Understanding Quantum Computer Technology: A New Era
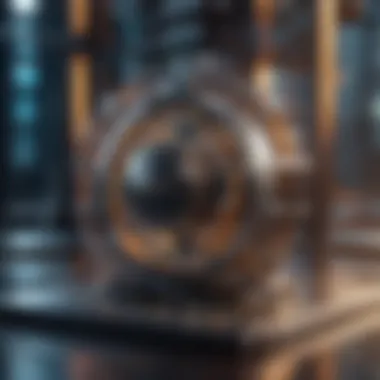
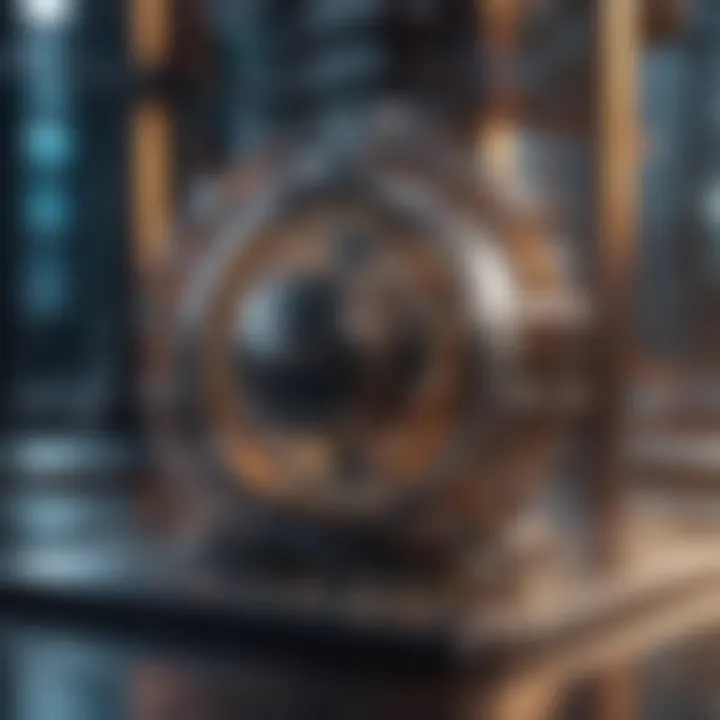
Intro
Quantum computing heralds a significant transformation in the capabilities of technology. It stands apart from classical computing by utilizing the principles of quantum mechanics. This shift opens up new avenues for processing information, with implications touching numerous sectors from cryptography to materials science and artificial intelligence.
Key Research Findings
Overview of Recent Discoveries
Recent studies have unveiled critical advancements in quantum computing. Researchers have made substantial progress in both hardware and algorithms. For instance, breakthroughs in error correction techniques prove vital for sustaining quantum states long enough for complex calculations. Companies like IBM and Google lead in developing quantum processors with increasing qubit counts, representing a significant leap in computational power.
Notably, the exploration of quantum entanglement has demonstrated potential uses in secure communications. In addition, quantum supremacy has been partially realized, which shows quantum computers can outperform classical ones in specific tasks.
Significance of Findings in the Field
These discoveries underscore the relevance of quantum computing in modern technology. The potential to solve problems previously deemed intractable captures the attention of researchers and industries alike. Fields like cryptography are facing a paradigm shift as algorithms such as Shor's Algorithm challenge traditional cryptographic protocols.
Moreover, sectors such as materials science stand to benefit vastly from quantum simulations, which allow for the modeling of molecular interactions at unprecedented accuracy. This might lead to the creation of new materials with tailored properties.
Breakdown of Complex Concepts
Simplification of Advanced Theories
Understanding quantum computing often involves grappling with complex theories such as superposition and entanglement. Superposition allows qubits to exist in multiple states simultaneously, unlike classical bits, which are either 0 or 1. This characteristic exponentially increases processing power. Entanglement, on the other hand, provides a unique correlation between qubits that can significantly enhance computational capabilities.
To simplify these ideas:
- Qubits can be seen as the building blocks of quantum computers, much like bits in classical computers.
- Superposition enables qubits to represent numerous states at once.
- Entanglement creates dependencies between qubits, allowing for complex computations to happen in parallel.
Visual Aids and Infographics
Visual aids can enhance understanding of these concepts. Diagrams illustrating the quantum states of qubits or infographics depicting quantum circuits lay out the architecture of quantum computers. These representations make the intricate theories more accessible for various audiences, particularly students and professionals new to the field.
"Quantum computing is more than an evolution; it represents a revolution in computational capabilities."
By delving into the latest research and efforts in quantum computing, it becomes clear that we are on the cusp of a new era in technology. The journey requires understanding both the fundamental principles and the ramifications of these advancements.
Intro to Quantum Computing
Quantum computing is an emerging field that harnesses the peculiar phenomena of quantum mechanics. This technology promises to revolutionize computing by performing complex calculations at unprecedented speeds. Understanding its foundations is critical for grasping how it can impact various sectors, from healthcare to cybersecurity.
The importance of introducing quantum computing lies in its potential to solve problems that are currently intractable for classical computers. Unlike traditional bits that represent either 0 or 1, quantum bits, or qubits, can exist in multiple states simultaneously. This unique property allows quantum computers to process a vast amount of information concurrently.
Defining Quantum Computing
Quantum computing is a type of computation that utilizes the principles of quantum mechanics. In essence, it represents information using qubits instead of classical bits. A qubit can be in a state of 0, 1, or both simultaneously due to superposition. This ability allows quantum computers to perform multiple calculations at the same time, vastly improving processing capabilities.
Additionally, qubits can become entangled, meaning the state of one qubit can depend on the state of another, even when they are separated by large distances. This entanglement is crucial in enhancing the computing power of quantum systems. Quantum computing fundamentally changes how we think about computational tasks, making certain calculations, such as factoring large numbers or simulating quantum systems, exponentially faster than classical approaches.
Historical Context
The journey of quantum computing began in the 1980s. Pioneers like Richard Feynman and David Deutsch envisioned utilizing quantum mechanics to emulate physical systems. In 1994, Peter Shor developed what is now know as Shor's Algorithm, which demonstrated that quantum computers could outperform classical ones in specific tasks, particularly in cryptography.
Over the years, significant advances have been made. In the 2000s, IBM and Google, among others, started investing heavily in quantum research. Fast forward to today, companies like D-Wave and Rigetti Computing offer cloud-based quantum computing services. These developments mark a substantial shift from theoretical curiosity to practical applications, establishing a foundation for the future of quantum technology.
"Understanding quantum computing is essential for grasping the future of technology and information processing."
The exploration of quantum computing not only unveils the mechanics behind this technology but also its implications for industries that rely on data analysis and security. In summary, understanding quantum computing sets the stage for innovation, pushing the boundaries of what is computationally possible.
Fundamental Principles of Quantum Mechanics
Superposition
Superposition is a key principle in quantum mechanics that allows a quantum system, such as a qubit, to exist in multiple states at once. Unlike classical bits that can be either 0 or 1, qubits can be in a state that is both 0 and 1 simultaneously. This behavior enables quantum computers to process vast amounts of data in parallel, significantly increasing computational efficiency.
Understanding superposition is essential for designing quantum algorithms that can exploit this property. For instance, when a qubit is manipulated through quantum gates, it can effectively carry out many calculations at the same time. With this capability, tasks such as factoring large numbers or searching databases become exponentially faster. Quantum computers leverage superposition to achieve a level of performance unattainable by classical systems.
Entanglement
Entanglement is another critical principle of quantum mechanics that allows qubits to be interconnected in such a way that the state of one qubit can instantly affect the state of another, regardless of the distance between them. This phenomenon is not only intriguing but also serves practical purposes in quantum computing.
When qubits become entangled, they can share information in a manner that classical bits cannot. This allows for faster processing and secure communication channels. In quantum cryptography, for example, entangled qubits can be used to create unbreakable encryption keys. Thus, understanding entanglement is essential in both the design of quantum algorithms and the development of advanced communication systems.
"Entangled qubits are like a digital handshake that ensures secure information exchange at phenomenal speeds."
Quantum Interference
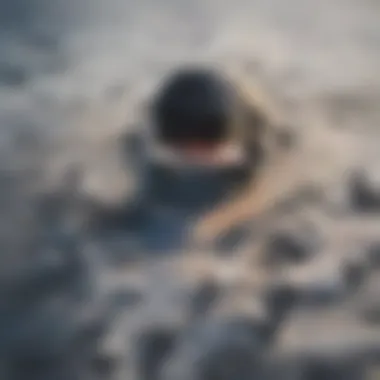
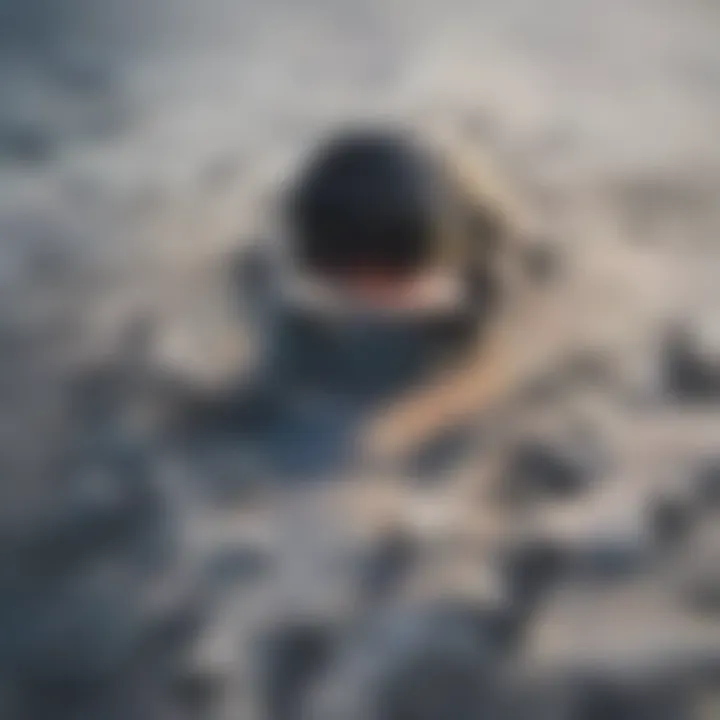
Quantum interference occurs when quantum states combine, leading to probabilities that can amplify or diminish particular paths of computation. This principle is crucial in quantum algorithms, where the goal is to enhance the probability of correct outcomes while reducing the chances of incorrect ones.
By manipulating the phases of quantum states, quantum computers can use interference to guide calculations toward favorable results. This principle is exemplified in algorithms like Shor's Algorithm, which employs interference to find the prime factors of large numbers efficiently. Without the concept of quantum interference, many of the advantages offered by quantum computation would not be possible.
In summary, the Fundamental Principles of Quantum Mechanics β especially superposition, entanglement, and quantum interference β lay the groundwork for quantum computing. They not only define how quantum computers operate but also illustrate their potential to revolutionize technology and various industries.
Quantum Bits: Qubits
Quantum bits, or qubits, are the foundational units of information in quantum computing. Their unique properties set them apart from classical bits, making them crucial for the vast capabilities of quantum computers. A classical bit can be either 0 or 1, but a qubit can exist in multiple states simultaneously, due to the principle of superposition. This allows quantum computers to perform complex calculations at speeds unattainable by their classical counterparts.
The exploration of qubits is vital, as it directly influences the effectiveness and efficiency of quantum computing. Understanding qubits provides insights into how quantum computers can tackle problems that challenge classical systems. Researchers and practitioners must familiarize themselves with the characteristics and types of qubits to harness their potential fully.
Qubit Definition and Characteristics
A qubit is the quantum version of a classical bit. It represents the state of a quantum system and can be visually understood as a vector on the Bloch sphere, which demonstrates the qubitβs ability to be in a state of 0, 1, or any quantum superposition of these states. The characteristics of qubits include:
- Superposition: This property allows qubits to be in multiple states at once, leading to parallel processing capabilities.
- Entanglement: Qubits can become entangled, meaning the state of one qubit is directly related to the state of another, regardless of distance.
- Decoherence: This refers to the loss of quantum coherence, which can limit the time a qubit can maintain its state before being observed or interfered with, presenting substantial challenges in quantum computing.
Types of Qubits
Several types of qubits exist, each with their distinct features and potential applications in quantum computing.
Superconducting Qubits
Superconducting qubits operate at very low temperatures and leverage the properties of superconductivity. They are highly regarded due to their scalability and compatibility with existing technologies. The key characteristic of superconducting qubits is their ability to perform rapid quantum operations, making them a popular choice for many current quantum computers.
One unique feature of superconducting qubits is their relatively fast gate speeds. However, they are susceptible to errors due to external disturbances, requiring sophisticated error correction methods to maintain qubit stability. Despite these challenges, superconducting qubits continue to be a front-runner in the development of quantum processors.
Ion-Trapped Qubits
Ion-trapped qubits utilize individual ions confined in electromagnetic fields. This method allows for precise control of qubit states through laser interactions. The key characteristic of ion-trapped qubits is their long coherence times, which facilitate extended processing periods without significant loss of information.
A notable feature of ion-trapped qubits is their ability to implement complex quantum gates with high fidelity. However, the scalability of this system can be a challenge, as creating larger arrays of trapped ions requires refined technology and precision. Still, ion-trapped qubits have shown great promise in achieving quantum supremacy.
Topological Qubits
Topological qubits present a novel approach to quantum computing, relying on the principles of topology to create robust qubit states. Their key characteristic is the inherent protection against local noise, which enhances their stability and coherence.
The unique feature of topological qubits is their ability to harness braiding operations of particles, known as anyons, to perform quantum computations. This adds an additional layer of security against decoherence, which is particularly attractive in building large-scale quantum systems. However, practical realization is still in the research phase, requiring more breakthroughs to establish them as a mainstream choice in quantum computing.
As quantum computing evolves, understanding qubits and their types is essential for realizing the full potential of this technology in various fields, including cryptography, drug discovery, and artificial intelligence.
Architecture of Quantum Computers
The architecture of quantum computers is pivotal to understanding how they operate and the advantages they bring over classical systems. This architecture incorporates various elements such as quantum gates, circuits, and algorithms, which work together to perform computations that are otherwise infeasible.
Understanding this architecture is not just for academic purposes; it is also essential for grasping the future potential of computational technology. As this field grows and develops, the nuances of quantum architecture can provide insights into potential breakthroughs and applications across different sectors.
Quantum Gates
Quantum gates are the basic building blocks of quantum circuits. Similar to classical logic gates, quantum gates manipulate the qubits, changing their state in a controlled manner. However, the operations employed in quantum gates differ significantly from classical gates due to the principles of quantum mechanics.
One important aspect of quantum gates is their ability to process complex information through manipulations of superposition and entanglement. In practical terms, quantum gates execute operations by applying mathematical transformations to qubits, enabling parallel computation. For instance, common quantum gates include the Hadamard gate, Pauli-X gate, and CNOT gate, each performing specific functions in a quantum processor system.
Quantum Circuits
In quantum computing, a quantum circuit refers to a model used for quantum computation, which consists of gates applied to qubits. The circuit's design dictates the flow of information, and its implementation can affect the overall efficiency of computation.
Quantum circuits are often implemented in a layer structure, allowing multiple operations to occur simultaneously. This layering enhances the computational speed and allows for complex calculations. The layout and connectivity of quantum circuits significantly influence the capability of algorithms executed within these circuits.
Quantum Algorithms
Quantum algorithms are specifically designed to run on quantum computers and exploit their unique properties.
Shor's Algorithm
Shor's Algorithm is a revolutionary quantum algorithm that addresses integer factorization. This algorithm allows a quantum computer to factor large integers exponentially faster than the best-known classical algorithms. Its importance lies in its potential to break widely used cryptographic systems, like RSA, making it critical for security discussions in the tech community.
A key characteristic of Shor's Algorithm is its method of exploiting quantum parallelism via quantum Fourier transforms. Consequently, it represents a landmark capability of quantum computation. However, its challenges include the requirement for fault-tolerant quantum systems to function effectively.
Grover's Algorithm
Grover's Algorithm focuses on unstructured search problems. It offers a quadratic speedup for searching through unsorted data compared to classical methods. This efficiency makes Grover's Algorithm a popular choice for applications in database search, cryptography, and optimization.
A notable feature of Grover's Algorithm is its simplicity, involving only a few quantum operations to achieve its desired result. This simplicity is advantageous for implementation; however, its quadratic speedup, while impressive, does not scale as significantly as algorithms like Shor's for problems such as factorization.
In summary, the architecture of quantum computers, including elements like gates, circuits, and algorithms, provides foundational insight into how these systems function and their transformative potential across multiple domains.
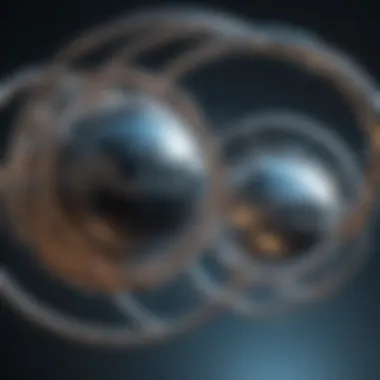
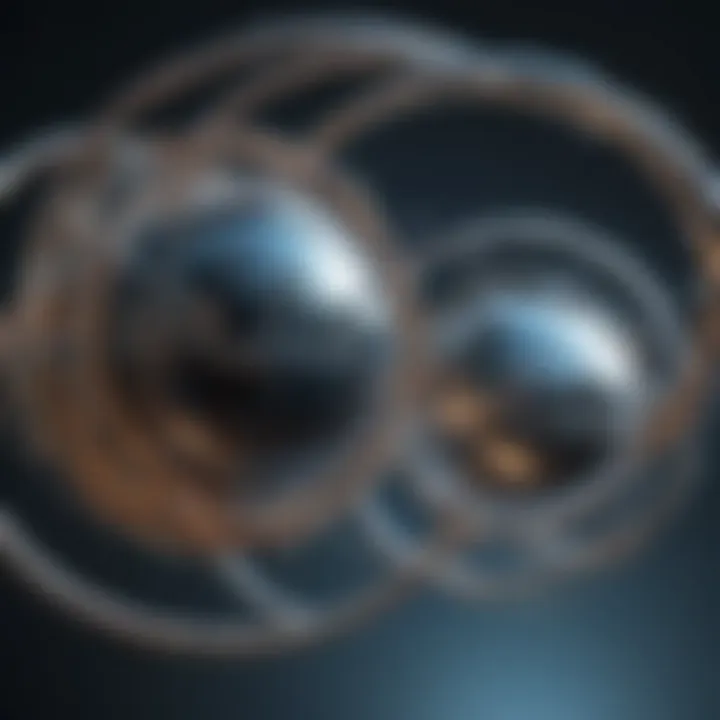
Comparison with Classical Computing
In the realm of computing, understanding how quantum computers differ from classical systems is crucial. This comparison not only highlights the benefits of quantum technology but also frames the ongoing advancements in the field. Classical computers, which employ binary bits (0s and 1s) as their fundamental units of information, operate using logical processes defined by their architecture. They excel in many domains, particularly for tasks based on straightforward algorithms and large-scale data processing. However, their processing limitations become evident when faced with complex problems that require exponential computations.
Processing Power
Quantum computing introduces a fundamentally different approach to processing information. Utilizing quantum bits or qubits, these systems leverage the principles of superposition and entanglement. A qubit can exist simultaneously in multiple states (0, 1, or both), allowing quantum computers to perform multiple calculations at once. This capacity leads to a potential for processing power that is beyond the capabilities of classical computers. For instance, Shor's Algorithm enables the factoring of large integers in polynomial time, a task that is significantly longer for classical algorithms. This advantage positions quantum computing favorably in fields like cryptography where speed is essential.
Storage and Memory Capacity
Classical computers manage data through a defined structure, relying on memory like RAM and storage disks. Quantum computers, on the other hand, store information in a way that reflects the quantum state of their qubits. This allows quantum computers to handle an enormous amount of data with a relatively small number of qubits. For example, while eight classical bits can only represent 256 distinct values, eight qubits can represent 256 distinct states simultaneously due to superposition.
However, the practical implementation of this storage capacity is still emerging, as it faces challenges like decoherence, which affects how long qubits can maintain their quantum state.
Applications and Use Cases
The distinctions in processing power and memory capacity between classical and quantum computers lead to varied applications in real-world scenarios. While classical computing remains optimal for everyday tasks and general computing needs, quantum computing opens doors to areas that require unparalleled analytics and processing speed.
- Cryptography: The implications for cryptographic security are immense, as quantum computers could break existing encryption methods. However, they also lead to the development of quantum-safe encryption.
- Drug Discovery: In pharmaceuticals, simulating molecular interactions can revolutionize drug discovery processes, making them faster and more effective.
- Artificial Intelligence: In AI, quantum computing can improve machine learning algorithms, enhancing their ability to solve complex problems and analyze large data sets faster.
Quantum computing serves as a transformative technology that pushes the boundaries of what computational tasks are possible, particularly in complex problem-solving.
The ongoing development of quantum computers may well redefine how problems are approached across various sectors, illustrating the fundamental divide between classical and quantum computing paradigms.
Applications of Quantum Computing
Quantum computing stands at the forefront of modern technological advancements. It holds promise across various sectors. Understanding the applications of quantum computing is crucial. These applications extend beyond theoretical insights, impacting practical realms. They indicate how quantum mechanics can reshape industries.
Cryptography
One of the most discussed applications of quantum computing is cryptography. Quantum computers can revolutionize how data security is approached. Classical encryption methods, such as RSA, rely on the difficulty of factoring large numbers. However, with Shor's algorithm, a quantum computer could efficiently break these encryptions. This creates a pressing need for quantum-safe encryption methods.
In response, researchers are developing new cryptographic protocols. Quantum Key Distribution (QKD) is one such protocol. It uses quantum mechanics principles to securely share encryption keys. This method ensures that any attempt to intercept the key is detectable. As quantum technologies evolve, so too must our strategies for data protection. With the rise of quantum computing, the realm of cryptography faces profound changes.
Drug Discovery and Materials Science
In drug discovery and materials science, quantum computing shows significant potential. Simulating molecular interactions is complex for classical computers. Quantum computers have the ability to handle vast datasets and complex calculations. This capability can lead to faster identification of new drugs. Companies are exploring quantum algorithms that could predict molecular behavior accurately.
Furthermore, materials science can benefit as well. The quest for new materials often requires simulating atomic-level properties. Quantum computing can accelerate the discovery process. Potential applications include superconductors and nanomaterials. These advances could lead to breakthroughs in various industries, such as energy and electronics.
Artificial Intelligence
Artificial intelligence is another field poised for transformation through quantum computing. Machine learning algorithms often require significant processing power. Quantum computing can enhance these algorithms, enabling faster data processing. Techniques like quantum machine learning can analyze larger datasets more efficiently.
Additionally, quantum computers may optimize complex decision-making processes. For example, reinforcement learning, widely used in AI, could benefit from quantum techniques. This optimization extends to various applications, including robotics and automation.
The integration of quantum computing into AI could redefine how we approach intelligence in machines. Overall, the potential applications of quantum computing are vast and significant. Its influence spans multiple domains, signaling a shift in how we solve complex problems.
Current State of Quantum Computing Research
Quantum computing research is crucial in understanding the progress and potential of this exciting field. The current landscape showcases rapid advancements, increased investments, and a growing interest from various sectors. Researchers and organizations are racing to develop quantum systems that can outperform classical computers, signaling a paradigm shift in technology.
Leading Organizations and Institutions
Numerous organizations are at the forefront of quantum computing research. These entities contribute significantly to advancing the technology. Some notable players include:
- IBM: IBM has introduced its Quantum Experience platform, which allows researchers and developers to experiment with quantum algorithms and learn about quantum mechanics.
- Google: Google has made headlines with its quantum supremacy claim, showcasing a quantum computer that performed a specific computation faster than a classical supercomputer.
- Microsoft: Microsoft focuses on its Quantum Development Kit, simplifying the programming of quantum algorithms. Its research emphasizes scalability and practical applications.
- D-Wave Systems: D-Wave is notable for its early production of quantum annealers, targeting optimization problems across various industries.
- Rigetti Computing: Rigetti is a startup concentrating on building quantum processors and enabling cloud-based quantum computing.
- National Laboratories: Institutions like Lawrence Berkeley National Laboratory and Los Alamos National Laboratory contribute to fundamental research and collaboration with academic and industry partners.
These organizations play vital roles in shaping the future of quantum computing through significant funding, research initiatives, and collaborations, paving the way for practical applications.
Recent Breakthroughs
Recent breakthroughs in quantum computing research emphasize growth in various aspects, including error rates, algorithms, and practical applications. Notable advancements include:
- Improved Qubit Designs: Researchers have developed new qubit architectures that minimize errors and improve coherence times, essential for practical computation.
- Quantum Algorithms Development: Enhanced algorithms, like quantum versions of classical algorithms, are increasing efficiency in problem-solving. For instance, Grover's algorithm has been optimized to require fewer operations in searching databases.
- Commercial Availability: The introduction of commercial quantum computing services allows businesses to access quantum capabilities. Platforms offered by Amazon Braket and IBM Quantum are examples.
- Collaborative Research: Collaborative efforts between academia and industry are yielding promising outcomes. Projects like the Quantum Internet initiative aim to explore secure quantum communication.
"The advancements being made in quantum computing are not just theoretical; they are inching closer to practical applications across various domains."
This current state of research reflects a solid foundation for future advancements. As the quantum computing community forges ahead, a concerted effort to address challenges, such as error rates and scalability, will be vital for realizing the full potential of this transformative technology.
Practical Challenges in Quantum Computing
The field of quantum computing is marked by its immense potential, yet it faces a critical set of challenges that must be addressed for progress to continue. The practical challenges in quantum computing are not just technical hurdles but also fundamental barriers that influence the trajectory of research and development. Understanding these challenges helps in grasping the limitations of current technologies and the innovative strides needed to overcome them.
Error Rates and Decoherence
One of the primary obstacles in quantum computing is the high error rates associated with qubits. Qubits, the building blocks of quantum computers, are highly susceptible to interference from their environments. This interference can lead to decoherence, a phenomenon where the quantum state of a qubit becomes disturbed, thereby losing its information. Such instability makes reliable computation challenging.
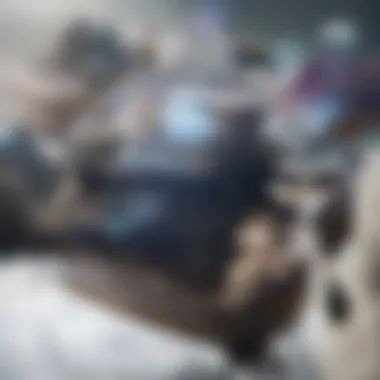
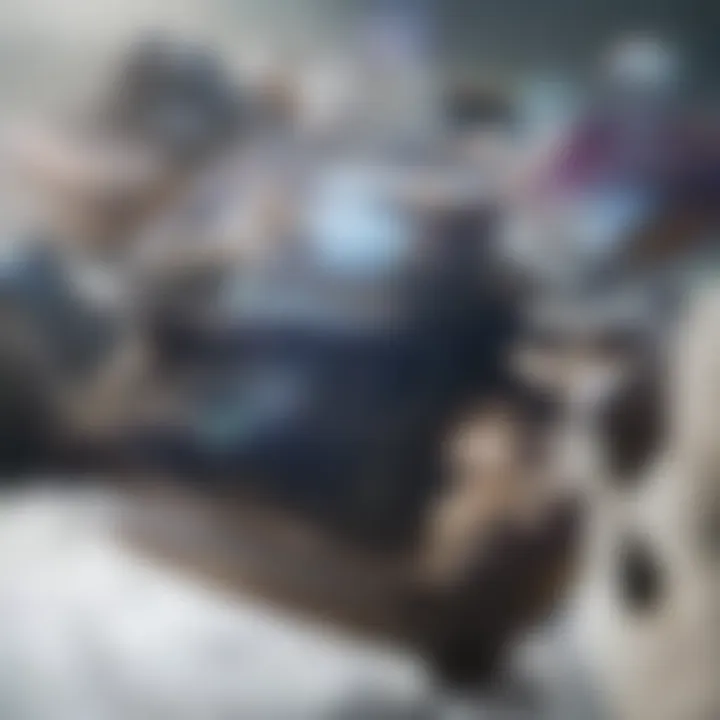
Error correction is a crucial area of research that seeks to mitigate these issues. Techniques like the Shor code and surface codes aim to ensure that information can be preserved despite errors occurring. However, error correction methods also require additional qubits, which complicates the design and scalability of quantum processors. Reducing error rates to levels comparable to classical bits is essential before quantum computers can be practical for general use.
In summary, error rates and decoherence represent significant barriers that need ongoing investigation. Alone, they can thwart numerous calculations and applications that quantum computing promises to deliver.
Scalability Issues
Scaling quantum computers presents another major challenge. Current prototypes consist of a limited number of qubits, often fewer than several dozen. While small-scale quantum computers can demonstrate certain advantages in computational tasks, developing a scalable system is a different matter altogether.
Several factors play a role in the struggle for scalability:
- Qubit Interconnectivity: To harness the full potential of quantum computing, qubits must be able to interact effectively. However, increasing the number of qubits can lead to complications in maintaining coherent operations among them.
- Technological Constraints: Many existing technologies for qubit implementation, such as superconducting circuits or trapped ions, face physical limitations in how many qubits they can accommodate without significant losses in accuracy and coherence.
- Resource Requirements: As systems grow, the resources for control and error correction increase substantially, which raises costs and complicates infrastructure.
The journey toward a scalable quantum computing paradigm is ongoing. Each advancement brings researchers a step closer to realizing a fault-tolerant quantum computer that can outperform classical systems in a wide array of applications. Addressing scalability is vital for transitioning from experimental setups to usable quantum processors that can impact industries and scientific fields.
"The quest for practical quantum computing requires overcoming various intricate challenges, but the potential rewards are unparalleled."
As the field evolves, constant innovation in both theoretical and applied quantum mechanics is essential to navigate these complex challenges.
Ethical Considerations in Quantum Computing
The realm of quantum computing is not just a technological frontier but also a landscape rich with ethical dilemmas. As this technology evolves, the implications stretch beyond mere performance gains and delve into areas that affect society at large. Understanding these implications is paramount for students, researchers, educators, and professionals alike. The ethical considerations surrounding quantum computing encompass several critical elements, including security risks, the effects on employment, and broader societal impacts.
Security Implications
Quantum computers possess the potential to revolutionize data processing, but this capability also brings significant security concerns. The ability of quantum computers to solve complex mathematical problems at unprecedented speeds poses a direct threat to current encryption methods. For instance, Shor's algorithm can efficiently factor large integers, which directly undermines the popular RSA encryption algorithm. This capability could lead to vulnerabilities in financial systems, personal data, and national security frameworks. As quantum computing reaches maturity, the risk of cyber threats heightens, necessitating a comprehensive reevaluation of existing security protocols. Organizations must proactively seek quantum-resistant cryptographic methods to safeguard sensitive information. The urgency is clear: without preemptive measures, data protection may fall drastically short.
One possible approach is quantum key distribution (QKD), which leverages the principles of quantum mechanics for secure communication. While promising, these strategies require widespread adoption and implementation, resulting in a crucial area for ongoing research and development.
"As we venture deeper into quantum computing, the security implications demand our immediate attention and action."
Impact on Employment
The advent of quantum computing will inevitably reshape industries and the job market. As machines become capable of performing complex calculations faster than any classical computer, certain professions may become obsolete. For example, jobs that rely on routine data processing could face significant reductions. However, this does not mean all will be lost; rather, quantum computing offers new career opportunities requiring advanced skills in quantum algorithms, programming, and system architecture.
Upskilling and reskilling initiatives will be crucial in addressing potential job displacement. Educational institutions must adjust curricula to include quantum mechanics, coding languages specific to quantum computing, and interdisciplinary studies that merge computer science with ethics and security. By preparing the workforce for the future, society can mitigate the adverse effects of job loss while fostering innovation and progress.
Key aspects for consideration include:
- Reskilling programs: Focus on retraining current employees in industries affected by quantum advancements.
- Interdisciplinary education: The importance of merging quantum computing studies with ethics and policy discussions.
- Job creation: Highlighting new roles that will emerge within the quantum landscape, such as system analysts and software developers.
Ultimately, the trajectory of employment in the context of quantum computing rests on how society reacts to these challenges. It requires thoughtful planning and proactive measures to harness the benefits while minimizing the disruptions.
Future Prospects of Quantum Computing
The future of quantum computing holds significant promise and potential. As advancements continue, we may see transformations in technology that will alter the fabric of computing itself. This section explores predicted advances and their implications, focusing on elements that could reshape various industries and environments.
Predicted Advances
As we look ahead, several key advances in quantum computing are expected. These may include:
- Improved Qubit Stability: One of the most pressing challenges is enhancing the stability and coherence of qubits. Advances in material science could lead to qubits that can maintain quantum states for longer periods, thereby increasing the reliability of quantum computations.
- Error Correction Techniques: Effective error correction is essential for practical quantum computing. Researchers are working on new algorithms that can quickly identify and rectify errors in quantum systems, enabling more complex calculations.
- Enhanced Quantum Algorithms: Development of new quantum algorithms may open new avenues for quantum computing applications. This can lead to performances in simulation, optimization, and problem-solving that far exceed classical capabilities.
"The next decade will determine the real-world applications of quantum computers."
- Integration with Classical Systems: Future advancements may also focus on creating hybrid systems. This means combining the strengths of classical and quantum computing to achieve optimal performance in various tasks, such as data analysis or cryptography.
These advances will not just improve existing quantum computers but can also enable entirely new types of computing tasks that are currently unimaginable.
Potential Societal Impacts
The implications of quantum computing extend far beyond technological advancements. Society will likely see profound changes in various sectors:
- Healthcare Innovations: In the medical field, quantum computing could lead to breakthroughs in drug discovery and personalized medicine. By simulating molecular interactions at quantum levels, researchers can design more effective treatments faster and more efficiently.
- Revolution in Security: With the rise of quantum computing, the field of cybersecurity must evolve. Quantum encryption methods could make data transmission nearly impervious to hacking, raising standards of privacy and security.
- Economic Shift: As industries adopt quantum computing, markets may experience shifts in job requirements and skills needed. Educational institutions will need to adapt their curricula to prepare students for new roles that involve quantum technologies.
- Environmental Solutions: Quantum computing has the potential to address complex environmental models. It can help in optimizing resource management and climate modeling, providing insights that lead to sustainable practices.
Epilogue
The conclusion serves as the vital capstone in any nuanced exploration of quantum computer technology. It consolidates the unordered ideas discussed throughout the article, encapsulating critical insights about this revolutionary field.
Summation of Key Points
In reviewing the vast landscape of quantum computing, several essential elements emerge:
- Defining Characteristics: Quantum computing differs markedly from classical computing through key principles such as superposition and entanglement, driving its unique processing capabilities.
- Architectural Framework: Understanding the architecture is crucial. Quantum gates and circuits represent foundational constructs that dictate how quantum information is manipulated.
- Practical Applications: There exist real-world applications in cryptography, drug discovery, and artificial intelligence, illustrating the technology's transformative potential.
- Research Landscape: The current state of research demonstrates both urgency and promise. Leading organizations are making commendable advances, spurring innovation across multiple sectors.
- Ethical Dimensions: Considerations surrounding security and employment remain a pressing concern. A balanced approach is essential for navigating the implications of adopting quantum technologies effectively.
Call for Continued Research
Continued research in quantum computing is not merely beneficial but necessary. As the field advances, understanding and addressing the emerging challenges becomes paramount. Efforts must focus on the following:
- Decoherence Mitigation: Solutions need to be found for error rates and decoherence, which hinder the full realization of quantum capabilities.
- Scalability Improvements: Fostering scalable quantum systems is vital for enabling broader application and integration into current computational paradigms.
- Ethical Inquiry: Proactively addressing ethical concerns will provide a framework to guide responsible innovation.
Each of these areas signifies not just challenges but also avenues for fruitful discovery and exploration. Therefore, a collaborative and focused approach to research will amplify the potential benefits of quantum computing, paving the way for future breakthroughs.
"As quantum technology grows, so does our understanding of its implications; the future is as much about ethical foresight as it is about technological prowess."