Understanding Magnets and Magnetic Fields: A Comprehensive Guide
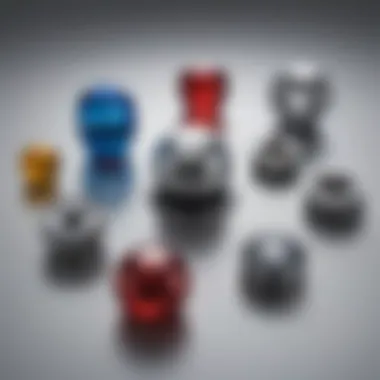
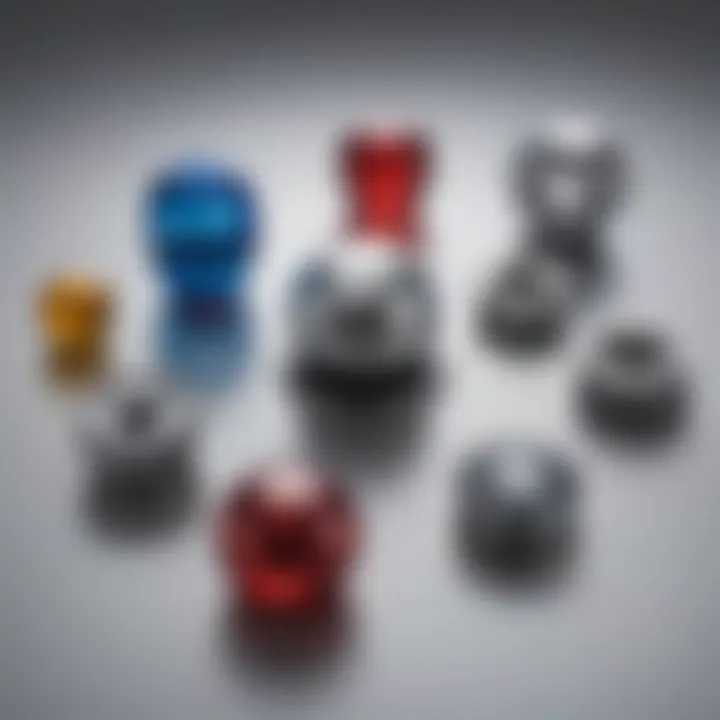
Intro
Magnets and magnetic fields are not just a set of scientific jargon; they are fundamental elements that shape various aspects of our daily lives. From the fridge magnets that hold up your children's drawings to the complex magnetic systems powering our modern technology, the implications of magnetic phenomena are vast and varied. Understanding the principles that govern these forces can illuminate pathways into fields ranging from physics to engineering and beyond.
In a nutshell, magnets are materials or objects that produce a magnetic field, which is an invisible force that can attract or repel other magnets and magnetic materials. This article aims to peel back the layers on magnets and magnetic fields, revealing how they function, their historical evolution, and their practical applications in contemporary society.
By going on this exploratory journey, we hope to address the intricate interplay between theory and application. Alongside, we want to create a resource that caters not just to students embarking on their scientific endeavors but also to educators seeking enriching content for instruction.
Let's delve deeper and begin by examining the Key Research Findings in this fascinating aspect of science.
Prologue to Magnets and Magnetic Fields
Magnets and magnetic fields are fundamental concepts that touch upon many aspects of both nature and technology. From the compasses that help travelers navigate the wilderness to the complex magnetic resonance imaging (MRI) machines used in hospitals, understanding the principles behind these phenomena is crucial. In this section, we will delve into what makes magnets tick and how magnetic fields come into play.
The importance of magnets goes beyond simple childhood fascination with refrigerator magnets. They are the silent partners in many mechanisms that drive modern technology. With applications ranging from data storage to electric motor functions, a comprehensive understanding of magnets and their interactions with magnetic fields lays the groundwork for comprehending many scientific and engineering principles. No matter your fieldโbe it physics, engineering, or educationโthe ideas we discuss will likely resonate with your professional endeavors.
Definition of Magnets
A magnet is defined as an object that produces a magnetic field. This magnetic field exerts forces on other magnets and magnetic materials, as well as affecting charged particles in motion. Essentially, magnets have two primary poles: north and south. When two magnets come together, they either attract or repel each other depending on the orientation of their poles. A good way to remember this is the phrase "opposites attract, likes repel." For example, if you bring the north pole of one magnet close to the north pole of another, they push away from each other. But a north pole and south pole will come together, demonstrating an attractive force.
Magnets can be divided into several categories: permanent magnets, temporary magnets, and electromagnets, each based on how they acquire their magnetic properties. For instance, permanent magnets maintain their magnetic characteristics over time, while temporary magnets only exhibit magnetism when they are in the presence of a magnetic field. On the other hand, electromagnets gain their magnetic properties through electric current, which makes them quite versatile.
Definition of Magnetic Fields
A magnetic field is the region around a magnet where magnetic forces can be observed. It can be visualized as a series of invisible lines that spread out from the magnetโs north pole and loop back around to its south pole. These lines indicate the direction of the magnetic force; they spread out into the space surrounding the magnet and become denser as one gets closer to it.
The strength of a magnetic field is measured in teslas (T), but in everyday scenarios, you might encounter the gauss (G), where 1 T equals 10,000 G. There are factors that can affect the intensity and orientation of a magnetic field, including the materialโs spacing and density.
Magnetic fields are not only confined to magnets. They occur in a variety of situations, such as the electric currents in wires and the planet's magnetic field, which protects us from harmful cosmic rays. Overall, understanding magnetic fields adds layers to your comprehension of how even the simplest magnets exert influence over their surroundings.
"Nothing is more powerful than a small group of committed individuals who can harness the power of magnetism to innovate."
In summary, magnets and magnetic fields constitute a vital area of study because they not only explain a wide range of physical phenomena but also provide practical solutions in technology and industry. With this foundation, letโs explore the historical context that shaped our comprehension of magnetism.
Historical Context
Understanding the historical context surrounding magnets and magnetic fields underscores the evolution of scientific thought, laying the foundation for practical applications we see today. This section gains significance not just for enthusiasts of physics but also for students and educators who are tasked with transferring this knowledge. The study of magnetism has roots that can be traced back to ancient civilizations, and these early explorations set the stage for later innovations. Delving into magnetic history offers valuable insights into how scientific methods developed, how theories were challenged and refined, and how serendipitous discoveries paved the way for modern technology.
Early Discoveries of Magnetism
Historically, magnetism began its journey in the mists of time when ancient Greeks and Chinese noted peculiar stones, now known as lodestones, that attracted iron. This natural phenomenon sparked curiosity and led to various myths and stories, weaving magnetism into the fabric of early scientific inquiry. The Greeks, especially Thales of Miletus around 600 BCE, heralded the earliest philosophical musings on magnetism. Lodestones fascinated people, who pondered their mysterious powers.
As the centuries passed, civilizations recognized practical applications of magnetism. By the Middle Ages, navigators used compassesโcrafted from lodestonesโto traverse the seas, altering trade routes and cultural exchanges entirely. These discoveries weren't just about functionality; they helped people gain a navigational understanding of the Earth itself.
Key Milestones:
- Thales of Miletus: Early observations around 600 BCE, emphasizing the lodestone's attraction.
- The Compass: Employed by sailors during the Middle Ages, enhancing sea travel.
- Scientific Exploration: Gradual transition from myths to empirical studies, setting the groundwork for future theories.
"From the first fascination with lodestones to the revolutionary compass, magnetism has shaped human endeavor."
Development of Magnetic Theory
The journey from discovery to theory is often paved with rigorous experimentation and intellectual debate. It wasn't until the 17th century that scholars like William Gilbert pioneered systematic studies of magnetism, distinguishing between magnetic forces and static electricity. His work, titled De Magnete, laid down foundational principles that would inspire future generations.
In the 19th century, the groundbreaking work of Hans Christian รrsted and later Andrรฉ-Marie Ampรจre moved the examination of magnetism beyond physical properties and into the realm of electromagnetism. รrsted demonstrated that electric currents could create magnetic fieldsโa pivotal revelation. This interconnection fueled theories around electromagnetic waves, culminating in the works of James Clerk Maxwell in the late 1800s, who formulated equations to describe electromagnetic phenomena in their entirety.
Development Highlights:
- William Gilbert: Author of De Magnete, establishing foundational magnetic principles.
- Hans Christian รrsted: First demonstration of the relationship between electricity and magnetism in the early 1800s.
- James Clerk Maxwell: Formulated laws linking electricity and magnetism, pivotal for modern physics.
Grasping the evolution of magnetic theory helps illuminate the connection between historical context and contemporary understanding, particularly relevant for today's educators aiming to enrich student perspectives on ongoing unearthing of scientific knowledge.
Types of Magnets
The distinction between various types of magnets forms a foundation for understanding magnetic applications in the real world. Each category of magnetโpermanent, temporary, and electromagnetsโcarries its unique characteristics and benefits, making them suitable for specific uses. Recognizing the significance of these differences allows students, researchers, and professionals to make informed choices about which type of magnet to use in their endeavors.
Permanent Magnets
Permanent magnets are fascinating creations of nature and engineering. They possess a stable magnetic field, which comes from the alignment of the magnetic domains during their manufacture. These domains are little regions where groups of atoms have their magnetic poles aligned in the same direction. Common materials for permanent magnets include neodymium, iron, and samarium, which are often mixed and processed to produce strong magnetic fields.
Their importance can't be overstated. Permanent magnets are widely used in everyday devices like refrigerator magnets, magnetic closures in purses and bags, as well as in high-tech applications like speakers and hard drives. By maintaining a consistent magnetic field without the need for electricity, they provide a reliable solution.
Key Characteristics of Permanent Magnets:
- Durability: They retain their magnetism over long periods, contributing to their utility in various applications.
- Simplicity: They do not require complex electrical systems, making them easier and cheaper to integrate into designs.
- Accessibility: They are available in various shapes and sizes, allowing for customization based on project needs.
Temporary Magnets
Temporary magnets are a different breed. These magnets are not always magnetic; they become magnetized when exposed to a magnetic field but lose their magnetism once the field is removed. Iron and nickel are commonly used materials for temporary magnets. Their application often involves situations where a magnetic field is needed only for a short time, which makes them somewhat resourceful in specialized contexts.
They can be found in items such as magnetic tools that can pick up screws for easy management in construction or assembly tasks. While their magnetism is not permanent, they play a crucial role when temporary magnetism is needed, especially in scenarios where a non-magnetic state is equally essential.
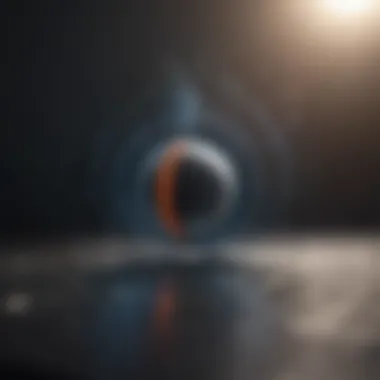
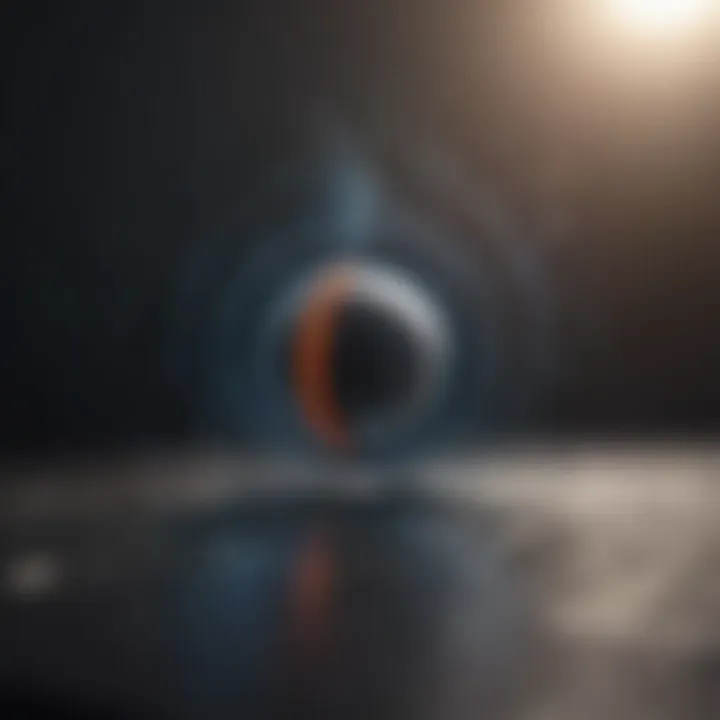
Characteristics of Temporary Magnets:
- Versatility: Their ability to be magnetized and demagnetized allows for adaptive use.
- Efficiency: They can be more effective in situations where a non-magnetic state is required.
- Cost-effectiveness: Often, they can be produced with less expensive materials compared to permanent magnets.
Electromagnets
Electromagnets are truly a marvel of engineering that illustrates the relationship between electricity and magnetism. These magnets are created by running electric current through a coil of wire, usually wrapped around a ferromagnetic core. The resulting magnetic field can be controlled by adjusting the strength of the current, which makes electromagnets incredibly versatile.
Their uses span across various sectors, from industrial machinery to medical technology. For example, in MRI machines, electromagnets help create strong magnetic fields, vital for imaging techniques. Additionally, they are also used in electric motors, magnetic cranes for lifting heavy metal objects, and even in telecommunications components. This makes them crucial not only in theoretical physics but also in practical applications.
Notable Aspects of Electromagnets:
- Controllable Strength: The magnetic field's intensity can be varied by changing the electric current.
- Temporary Nature: They only exhibit magnetism when powered, which can be beneficial in preventing interference when not in use.
- Complexity: Their design requires more intricate engineering, but this complexity offers an array of functional advantages.
Understanding these three types of magnets provides a clearer perspective on how magnetic technology has evolved and is applied in various fields today. Recognizing their unique characteristics and applications ensures that both educators and students can engage with this topic in a meaningful way.
Properties of Magnets
Understanding the properties of magnets is crucial in grasping how they interact with their surroundings, which is particularly relevant in both academic and practical contexts. These fundamental characteristics not only inform a studentโs knowledge but also influence innovations in technology and engineering. Comprehending how magnets behave aids in diverse applications, from basic schooling experiments to advanced medical imaging techniques.
Magnetic Poles
Every magnet, regardless of its size or shape, possesses two magnetic poles: the north pole and the south pole. This feature is not just a technicality. The fundamental concept here is that opposite poles attract while like poles repel. For instance, if you have a bar magnet and you try to bring its north pole close to another north pole, youโll feel the push away from each other. However, bring the north pole towards a south pole, and they clasp together like long-lost friends reuniting.
The existence of these poles explains countless phenomena. For example, if you break a magnet into two pieces, each piece will become a smaller magnet complete with its own north and south pole. This property illustrates the principle of conservation; magnets never truly lose their poles. Additionally, experiments with compasses demonstrate how Earthโs magnetic field exhibits this pole behavior. A compass needle aligns itself with the Earthโs magnetic field, pointing toward the magnetic north. This interaction is more than academic; it guides travelers and explorers.
Magnetic Field Strength
The strength of a magnetic field is also a pivotal property determining how effectively a magnet can exert force over a distance. Measured in teslas (T), the strength influences many applications, such as electric motors or magnetic storage devices. A stronger magnetic field can interact with more objects, and knowing how to manipulate that strength is key in technological applications.
To visualize this, consider the magnetic field strength of a typical refrigerator magnet versus that of an industrial electromagnet. The refrigerator magnet can hold light items on a ferromagnetic surface but would fail to hold anything heavier or farther away. On the other hand, electromagnets can generate significantly more power and can be turned on or off by controlling the electric current. This flexibility shows how engineers can utilize magnetic field strength in a variety of settings, tailoring solutions based on specific needs.
Magnetic Flux
Magnetic flux, which involves both the strength of the magnetic field and the area through which it passes, is essential in understanding electromagnetic concepts. It can be thought of as a measure of the total magnetic field that penetrates a given area, and it is often discussed in the context of Faradayโs laws of electromagnetic induction.
To break this down, calculating magnetic flux involves the formula:
[ \Phi = B \cdot A \cdot \cos(\theta) ]\
Where:
- ( \Phi ) = magnetic flux in webers
- ( B ) = magnetic field strength
- ( A ) = area
- ( \theta ) = angle between the magnetic field and the normal to the surface
This property not only plays a role in generators and transformers but also helps explain phenomena in electric circuits. Higher magnetic flux can induce larger currents, which is why understanding this property is vital for students, educators, and those delving into the realms of electrical engineering or physics. With this knowledge, professionals can innovate and optimize designs that rely on magnetism, showcasing the endless possibilities within this field.
Understanding Magnetic Fields
Understanding magnetic fields is crucial not just in the realm of physics, but also in everyday life. These invisible forces play a significant role in both natural phenomena and practical applications. By harnessing the knowledge of magnetic fields, we can unlock advancements in technology, medicine, and even environmental science. This section focuses on how magnetic fields are generated and visualized, each aspect serving distinct yet interconnected purposes.
Generating Magnetic Fields
Magnetic fields stem from various sources, each with unique characteristics and applications. The two main types of magnetsโpermanent and electromagnetsโgenerate magnetic fields through different mechanisms. Permanent magnets have a constant magnetic field due to their materialโs internal structure, a feature often likened to the arrangement of soldiers in formationโeach atom in a magnet maintaining its position and orientation consistent with the others.
On the other hand, electromagnets create a magnetic field through electric current flowing in a coil of wire. This principle can be understood using the right-hand rule. By curling the fingers of your right hand in the direction of current flow and pointing your thumb, you can visualize the direction of the magnetic field created. This ability to turn off and on makes electromagnets incredibly useful in devices like motors and magnetic locks.
Here are a few key points about generating magnetic fields:
- Permanent Magnets: Made from materials like neodymium and samarium-cobalt, they are reliable but less flexible in application.
- Electromagnets: These allow for control over the magnetic field, making them ideal for tasks requiring variable strength.
Understanding how to generate magnetic fields enhances our ability to innovate technologies ranging from magnetic resonance imaging (MRI) machines in hospitals to maglev trains that float above tracks.
Visualizing Magnetic Fields
It's one thing to know that magnetic fields exist; itโs another to actually visualize them. While magnetic fields are invisible to the naked eye, there are several effective methods to visualize these forces. One common approach is using iron filings. When sprinkled around a magnet, these filings align themselves along the lines of the magnetic field, creating an awe-inspiring pattern that reveals the otherwise hidden geometry of these forces.
Another sophisticated method includes using magnetic field sensors coupled with graphic displays, which render real-time visualizations. An example can be seen in scientific research labs where physicists map out magnetic fields, providing invaluable insights into both natural and engineered systems.
Additionally, computer simulations can depict magnetic fields in three dimensions, creating interactive models that enhance understanding. This multifaceted approach is beneficial for a range of audiences, from students learning concepts in the classroom to researchers working on cutting-edge technology.
In summary, visualizing magnetic fields not only deepens our understanding of their nature but also aids in education and innovation, enabling clearer communication of complex scientific ideas.
"A good visualization is worth a thousand words; it transforms abstract concepts into something tangible that everyone can grasp."
The significance of understanding how to generate and visualize magnetic fields extends beyond academic curiosity. It opens doors to practical applications that spur progress across many sectors, underscoring the importance of magnetic studies in both history and future developments.
Interaction Between Magnets and Magnetic Fields
Understanding the interaction between magnets and magnetic fields is crucial in grasping the fundamental principles of magnetism. Magnets do not exist in isolation; their influence extends into the space around them, affecting other magnets and magnetic materials. Knowing about this interaction opens doors to various applications ranging from everyday tools to sophisticated technologies. By delving into these interactions, we can appreciate not only the strength and dynamics of magnetic forces but also their implications in both natural phenomena and engineered systems.
Forces Between Magnets
The forces between magnets can be simple yet deeply fascinating. When two magnets come close, they either attract or repel each other. This pull or push is a direct manifestation of the magnetic fields they generate. Each magnet has two poles: north and south. If you put together opposite poles, like North of one magnet with South of another, they will pull each other together. On the flip side, if you try to push two north poles together, they'll send each other flying apart, as if theyโve just seen a ghost.
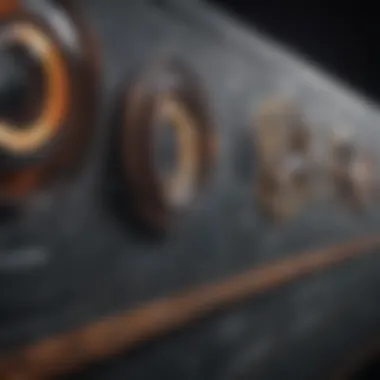
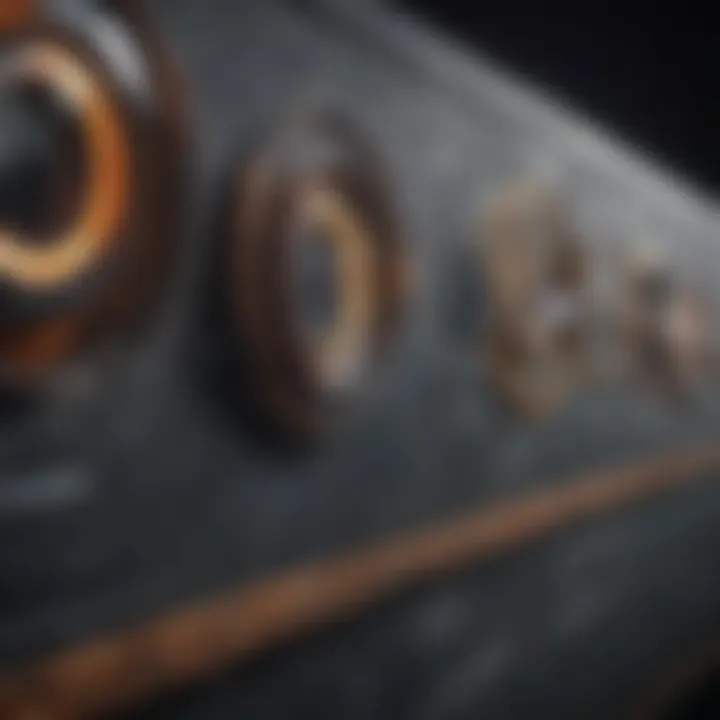
The strength of this force depends on several factors:
- Distance: The closer the magnets are to each other, the stronger the interaction. For instance, if youโve ever played with fridge magnets, youโd know that if they are too far apart, they donโt stick at all.
- Strength of Magnets: The intensity of the magnetic field a magnet possesses contributes to the force it exerts. A stronger magnet, like a neodymium magnet, can produce a much more powerful force compared to a weaker one made from ceramic materials.
- Orientation: How the magnets are oriented can play a vital role. Aligning the poles correctly enhances interaction.
Understanding these forces helps in designing systems where magnetic interaction is vital, such as in magnetic levitation trains, where strong magnets repel each other to float above the tracks, significantly reducing friction.
Magnetic Field Lines
Visualizing magnetic fields through magnetic field lines provides an intuitive grasp of magnetism. These lines are a way to illustrate the direction and strength of the magnetic fields surrounding a magnet. Each line emanates from the north pole of a magnet and loops back to the south pole. The density of these lines in a particular area indicates the strength of the magnetic field; more lines packed together means a stronger magnetic influence.
Some key characteristics of magnetic field lines include:
- Directionality: The lines always flow from north to south outside the magnet, and from south to north inside the magnet.
- Non-intersecting Nature: Magnetic field lines never cross each other; if they did, it would mean that there are two different directions for the magnetic field at one point, which is impossible.
- Curvature: The lines curve and spread out, demonstrating how the magnetic force fades with increasing distance from the magnet.
"Magnetic field lines help visualize how magnets interact. They map the invisible but immensely powerful influence of magnets in our world."
This understanding of magnetic field lines is essential in a range of applications, including electric generators, transformers, and magnetic resonance imaging. By visualizing and analyzing these lines, scientists and engineers can predict how magnets will behave in various situations, tailoring their designs for optimal performance.
In summary, the interaction between magnets and magnetic fields is a cornerstone in understanding magnetism. From the forces that govern their behavior to the visualization provided by magnetic field lines, these concepts not only deepen our knowledge but also propel advancements across various fields."
Applications of Magnets
Magnets play a pivotal role in modern technology, extending their influence into various sectors that significantly enrich our daily lives. Understanding applications of magnets isn't merely an exploration of physical principles; it touches on the transformative advancements within numerous fields. With their capability to produce magnetic fields, magnets are utilized in everything from data storage to medical innovations and industrial machinery. The importance of magnets extends beyond mere functionality; they serve as cornerstones for technologies that push boundaries and foster progress.
Magnetic Storage Devices
In the realm of information technology, magnents are the unsung heroes behind the functionality of magnetic storage devices. Devices like hard disk drives use magnetic fields to store and retrieve vast amounts of data. The basic principle revolves around the orientation of tiny magnetic bits that represent digital information. When you save a file, a write head alters the magnetic state of these bits, allowing your computer to โreadโ the information later.
The significance of magnetic storage is often taken for granted. It enables us to efficiently save photos, documents, and videos. This storage method also provides robust longevity, making hard drives a solid choice for archiving. Nonetheless, solid-state drives (SSDs) have gained traction, often overshadowing traditional magnetic storage. Yet, the latter continues to be vital due to its affordability and vast storage capacity.
"The continued evolution of magnetic storage technology keeps them relevant, particularly for enterprise applications where large data volumes are paramount."
Medical Imaging Techniques
In health care, magnets are indispensable. Techniques such as Magnetic Resonance Imaging (MRI) utilize powerful magnets to generate detailed images of internal body structures. The process works by aligning hydrogen atoms in the body with the magnetic field and then disrupting this alignment with radio frequency pulses, creating signals that are converted into images. This non-invasive imaging method provides critical insights without the need for surgical procedures.
The importance of MRI goes beyond just diagnostics. It aids in monitoring the effectiveness of treatments, assisting in research, and fostering advancements in various medical fields. This application exemplifies how magnetic technology contributes immensely to healthcare, enhancing patient care and enabling breakthroughs in medical research.
Industrial Applications
In industry, magnets find a myriad of uses, from automation to material handling. They play a crucial role in electric motors, generators, and even magnetic separators that filter materials in production processes. Industrial applications can range from lifting heavy objects using magnetic cranes to sorting metals in recycling facilities.
Moreover, they significantly enhance the efficiency of manufacturing processes. Using electromagnets, for instance, allows the creation of adjustable magnetic fields, which can be fine-tuned for specific requirements, providing solutions that are both effective and energy-efficient. Not to mention, their ability to provide safety mechanisms in various machines leads to improved operational efficiency.
In summary, the exploration of magnets and their applications unveils layers of interconnected technologies that have become integral to our lives. From storing our cherished memories to revolutionizing healthcare and powering industries, the utilization of magnets continues to shape our future.
Magnetic Fields in Nature
Understanding magnetic fields in nature allows us to appreciate the intricate connections between natural phenomena and the principles of magnetism. These magnetic fields play vital roles in various aspects of the world around us, influencing everything from navigation to biological processes. By exploring these fields, we gain insights into how they function, their significance, and their multifaceted implications for both science and daily life.
Earth's Magnetic Field
The Earth's magnetic field is perhaps one of the most crucial examples of natural magnetism. Generated by the movement of molten iron in the Earth's outer core, this magnetic field extends thousands of kilometers into space and is essential for life on Earth. It acts as a shield, protecting us from harmful solar and cosmic radiation. Moreover, the magnetic field helps to maintain the atmosphere by preventing solar wind from stripping it away.
The magnetic field is described by magnetic lines of force that emerge from the Earth's magnetic poles. Interestingly, these poles are not fixed; they wander due to changes in the Earth's core dynamics. This wandering can sometimes confuse navigational systems like compasses, which rely on the consistent alignment with Earth's magnetic north. For instance, the North Magnetic Pole has been moving at an alarming rate towards Russia over the last few decadesโit's a clear reminder that even the most stable systems in nature are in constant flux.
"The Earthโs magnetic field not only helps birds navigate during migrations but also regulates several atmospheric phenomena, indicating the integrated nature of magnetism in our ecosystem."
Magnetism in Animals
Remarkably, many animals harness Earthโs magnetic fields to navigate their environments. The phenomenon, known as magnetoreception, has been observed in various species, including birds, turtles, and even some insects. For example, the European robin uses the Earth's magnetic field to find its migratory path each spring and fall. Researchers have identified specialized proteins called cryptochromes in birds' eyes, which might enable them to sense the magnetic field visually.
Certain marine animals, like sea turtles, also exhibit this fascinating ability. After hatching, they instinctively navigate vast oceanic distances back to their natal beaches, likely using the Earthโs magnetic field as a guide.
Additionally, studies on the blacktip reef shark suggest that these creatures possess receptors in their snouts that allow them to detect variations in the magnetic field, aiding their hunting and migration patterns.
Understanding these magnetic adaptations in animals highlights the interplay between biology and physics, revealing nature's clever solutions to survival.
Through these examples, we see that magnetic fields are not just abstract concepts confined to physics laboratories. They are woven into the fabric of nature, influencing behavior and navigation in ways we are only beginning to understand.
Recent Advances in Magnetic Research
Magnetic research has become a focal point in physics and material science, unlocking new avenues for both theoretical inquiry and practical applications. As technology progresses, understanding the latest developments can lay a foundation for innovations that could significantly impact various sectors, from electronics to healthcare. The interest in magnetism goes beyond just curiosity; itโs about harnessing the properties of magnets to improve the devices and systems we use daily. In this section, we will explore some novel magnetic materials and the intriguing field of quantum magnetism.
Novel Magnetic Materials
Novel magnetic materials are the result of combining science with a bit of creative engineering. These materials exhibit unique magnetic properties that can be tailored for specific applications. For example, researchers have developed materials like magnetocaloric materials that change temperature in response to a changing magnetic field. This could pave the way for new cooling systems that are more efficient than traditional methods.
Some recent advancements include:
- Artificial Spin Ice: This is a type of magnetic material that mimics the properties of natural ice but at a smaller scale. Itโs useful for studying magnetic interactions and could lead to better data storage techniques.
- High-Temperature Superconductors: These materials can conduct electricity without resistance at temperatures previously thought impossible. Their magnetic properties also hold promise for energy applications.
- Topological Insulators: They possess unique surface states that can conduct electricity while insulating in the bulk. This characteristic is tied to their magnetic behavior and is crucial in developing next-gen electronic devices.
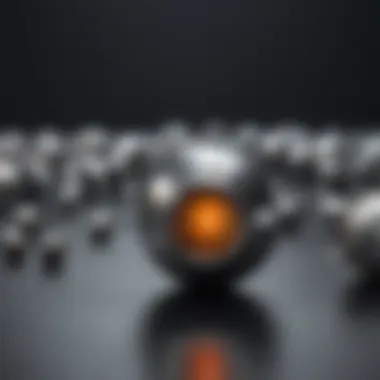
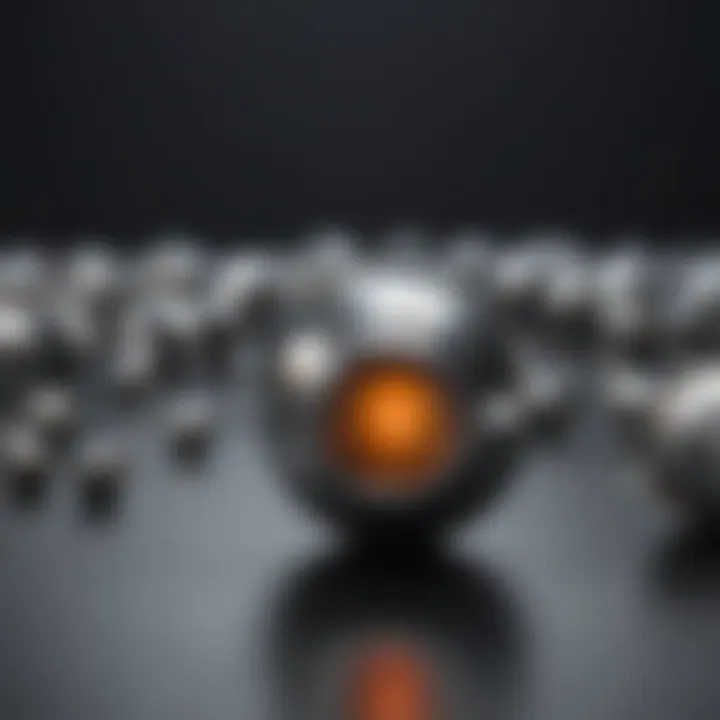
The impact of these materials canโt be understated; they are reshaping industries and inspiring new research. Their potential use in green technologies offers a glimpse of what future innovations could look like, providing sustainable and more efficient alternatives to conventional materials.
Quantum Magnetism
Quantum magnetism unravels the complexities of magnetism at microscopic levels, where quantum mechanics reigns supreme. The interaction of magnetism and quantum physics brings to light phenomena that defy classical understanding. For instance, the behavior of electrons in a magnetic field showcases quantum entanglement, leading to some baffling yet fascinating conclusions about how particles can influence each other across distances.
This domain of study has given rise to:
- Quantum Dots: These tiny particles can control electronic states and exhibit unique magnetic properties, making them suitable for applications such as quantum computing. Researchers aim to leverage these innovative features to create more robust quantum bits (qubits).
- Frustrated Magnetism: Here, the arrangement of magnetic moments can lead to unexpected behaviors, such as the formation of spin liquids, which have implications for understanding materials with novel magnetic properties.
- Spintronics: This field exploits the intrinsic spin of electrons for information processing. The integration of quantum principles into spintronic devices could revolutionize data storage and processing efficiency.
"Quantum magnetism opens a door to a new realm of technological possibilities. Understanding these principles is essential for engineers and physicists alike."
As research in quantum magnetism unfolds, it offers a better grasp of not just traditional magnetic materials but also the broader implications of how these particles interact. This knowledge will likely lead to breakthroughs we can hardly predict today, cementing magnetismโs role in the future of technology and science.
By exploring these advances, we become aware of how fundamental research can have ripple effects across various fields, underlining the idea that magnetism is not merely a subject learned in classrooms but a vibrant area that drives modern-day innovations.
Future Directions in Magnetism
The field of magnetism is not static; it continually evolves, pushing the boundaries of what we know and expanding into new territories. As we stand at this crossroads of innovation, understanding the future directions in magnetism becomes critical. The advancements in this field will influence not just scientific research but can also have far-reaching impacts across various sectors, from technology to medicine and beyond.
Emerging Technologies
Emerging technologies in magnetism are paving the way for remarkable breakthroughs. One prime example is the advancement of magnetic materials. Recent developments focus on materials that are not only stronger but also more adaptable to different environments. These materials can potentially replace or enhance existing technologies. For instance, innovations in permanent magnet technologies can lead to lighter and more efficient electric motors, thus fostering improvements in electric vehicles.
Moreover, the realm of spintronics, which exploits the intrinsic spin of electrons in addition to their charge, opens up the doors for faster and more energy-efficient data storage and processing techniques. Think about the potential of having computers that bound infinely faster due to these spin-based actions.
- Quantum Computing: Another area of interest is how magnets may influence quantum computing. Quantum bits, or qubits, can be influenced by surrounding magnetic fields, enhancing coherence time and overall performance.
- Magnetic Sensors: There's also increasing focus on developing magnetic sensors that can detect subtle changes in the environment, which can be crucial for applications in various industries such as healthcare and environmental monitoring.
Theoretical Developments
On the theoretical front, researchers are delving deeper into magnetic phenomena, from exploring quantum magnetism to unraveling the complexities behind magnetic domains. Theoretical developments can provide insight into how magnets interact at a nano level, which can further refine the design of materials used in high-tech applications. Understanding these interactions can lead to better magnetic imaging techniques and improved technologies in telecommunications.
Theories are also maturing around concepts such as topological insulators, which exhibit unique magnetic properties and offer pathways to new applications in electronics.
"Theoretical advancements can often serve as the backbone for future practical applications, establishing a bridge between what seems theoretically possible and what is feasible in the real world."
Lastly, interdisciplinary collaborations are starting to bear fruit. When physicists, materials scientists, and engineers pool their knowledge, these synergies can lead to unprecedented insights. Such teamwork is crucial, particularly in tackling complex problems and translating theories into tangible technologies.
In summary, the future directions in magnetism hint at a landscape rich with opportunity and discovery. As these emerging technologies and theoretical elements come into play, we can expect a significant reshaping not just of magnetic materials, but of a broad spectrum of industries and scientific disciplines.
Educational Significance of Magnetism
Magnetism plays a multifaceted role in education, acting as both a bridge to understanding fundamental physics concepts and a means of engaging students with hands-on experiments. In todayโs tech-driven world, the significance of magnetism is undeniable, offering insights not only into science but also into fields such as engineering and medicine. By grounding students in the principles of magnetism, educators prepare them for a future where this knowledge will be pivotal for innovation.
The ability to demonstrate magnetic principles through practical applications is among the most compelling reasons to teach magnetism in schools. When students can see and feel magnetic forces in action, their grasp of the material deepens, often leading to a heightened curiosity about the physical world. This experiential learning fosters critical thinking and problem-solving skills, vital components for academic and professional success.
Moreover, incorporating magnetism into the curriculum allows educators to create interdisciplinary links. For instance, addressing how magnets work can smoothly transition into discussions about electricity, materials science, and even environmental studies.
Another key aspect to consider is the growing array of technologies that rely on magnetic principles. From data storage solutions like hard drives to medical imaging devices such as MRIs, the applications of magnetism span numerous sectors. Consequently, grounding students in these principles cultivates not only knowledge but also awareness of career pathways that they may not have previously considered.
"Understanding magnetism is not just an academic exercise; it's a gateway to discovering the interconnectedness of scientific disciplines and innovations that shape our world."
Ultimately, the educational significance of magnetism lies in its capacity to engage, inspire and prepare future generations for complex problems yet to come. As educators, prioritizing this vital topic in the classrooom will unlock the potentials within students to navigate both current and future scientific landscapes.
Teaching Magnetism in Schools
When teaching magnetism, a hands-on approach can lead to enhanced student engagement and understanding. Teachers can organize activities using simple materials like bar magnets, iron filings, and compasses to illustrate the various properties and principles of magnets.
Hereโs a brief look at some effective techniques:
- Experiments: Simple experiments, like demonstrating how iron filings align with a magnetic field when sprinkled over a paper, can vividly illustrate magnetic field lines.
- Interactive Lessons: Using electronic platforms or models that show magnetic forces in action can complement traditional methods.
- Field Trips: Visits to science museums or industrial sites that utilize magnetism can help contextualize the subject matter.
In addition to practical activities, integrating discussions about historical figures in magnetism, such as Oersted and Faraday, can round out the learning experience, connecting present ideas with past discoveries.
Resources for Educators
Here are some valuable resources that can assist educators in effectively teaching magnetism:
- Wikipedia - Magnetism: A solid starting point for fundamental concepts and historical context.
- Britannica - Magnetism: Offers in-depth articles and insights related to magnetism and its applications.
- Reddit - r/Physics: An engaging community where educators can share resources and best practices in teaching complex topics, like magnetism.
- DIY Kits: Numerous educational companies offer magnetism experiment kits that provide hands-on learning.
- Educational Videos: Platforms like YouTube have a variety of educational channels focusing on physics experiments involving magnets.
Equipping educators with these tools not only enhances their teaching practice but also enriches the learning experience for students, ultimately instilling a profound appreciation for the dynamics of magnetism.
Epilogue
In wrapping up our exploration of magnets and magnetic fields, itโs vital to recognize their profound significance in both science and daily life. From the early encounters with naturally occurring magnets to the complexities of contemporary electromagnetic applications, this topic captures the essence of fundamental physics. Understanding these concepts is not just academic; it offers a window into the workings of our world.
Recap of Key Concepts
Weโve walked through various pivotal elements that describe magnets and their associated fields. Here is a sum-up of the key aspects:
- Definitions: Magnets generate a magnetic field that affects other magnetic materials and electric currents.
- Types of Magnets: There are permanent, temporary, and electromagnets, each serving distinct purposes in technology.
- Properties: The intrinsic characteristics, like polarity and strength, are crucial for their functionality.
- Interactivity: The forces between magnets and their fields dictate how these energy patterns interlace with each other.
- Applications: The relevance of magnets extends into storage, medical imaging, and even natural occurrences in Earthโs structure.
By revisiting the foundations, weโve solidified an understanding that will serve students, teachers, and professionals alike. Magnetic phenomena govern not merely gadgets but also essential natural processes.
Implications for Future Study
Looking ahead, the domain of magnetism is ripe for further inquiry. Consider the following directions:
- Emerging Technologies: As digital landscapes evolve, magnets will play a vital role in powering next-gen electronics, possibly leading to breakthroughs in quantum computing.
- Sustainable Solutions: Research into new magnetic materials could pave the way for greener technologies.
- Cross-Disciplinary Applications: The intersection of magnetism with biology and materials science is an area awaiting exploration; understanding how magnetism affects living organisms might unveil untold applications in medicine.