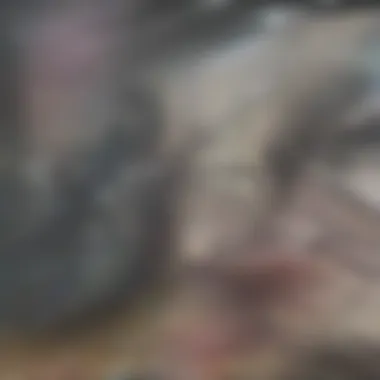
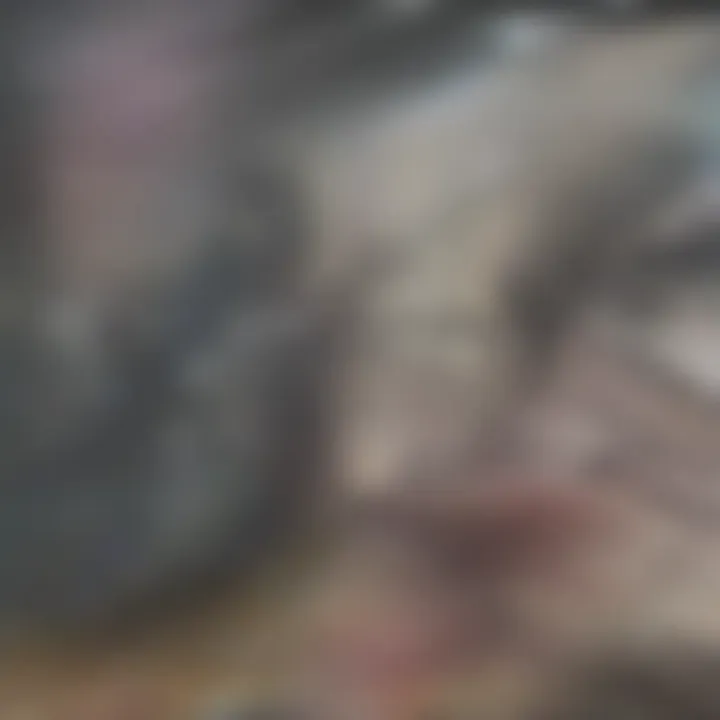
Drug Discovery
The field of drug discovery is extremely resource-intensive, often requiring years of research and development. Quantum computing can expedite this process through its unique ability to simulate molecular interactions at quantum levels. By utilizing quantum simulations, researchers can model complex biological systems more accurately.
For instance, D-Wave Systems is working on quantum approaches that might allow scientists to predict how drugs interact with their target proteins. This could substantially reduce the time and cost of drug development, potentially leading to quicker delivery of new medications to the market.
In summary, quantum computing may enable personalized medicine, where treatments are tailored to individual genetic profiles.
Optimization Problems
Many industries face optimization challenges, from logistics to finance. Quantum computing offers a novel way to approach these problems by evaluating numerous possibilities simultaneously through its principles of superposition. For instance, in supply chain management, companies can optimize routes, reducing time and costs by assessing complex variables with ease.
In financial modeling, algorithms can analyze large datasets to optimize trading strategies, portfolio allocations, and risk management. Major firms are already exploring how quantum algorithms can enhance their operational efficiencies.
The use of quantum computing for optimization issues not only promises reduced costs but also paves the way for innovative solutions previously thought unattainable.
"Quantum computing could reshape industries, providing solutions faster than we thought possible."
By tapping into these diverse applications, quantum computing can significantly enhance processes across various domains. The ongoing advancements in this field will likely lead to more practical implementations and foster further research, underscoring the sustained interest and investment in quantum technologies.
Challenges in Quantum Computing
The development of quantum computing presents significant challenges that researchers and engineers must overcome. Understanding these challenges is crucial, as they directly influence the pace of technological progress and the feasibility of practical applications. This section focuses on two main areas of concern: error rates and scalability issues.
Error Rates and Quantum Stability
One of the foremost challenges in quantum computing is the issue of error rates. Quantum bits, or qubits, are notoriously susceptible to errors due to decoherence and noise. Decoherence is when a qubit loses its quantum state, which can occur through interactions with the environment. Even slight environmental changes like temperature fluctuations or electromagnetic interference can lead to catastrophic outcomes in a computation.
Here are several key aspects to consider regarding error rates:
- Quantum Error Correction: There are methods being investigated to correct errors without measuring the quantum state directly. These methods include the use of redundant qubits to encode information, but they come with increased resource demands.
- Stability of Qubits: Different types of qubits (like superconducting qubits and trapped ions) have varying levels of stability and susceptibility to errors, impacting system design choices based on use cases.
- Impact on Computation: High error rates extend the required computation time, making it less practical for complex tasks that are expected to run efficiently.
"Quantum computing requires a delicate balance between maintaining qubit coherence and executing operations accurately, making error management a top priority in research."
Scalability Issues
Scalability is another critical challenge. As the demand for more powerful quantum computers grows, so does the need to build larger systems of qubits. Familiar scaling challenges arise in both physical hardware and algorithmic processes. Typical concerns include:
- Physical Limitations: Current technologies to create and manipulate qubits often face limits in size and performance. Assembling a functional quantum computer with thousands of qubits is not trivial, given the complexity of managing those individual qubits.
- Interconnectivity: Linking numerous qubits in a manner that maintains their quantum state is complex and often results in increased error rates. Effective quantum communication is required to minimize interference and maintain stability.
- Resource Management: The power requirements for cooling and managing quantum systems can become prohibitive, especially as we scale up.
The path toward resolving these challenges is fraught with obstacles, but continuous advancements in technology, materials science, and quantum theory offer hope for substantial breakthroughs. A clear vision and ongoing research will be vital for overcoming these hurdles and fully harnessing the power of quantum computing.
Future of Quantum Computing
The future of quantum computing is of paramount significance within the context of this article on understanding quantum computing. This section discusses the advancements we can expect, potential challenges that may arise, and the broader implications on various aspects of life. As technology continues to evolve, quantum computing holds promises that could redefine computational capabilities, reasoning, and problem-solving approaches. Knowing the trajectory of quantum computing is essential for students, researchers, and professionals aiming to grasp the evolving landscape of technology.
Predictions and Trends
Several predictions about the future of quantum computing have surfaced, driven by ongoing research and development. Here are some notable trends to consider:
- Increased Availability of Quantum Resources: Firms like IBM, Google, and Rigetti are investing heavily in quantum technology. Future generations of quantum computers will likely become more accessible to the general public, with cloud-based quantum computing platforms emerging.
- Advancements in Quantum Algorithms: As the field matures, we can expect new quantum algorithms to evolve, improving problem-solving efficiency. These algorithms may enhance tasks in cryptography, artificial intelligence, machine learning, and optimization problems.
- Hybrid Quantum-Classical Systems: A trend toward integrating classical and quantum systems will develop. This hybrid approach can utilize the strengths of both types of computing, making it easier to solve complex real-world problems.
- Focus on Error Correction: Researchers are prioritizing quantum error correction techniques. As these methods improve, the stability and reliability of quantum systems should see a noticeable enhancement.
- Industry-Specific Applications: Industries such as finance, pharmaceuticals, and logistics are likely to increasingly rely on quantum computing to gain competitive advantages through enhanced simulations and optimizations.
Potential Societal Impacts
The societal implications of quantum computing can be profound and multifaceted, influencing various domains:
- Transformational Changes in Security: With the rise of effective quantum algorithms, particularly in cryptography, traditional encryption methods could become obsolete. This shift demands a transition toward quantum-safe cryptography to ensure data protection in the information age.
- Healthcare Advancements: Quantum computing may expedite drug discovery and development processes. This could result in efficient modeling of molecular interactions, leading to breakthroughs in treatments and vaccines.
- Environmental Sustainability: Quantum computing can optimize energy systems and improve logistics, leading to reduced waste and more efficient resource management. This advancement has the potential to help in combating climate change and improving sustainability efforts.
- Economic Shifts: As quantum computing technologies advance, new job roles will emerge in quantum programming, hardware maintenance, and research. This could spark a new economic sector while also changing existing employment landscapes.
"The true promise of quantum computing lies not just in faster computations, but in the potential to solve problems previously deemed unsolvable."
Epilogue
In this article, we have traversed the world of quantum computing, uncovering its intricacies and significance. The conclusion is a crucial element in summarizing the complexity of a topic such as quantum computing, allowing for reflection on the material covered and its broader implications.
Understanding quantum computing is no longer an academic luxury; it holds profound importance in shaping future technologies. Reflections in this concluding section aim to encapsulate the essence of what has been discussed, stressing key elements and benefits of this field. This includes the fundamental principles of quantum mechanics, the nature of qubits, the power of quantum algorithms, and the current advancements in technology.
As industries are confronted with complex problems that classical systems struggle to solve, quantum computing presents an avenue for unprecedented efficiency and capability. It is essential to consider how this technology may transform sectors such as cryptography, optimization, and drug discovery.
A few key points to highlight in our summary include:
- The foundational concepts of quantum mechanics and their practical applications.
- The pioneering algorithms that leverage quantum properties for problem-solving.
- The notable challenges facing the field, including error rates and scalability issues.
These factors underscore the urgency for ongoing research and development in quantum technologies, signaling a shift in how we approach computational problems.
"Quantum computing is a powerful tool that will change the landscape of technology, impacting every aspect of modern life."
As we continue to explore the possibilities of quantum computing, it is evident that the implications of this research extend beyond academia and industry. The diverse scope of applications may influence economic structures, national security frameworks, and healthcare innovations.
Thus, the conclusion serves not merely as a final thought but as an invitation to look ahead at the exciting possibilities within quantum computing.