Understanding Magnetism: Principles and Applications
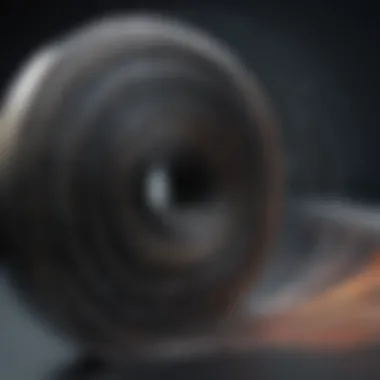
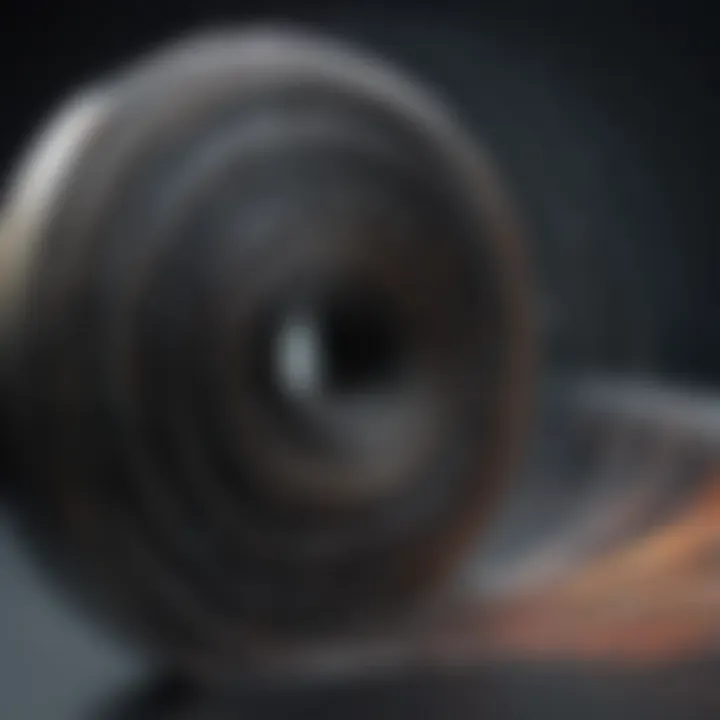
Intro
Magnetism plays a crucial role in the fabric of physics. Not limited to mere attraction and repulsion, it is part of a broader spectrum of electromagnetic phenomena. Understanding magnetism involves examining both its historical developments and fundamental principles. This exploration reveals its significance in various applications ranging from everyday technology to advanced scientific research.
Key Research Findings
Overview of Recent Discoveries
Recent advancements in the field of magnetism have revealed several intriguing discoveries. Researchers have observed the behavior of magnetic materials at the atomic level. These insights have led to a better understanding of how magnetism interacts with other physical properties. For instance, the phenomenon of spintronics utilizes electron spin in addition to charge, opening new avenues for more efficient data storage and processing.
Significance of Findings in the Field
The implications of these discoveries extend beyond mere theoretical knowledge. They are pivotal in fields such as materials science and quantum computing. Enhanced understanding of magnetism aids in the design of more effective magnetic materials. Moreover, these insights serve as the foundation for innovations in technology, potentially impacting future designs of electronic devices.
Breakdown of Complex Concepts
Simplification of Advanced Theories
To fully grasp the complexities of magnetism, it is essential to break down advanced theories into digestible parts. Concepts such as the Lorentz force, magnetic domains, and the hysteresis loop exhibit the multifaceted nature of magnetic phenomena. By simplifying these ideas, we can better appreciate their real-world implications and the underlying physics that governs magnetic behavior.
Visual Aids and Infographics
Using visual aids can further enhance our understanding. Infographics illustrating magnetic field lines or the arrangement of magnetic domains can provide clarity. These tools enable students and enthusiasts to visualize abstract concepts, reinforcing comprehension. For example:
- Magnetic Field Lines: These lines illustrate the strength and direction of a magnetic field. They always form closed loops, showcasing the continuity of magnetic forces.
- Magnetic Domains: Each domain acts like a tiny magnet. Their alignment determines whether a material is magnetic.
"Magnetism is not just a subject of study; it is integral to many domains of modern life."
The exploration of these concepts lays the groundwork for a more nuanced understanding of magnetism, bridging the gap between textbook knowledge and practical application.
Prelims to Magnetism
Magnetism holds a critical place within the landscape of physics. It is not just a force observed in magnets but a fundamental principle that governs various natural phenomena and technological applications. The study of magnetism can illuminate the behavior of materials, influence electrical systems, and enhance our understanding of the universe.
The domain of magnetism intertwines with concepts of electromagnetic forces, allowing us to comprehend how charged particles interact. Recognizing these connections is vital when exploring topics like electromagnetic induction, magnetic fields, and even quantum physics. The implications are vast, affecting everything from everyday appliances to advanced medical technologies.
Definition and Overview
Magnetism can be defined as a physical phenomenon produced by the motion of electric charge, which results in attractive and repulsive forces between objects. This force arises from microscopic attributes of materials, stemming from the alignment of atomic magnetic moments. Such moments are generated by electron spin and orbital angular momentum.
In essence, magnetism manifests in various forms, most notably through ferromagnetism, where materials like iron exhibit strong magnetic properties. Less intense forms such as paramagnetism and diamagnetism exist but are often overshadowed by their ferromagnetic counterparts. Understanding these distinctions is crucial for anyone delving into the subject.
Magnetism plays a pivotal role in our daily lives. It drives much of modern technology, from data storage devices to medical imaging. Thus, a solid grasp of its definition and underlying principles lays the groundwork for deeper exploration into its practical applications.
Historical Perspectives
The journey of magnetic exploration dates back thousands of years. The ancient Greeks discovered lodestone, a naturally magnetized mineral, while the Chinese used compasses by the 11th century. However, it was not until the 19th century that significant advancements occurred, largely attributed to scientific giants like Hans Christian Γrsted and James Clerk Maxwell.
In 1820, Γrsted observed that a charged wire could influence a compass needle, establishing a fundamental link between electricity and magnetism. Maxwell later synthesized these ideas into his equations, showcasing how electric and magnetic fields interrelate. This laid the groundwork for modern electrodynamics.
"The study of magnetism has evolved from mysticism to a pivotal field in physics that links various domains of science together."
As research progressed, the scope of magnetism expanded beyond classical physics into contemporary realms like quantum mechanics. Understanding the historical context enriches our appreciation of magnetism's relevance today. Each discovery is a stepping stone towards harnessing these phenomena for future innovations in technology and materials science.
Fundamental Principles of Magnetism
Understanding the fundamental principles of magnetism is essential for grasping how this physical phenomenon operates in various contexts. Magnetism is interconnected with electricity and plays a key role in numerous applications, from everyday devices such as motors and generators to advanced technologies like MRI machines and quantum computing. The importance of this section lies in elucidating core concepts that form the basis of magnetic theories and applications. By exploring the essentials, readers can appreciate how magnetism influences both scientific inquiry and technological advancements.
Magnetic Fields and Forces
Magnetic Field Lines
Magnetic field lines serve as a conceptual tool to visualize the magnetic field's nature and behavior. These lines provide insight into the magnetic force's direction and strength at various points in the field. A primary characteristic of magnetic field lines is that they always form closed loops, emerging from the north pole of a magnet and entering through its south pole. This property is a beneficial choice for this article because it effectively illustrates the physical reality of magnetic fields. A unique feature of magnetic field lines is their density; closer lines indicate a stronger magnetic field. This characteristic allows for ease of understanding when analyzing magnetic phenomena, helping students and enthusiasts conceptualize complex interactions among magnetic forces and materials.
Field Strength and Direction
Field strength and direction are critical aspects in the study of magnetism. They determine the degree of magnetic influence on charged particles and nearby magnets. A key aspect of field strength is the gradient of force; as one moves away from the magnet, the strength of the magnetic field decreases rapidly. This behavior is noteworthy because it highlights how field interactions change with distance. The direction of the field aligns with the flow of magnetic force, indicating that it points from the north to south pole of a magnet. This dual focus on strength and direction makes it a popular topic for further exploration within this article. Understanding field strength and direction aids in practical applications, such as creating more efficient electric motors or optimizing magnetic storage devices.
Magnetic Poles and Dipoles
North and South Poles
The concept of north and south poles is fundamental in magnetism. Every magnet has a distinct north and south pole, with each exerting forces on other magnets and materials. A critical feature of north and south poles is their inherent property of attraction and repulsion; like poles repel and opposite poles attract. This binerity is a beneficial property in understanding magnetic poles, as it sheds light on the basic interactions between different types of magnets. The unique aspect of north and south poles in this context is their role in forming dipoles, which are essential for studying magnetic fields in detail. This simplicity underpins various magnetic applications, ranging from compasses to advanced electromagnets.
Behavior of Magnets
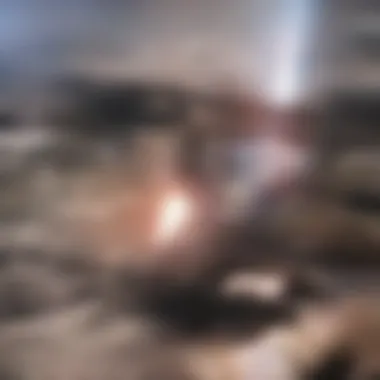
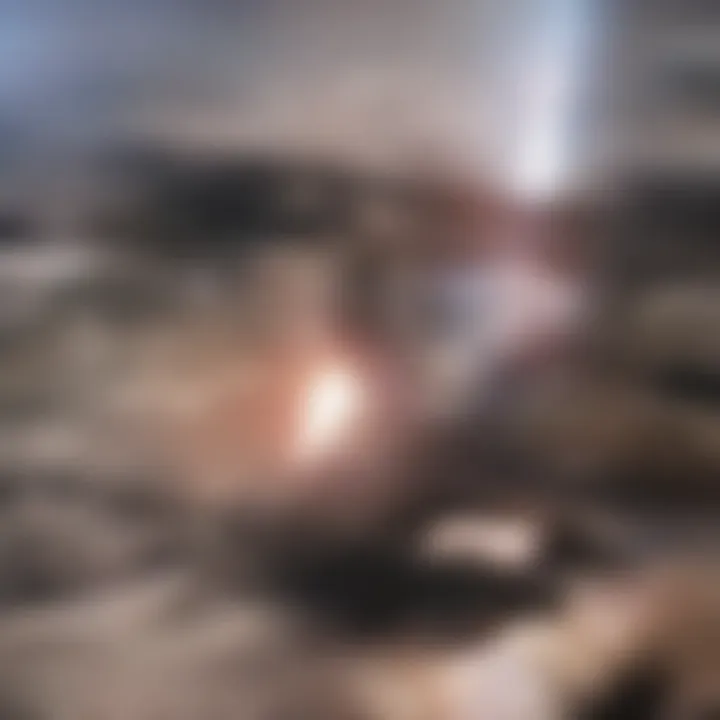
The behavior of magnets encompasses how they interact with one another and with magnetic fields. Magnets respond predictably to opposing forces, demonstrating attraction or repulsion based on their poles. One key characteristic of magnet behavior is that all magnets experience a magnetic torque when placed in a magnetic field, which can rotate them to align with the field. This understanding is a beneficial aspect, providing a basis for practical designs of magnetic devices. A unique feature of studying magnet behavior is the concept of magnetic domains in ferromagnetic materials; these small regions within a material can align to produce a significant magnetic effect. This intrinsic property is advantageous for enhancing the effectiveness of magnetic materials in technology and industry.
"Magnetic poles follow the fundamental rule of attraction and repulsion, essential for practical applications in our daily lives."
Understanding the fundamental principles of magnetism equips readers with foundational knowledge necessary for examining more complex magnetic interactions and innovations. These principles pave the way for exploring technological advancements and their implications in various fields.
Types of Magnetism
Understanding types of magnetism is vital in grasping the broader framework of magnetic phenomena. Each type represents distinct behaviors exhibited by materials under magnetic fields. This article focuses on ferromagnetism, paramagnetism, and diamagnetism. These categories are not just academic; they have practical implications in various fields such as technology, medicine, and materials science.
Ferromagnetism
Characteristics and Examples
Ferromagnetism is a prominent form of magnetism. Materials like iron, nickel, and cobalt exhibit this property. A key characteristic is the alignment of magnetic moments in the same direction, resulting in a strong, permanent magnet. Unlike other materials that may only exhibit magnetism in the presence of an external field, ferromagnetic materials can retain their magnetic state after the external field is removed.
Some good examples include common items such as refrigerator magnets and magnetic tools. Their widespread use showcases ferromagnetism's practical benefits. However, one must consider that ferromagnetic materials can become demagnetized under high temperatures, known as the Curie temperature.
Applications in Industry
The applications of ferromagnetism in industry are vast. This type of magnetism is foundational in designing electrical transformers and inductors. Because of its ability to sustain magnetization, it is a fundamental choice for power generation. The unique feature of ferromagnetic materials is their capacity for saturation, which means they can carry a maximum magnetic field strength before becoming ineffective. While advantageous for efficiency, a possible disadvantage is overheating that can lead to loss of magnetic properties.
Paramagnetism and Diamagnetism
Difference in Behavior
Paramagnetism and diamagnetism represent two contrasting behaviors under magnetic influences. Paramagnetic materials, such as aluminum and certain metal oxides, have unpaired electrons that align with an external magnetic field, creating weak attraction. In contrast, diamagnetic materials, including copper and bismuth, develop a weak repulsion when exposed to a magnetic field due to a decrease in the electron's motion.
Understanding the differences in behavior is crucial for their applications. While paramagnetic materials can enhance the strength of a magnetic field, diamagnetic materials are often used to shield sensitive instruments from external magnetic interference.
Real-world Examples
Real-world examples further elucidate these phenomena. For instance, paramagnetism is utilized in Magnetic Resonance Imaging (MRI), where gadolinium compounds enhance image clarity. Diamagnetism is employed in certain levitation technologies such as superconductor magnets that repel magnetic fields. The unique feature of paramagnetic and diamagnetic materials collectively underscores their importance in practical applications. However, one might note that both types are generally weaker compared to ferromagnetic materials, limiting their usage in some conditions.
"The meticulous study of magnetism opens doors to innovative technologies and applications across various sectors."
Magnetism and Electricity
Magnetism and electricity are two interrelated aspects of physics that form the basis of many modern technologies. This connection is crucial for understanding various phenomena in both scientific and practical contexts. The interplay between these forces leads to significant applications across energy generation, data storage, and various forms of transportation.
Understanding this relationship offers invaluable insights into how technologies operate and evolve, making it a critical focus in this exploration of magnetism. The implications of these principles extend far, influencing daily life in ways often overlooked yet pivotal to technological advancement.
Electromagnetism
Fundamental Concepts
Electromagnetism is the branch of physics that deals with the interaction of electric and magnetic fields. At its core, it describes how electric charges create magnetic fields, and how these fields can influence charges. One significant aspect of electromagnetism is the concept of electromagnetic waves, which includes light, radio waves, and more. This characteristic makes it incredibly versatile.
Electromagnetism is a beneficial choice for this article because it serves as a fundamental concept that binds various topics within magnetism. Understanding these concepts can demystify complex interactions and provide a clearer view of how energy and signals are transmitted.
A unique feature of electromagnetism is its ability to create forces in remote locations through field effects. This allows for innovations in communications and power systems, showcasing its vast advantages in modern technology while also presenting challenges, such as managing interference between devices.
Maxwell's Equations
Maxwell's equations are a set of four equations that describe how electric and magnetic fields interact. These equations are pivotal because they unify electricity, magnetism, and light into a single theoretical framework. The significance of Maxwell's equations lies in their ability to predict how changing electric fields produce magnetic fields and vice versa.
These equations represent a foundational element of electromagnetic theory, making it a popular inclusion in discussions surrounding magnetism. They offer an elegant mathematical representation of electromagnetic phenomena, illustrating both theoretical and practical applications.
The unique feature of Maxwell's equations is their ability to simplify complex relationships into manageable forms that can be applied to a range of problems in electricity and magnetism. However, their abstract nature can sometimes be challenging for those new to the concepts, presenting a potential disadvantage in introductory discussions.
Applications of Electromagnetic Principles
Transformers and Generators
Transformers and generators are invaluable devices that utilize electromagnetic principles for energy transformation and generation. Transformers adjust voltage levels in electrical systems, allowing efficient energy transmission over long distances. Generators, on the other hand, convert mechanical energy into electrical energy using electromagnetic induction.
These devices are beneficial to this article because they exemplify how theoretical principles translate into real-world applications, demonstrating practical aspects of electromagnetism. Their design harnesses the fundamental concepts of magnetic fields and conductors, making them essential for energy management.
A unique aspect of transformers and generators is their efficiency and ability to function across various scales. They are integral to national power grids and small-scale applications alike. However, challenges such as energy losses and maintenance requirements must be considered in their use.
Magnetic Levitation
Magnetic levitation is a fascinating application of magnetism, using magnetic forces to suspend objects without physical contact. This technology is employed in maglev trains and other transport systems. Its importance cannot be understated as it showcases the potential of magnetism in achieving groundbreaking advancements in technology and transportation.
One key characteristic of magnetic levitation is its ability to reduce friction in moving systems, significantly increasing efficiency and speed. This makes it an attractive option for modern transportation solutions.
The unique feature of magnetic levitation lies in its capacity to enable smooth and rapid travel, transforming how we think about transit methods. Although it offers several advantages, such as high efficiency and low wear on moving parts, the infrastructure required for magnetic levitation can be costly, limiting its broader adoption.
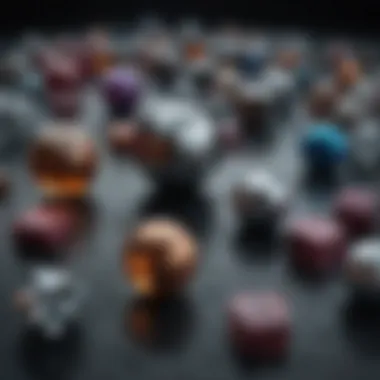
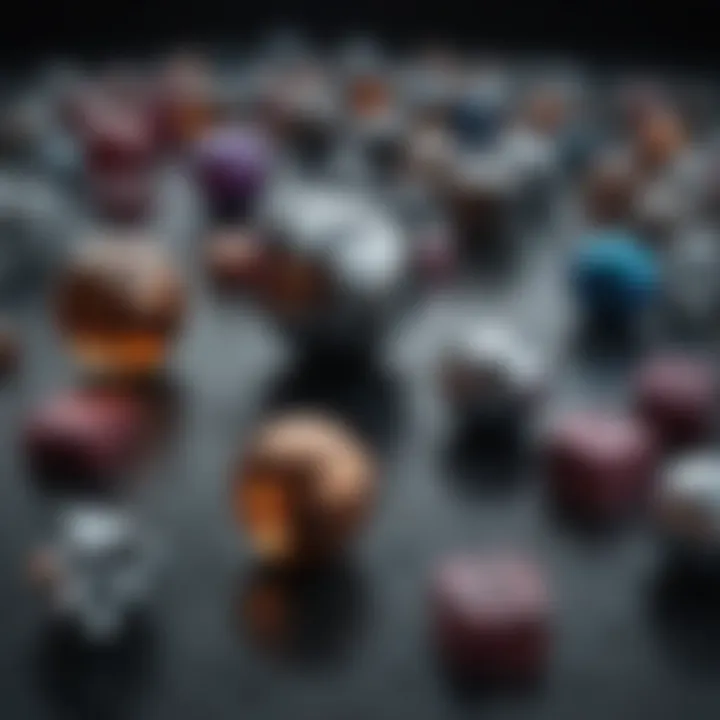
Magnetic Materials
The study of magnetic materials is crucial in understanding the broader field of magnetism in physics. These materials exhibit various magnetic behaviors that are essential for numerous applications in technology and industry. Magnetic materials can be classified based on their intrinsic properties, which include how they respond to external magnetic fields. Understanding these materials fosters insight into their practical uses, as well as their roles in modern technological advancements.
Classification of Magnetic Materials
Hard and Soft Magnetic Materials
Hard magnetic materials are characterized by their ability to retain significant magnetization after an external magnetic field is removed. They are commonly used in permanent magnets found in devices like motors and speakers. The advantage of hard magnetic materials lies in their strong magnetic properties, which make them essential for applications needing long-lasting strength. Examples include ferrites and neodymium magnets. These materials, however, can be more difficult to demagnetize, which may pose problems in certain applications.
In contrast, soft magnetic materials, such as iron and certain alloys, are easily magnetized and demagnetized. Their ability to quickly respond to changing magnetic fields makes them useful in electromagnetic applications. The key characteristic of soft magnetic materials is their lower coercivity, enabling them to switch states with minimal energy loss. This flexibility is advantageous in transformers and inductors. However, they generally cannot maintain a strong magnetic field on their own.
Conductors and Insulators
The distinction between conductors and insulators also plays a significant role in examining magnetic materials. Conductors are materials that facilitate the flow of electric current. Common examples include copper and aluminum. In the context of magnetism, conductive materials can create magnetic fields when electrical current passes through them. This property is vital for applications involving electromagnets, as the efficiency of magnetic fields relies heavily on the conductor's characteristics.
On the other hand, insulators prevent the flow of electric current. Materials like rubber and plastic are classified as insulators. While they do not contribute to conductivity, they are important in providing safety and stability in electronic applications. In magnetic contexts, insulators can help prevent unwanted interference from stray magnetic fields, thus protecting sensitive components in various technologies.
Magnetization Process
The process through which a material becomes magnetized is fundamental to the understanding of magnetism. Various factors influence this process, including temperature and material composition.
Curie Temperature
Curie temperature refers to the specific temperature at which a ferromagnetic material loses its permanent magnetic properties. Above this temperature, thermal agitation prevents alignment of magnetic domains, and the material behaves as a paramagnet. Understanding the Curie temperature is important because it determines operational limits for materials used in applications from sensors to storage devices. The effectiveness of magnetic materials in technology hinges on maintaining they operate below their Curie points.
Magnetic Hysteresis
Magnetic hysteresis describes the lag between the applied magnetic field and the magnetization of the material. This phenomenon is critical in applications involving permanent magnets and magnetic storage. Hysteresis loss can result in energy inefficiencies, highlighting the importance of material selection in device construction. The unique feature of hysteresis is that it demonstrates how a material retains magnetization even after a magnetic field is removed, which can either be advantageous or disadvantageous depending on the specific application.
Recent Advancements in Magnetism Research
The field of magnetism is constantly evolving. With modern technology and increasing research efforts, significant advancements have emerged, driving new applications and enhancing existing theories. These recent advancements play a critical role in various scientific and industrial contexts. They not only redefine our understanding of magnetism but also open pathways for innovations that could reshape technology and healthcare significantly.
Quantum Magnetism
Spintronics
Spintronics represents a novel approach in utilizing both the intrinsic spin of the electron and its fundamental electronic charge for information processing. This area is characterized by its potential to revolutionize the way data is stored and manipulated. Conventional electronics primarily depend on the charge of electrons. However, spintronics introduces a dual functionality that could enhance memory devices.
Spintronic devices have low power consumption and greater data transfer speeds. This makes them an advantageous area in magnetism research. The key characteristic of spintronics is its ability to maintain a magnetic state after the external magnetic field is removed.
This unique feature means data can be retained without continuous power supply, leading to energy efficiency. However, there are challenges in material development and integration within existing infrastructures.
Quantum Computing Applications
Quantum computing represents another frontier in recent advancements, particularly within magnetism research. In essence, quantum computers exploit quantum bits, or qubits, which can exist in multiple states simultaneously, unlike classical bits. This characteristic offers remarkable computational power. A specific aspect of interest is how quantum properties of magnetism can enhance computational capabilities. Quantum algorithms that utilize quantum magnetism can process complex data more efficiently. One key benefit is the potential for breakthroughs in solving previously intractable problems in fields such as cryptography and optimization. Nevertheless, the challenge lies in the implementation of stable qubits and error correction techniques essential for practical applications.
Nanomagnetism
Properties and Fabrication Techniques
Nanomagnetism is a defining area in current research. This focuses on studying magnetic properties at the nanometer scale. The significance of properties and fabrication techniques lies in their ability to create microscopic magnetic materials with tailored characteristics for specific applications. Key techniques include lithography and self-assembly which allow for precise control of magnetization. These advances facilitate the production of smaller, more efficient magnetic components. However, while they promise enhanced performance, the scaling down of materials can introduce unexpected behavior due to quantum effects, making thorough research necessary.
Applications in Medicine and Technology
Nanomagnetism finds its applications not only in technology but also notably in medicine. Magnetic nanoparticles are increasingly used in drug delivery systems, where they can be directed to specific sites in the body using external magnetic fields. This targeted approach holds great promise in improving treatment efficacy and minimizing side effects. Moreover, technologies like magnetic resonance imaging (MRI) benefit from advancements in nanomagnetism. The unique features of these nanoparticles enhance the contrast of images produced, leading to better diagnostic capabilities. However, one must consider safety and biocompatibility when developing these applications, ensuring that they are safe for human use.
"Recent advancements in magnetism research could lead to smarter technologies and novel medical treatments never seen before."
In summary, recent advancements in magnetism research demonstrate how multidisciplinary approaches can enhance understanding and applications within the field. The exploration of quantum magnetism and nanomagnetism presents numerous opportunities for breakthroughs that can impact technology and healthcare positively.
Implications of Magnetism in Modern Technology
Understanding the implications of magnetism in modern technology is crucial, as it shapes numerous aspects of our daily lives and drives advancements in various sectors. The role magnetism plays in storage devices and medical applications highlights its broad significance. These applications not only dictate how we manage data but also influence how we approach health care and diagnostics. By examining these topics, we appreciate the intricate connection between magnetism and technological innovation.
Magnetic Storage Devices
Hard Drives and Magnetic Tapes
Hard drives and magnetic tapes are two primary technologies that utilize magnetic principles for data storage. Their main component is the magnetic material that allows for the recording and retrieval of data. Hard drives work by using magnetic disks to record data, rotating at high speeds, while magnetic tapes use long strips of magnetically coated plastic to store information in a sequential manner.
A key characteristic of hard drives is their speed in accessing data. They offer rapid read and write capabilities, making them popular choices for personal computers and servers. Magnetic tapes, although slower, excel in archiving due to their capacity for holding vast amounts of data very economically.
The unique feature of hard drives is their ability to perform numerous operations per second. This trait is advantageous in environments needing quick data retrieval, such as in business applications. However, they are more susceptible to wear and can be damaged by physical shocks.
In contrast, magnetic tapes provide durability and lower cost per gigabyte, perfect for long-term storage practices. They are not as portable as hard drives, which can be a downside. In this article, both technologies exemplify the critical role of magnetism in data management.
Future of Data Storage
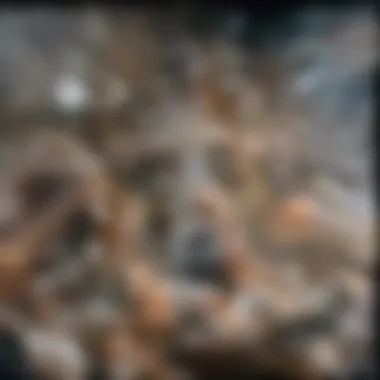
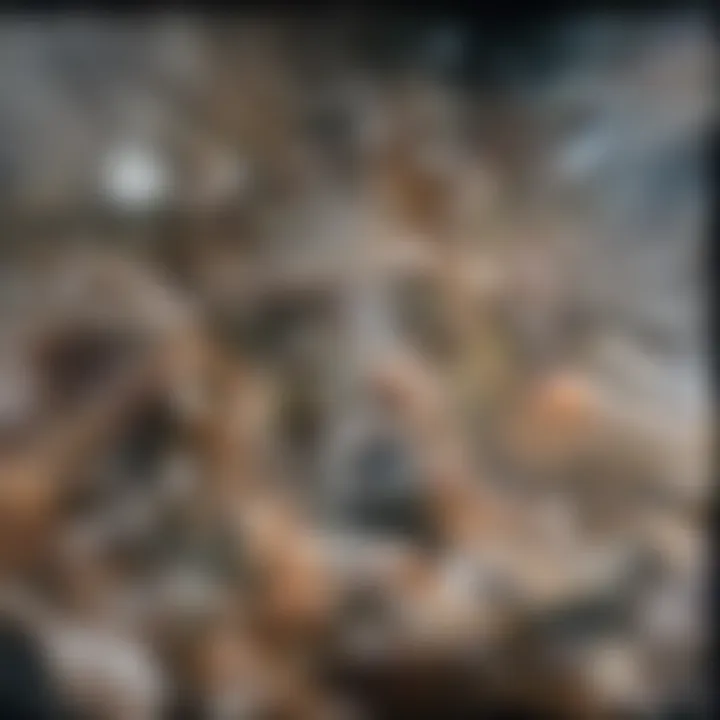
The future of data storage is moving towards innovative solutions that integrate quantum and optical storage with magnetic benefits. Researchers are investigating hybrid systems that combine the advantages of traditional magnetic storage with new technologies, leading to increased data density and speed.
A notable characteristic of future data storage methods is their potential for scalability. These new technologies are being designed to accommodate ever-growing amounts of data efficiently. The unique feature of these systems will likely be their ability to manipulate magnetic fields at a quantum level, allowing for faster and denser data storage solutions.
While the prospects are promising, challenges remain in making these technologies commercially viable. Addressing issues such as energy consumption and material costs will be vital. These future advancements hold promise for vast improvements in how we store and access data, reinforcing the significance of magnetism in modern technology.
Medical Applications
Magnetic Resonance Imaging (MRI)
Magnetic Resonance Imaging (MRI) is a key application of magnetism in the medical field. It uses strong magnetic fields and radio waves to produce detailed images of organs and tissues within the body. This non-invasive imaging technique has revolutionized diagnostics, allowing for better visualization without relying on ionizing radiation.
The primary characteristic of MRI is its ability to provide high-resolution images, giving clinicians crucial insights into patient conditions. It is popular because of its effectiveness in identifying abnormalities in soft tissues, such as the brain, muscles, and ligaments.
A unique feature of MRI systems is the powerful magnets they employ. These magnets generate strong enough magnetic fields to align the protons in water molecules within the body. The advantages of MRI include its safety and effectiveness. However, MRIs can be cost-prohibitive for some healthcare facilities and may require a significant investment in technology.
Magnetotherapy
Magnetotherapy is an alternative medical treatment that uses magnetic fields for therapeutic purposes. It is believed to promote healing and alleviate pain by influencing the cellular processes within the body. Advocates claim this method can enhance circulation, reduce inflammation, and encourage tissue repair.
A key characteristic of magnetotherapy is its non-invasive nature. Many patients prefer this approach, as it does not involve drugs or chemicals, making it appealing for those seeking natural treatment options. The unique feature lies in its application methods; devices can deliver varying magnetic field strengths and frequencies, tailored to individual needs.
While the benefits are touted by some, magnetotherapy remains controversial and lacks extensive scientific validation. This treatment does offer an interesting perspective on the potential for further exploration of magnetism's health applications. In summary, magnetotherapy presents a compelling area of research within medical magnetism.
"The understanding of how magnetism intersects with technology not only enhances our toolkit but also spurs innovation that can potentially transform multiple industries."
Challenges and Future Directions in Magnetism Research
The field of magnetism is both rich and complex, presenting a variety of challenges that researchers encounter. Addressing these challenges is crucial for advancing the practical applications of magnetism. The future directions in magnetism research will not only broaden scientific understanding but also enhance technological developments.
Current Limitations
Material Constraints
One significant challenge in magnetism research is material constraints. This aspect highlights the limitations of existing materials in achieving desired magnetic properties. The key characteristic of material constraints is that many materials do not possess the ideal magnetic characteristics necessary for specific applications. For example, common magnets like neodymium-iron-boron have excellent magnetization properties, but their cost and availability can hinder widespread usage. This makes them a popular choice in research but limits their application in many scenarios.
The unique feature of material constraints is how it affects research and development. Researchers continuously seek new materials or methods to overcome these limits. The advantages of focusing on material constraints include better resource allocation and innovations in material science. However, the disadvantages involve the time and resources needed to discover or synthesize new magnetizable materials, which can delay progress in the field.
Energy Efficiency
Another layer of complexity is energy efficiency in magnetic applications. Energy efficiency plays a critical role in the development of devices that utilize magnetic principles. The key characteristic here is balancing performance with energy consumption. Efficient designs reduce the environmental impact and enable sustainable use of resources.
Unique features of energy efficiency in magnetism include advancements in inductive charging and energy harvesting technologies. These technologies exemplify how optimizing energy use can increase overall system effectiveness in magnetic applications. Advantages include cost savings in the long run but downsides can involve initial research and development costs which are sometimes significant. Navigating these limitations is essential for integrating magnetism effectively in modern technology.
Future Research Avenues
Interdisciplinary Approaches
The future of magnetism research lies significantly in interdisciplinary approaches. This aspect encompasses collaboration across various scientific fields, such as physics, materials science, and engineering. The key characteristic of this approach is its ability to combine diverse methodologies and perspectives to tackle complex problems. It is beneficial for the article as it illustrates how integrated knowledge can lead to more innovative solutions in magnetism.
A unique feature of interdisciplinary approaches is the blend of techniques from different fields that can enhance the understanding of magnetic phenomena. For instance, using computational simulations alongside experimental methods allows for more comprehensive studies. The advantages include a richer perspective and potentially faster breakthroughs in the research process, but the challenge remains in coordinating such multifaceted efforts, which can be demanding.
Potential Breakthroughs in Material Science
There is significant potential for breakthroughs in material science related to magnetism. This aspect focuses on developing new magnetic materials that can outperform traditional options. A key characteristic is exploring properties of materials at microscopic levels to create improved magnets. This choice is beneficial as it promises to produce materials tailored for specific applications, enhancing performance and efficiency.
The unique features of these breakthroughs include the possibility of discovering new compounds that can exhibit superparamagnetic properties at room temperature. Advancements in material science not only aim to optimize magnetic properties but also involve considerations of production methods and cost-effectiveness. The potential advantages include a transformation of technologies that rely on magnetism, while disadvantages could involve the lengthy testing and validation processes required before practical applications are realized.
Ending
The conclusion serves as a vital component of this exploration into magnetism. It helps to synthesize and reinforce the essential concepts discussed throughout the article. By summarizing key points, readers are reminded of the fundamental principles and applications of magnetism that have been examined.
A clear understanding of these aspects is necessary for both academic and practical reasons. Magnetism plays a pivotal role in various technological advancements and scientific research, thus making its study highly relevant.
Summary of Key Points
To reiterate, this article covered significant areas in the field of magnetism:
- The development of magnetism from historical perspectives, illustrating how concepts evolved.
- Fundamental principles such as magnetic fields, forces, and the behavior of materials under different influences.
- Distinctions among various types of magnetism, including ferromagnetism, paramagnetism, and diamagnetism, elaborating on their distinctive characteristics and real-world applications.
- The relationship between magnetism and electricity, capturing the essence of electromagnetism and its underlying equations.
- Insights into modern technological applications, such as magnetic storage devices and medical imaging techniques.
- Discussion of current limitations in magnetism research and potential future directions, spotlighting the interdisciplinary nature of the field.
The summary guides readers through the scope of magnetism's implications in both scientific and practical contexts, ensuring a rounded comprehension of the subject.
The Future of Magnetism in Science and Technology
Looking ahead, the future of magnetism in science and technology appears promising. Researchers continue to seek innovative solutions that could overcome existing limitations, such as material constraints and energy efficiency challenges. The integration of magnetism with other fields like materials science and engineering paves the way for novel applications.
Future advancements may focus on areas such as:
- Spintronics, which utilizes the intrinsic spin of electrons for information processing and storage, could revolutionize computing.
- Quantum computing applications, where exploiting magnetic interactions might lead to unprecedented processing capabilities.
Magnetism also holds potential in various domains, from energy harvesting to medical technology. The continuous exploration of nanomaterials may introduce new magnetic phenomena that can be harnessed across multiple industries. As this field evolves, it will likely intersect with emerging technologies, thereby enhancing its significance across scientific communities.
"The study of magnetism is not only about understanding how forces interact; it is about unveiling the fundamental essence of nature that governs our universe."