Understanding Magnetism: A Comprehensive Overview
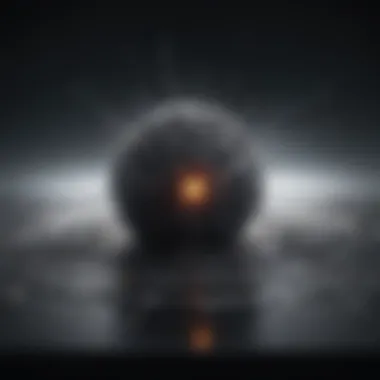
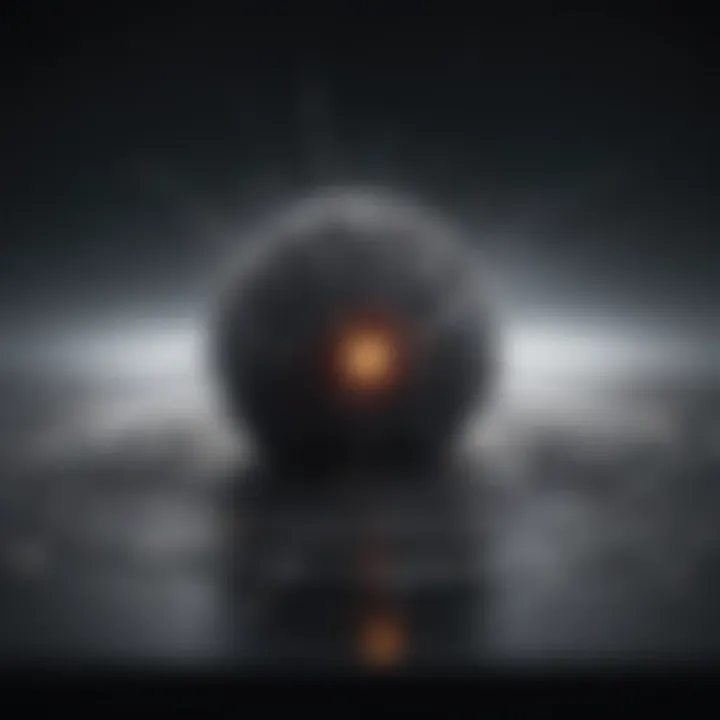
Intro
Magnetism is a fundamental physical phenomenon seen in many aspects of the natural world. This article shall dissect this complex subject, aiming to provide clarity on its core principles, characteristics, and numerous applications. Understanding magnetism is not a mere academic pursuit; it plays a critical role in various fields such as physics, engineering, health, and even everyday technology.
Throughout history, scientists have been fascinated by magnetic forces. From early explorations of lodestones to modern applications like magnetic resonance imaging, the journey of understanding magnetism reflects human ingenuity and scientific progress. Clarity on these concepts lays the groundwork for further exploration in both theoretical study and practical applications.
This comprehensive overview will take the reader through key research findings in magnetism, break down complex concepts to enhance understanding, and reveal the significance of magnetism in our daily lives.
Key Research Findings
Overview of Recent Discoveries
Recent advancements in magnetic research have uncovered new insights into how magnetism operates at quantum levels. Researchers have made significant strides in understanding quantum magnetism, which investigates the behavior of magnetic materials in the context of quantum mechanics. Notable discoveries involve the examination of topological insulators and their capacity to carry electrical current without resistance through magnetic fields. This understanding is paving the way for innovations in energy efficiency and data storage technologies.
Additionally, the use of magnetic materials in spintronics, which employs the intrinsic spin of electrons for information processing, has garnered attention. This field promises a plethora of benefits, including faster processing speeds and reduced power consumption in electronic devices.
"Advances in spintronics could lead to the next generation of computers, which will be significantly more powerful and efficient."
Significance of Findings in the Field
The implications of these findings extend far beyond theoretical physics. Everyday products, such as smartphone storage and electric motors, utilize magnetic principles. The evolution of magnetism research also contributes to medical technology. Devices like MRI machines rely heavily on magnetic fields for non-invasive imaging.
Moreover, these findings hold potential for transformative technological development. The combination of quantum mechanics and magnetism may lead to breakthroughs in superconductivity, which can revolutionize power grids by allowing electricity to flow without resistance.
Breakdown of Complex Concepts
Simplification of Advanced Theories
Understanding advanced theories in magnetism such as electromagnetic induction or Lorentz force can be daunting. To clarify, electromagnetic induction refers to the generation of voltage in a conductor through a changing magnetic field. Applied in devices like generators and transformers, this principle is key to power generation.
Lorentz force represents the influence of magnetic and electric fields on charged particles. If a charged particle moves through a magnetic field, it experiences a force perpendicular to both the magnetic field and the direction of its motion. This relationship is critical in determining the motion of electrons in biotechnology and communications.
Visual Aids and Infographics
Utilizing visual aids can significantly enhance comprehension. Infographics depicting the relationship between electricity and magnetism illustrate complex interactions more simply. Flowcharts can also be helpful, outlining how magnetic fields interact with various objects, facilitating an intuitive understanding of the principles discussed.
By simplifying these complex ideas, we can make magnetism accessible to students, researchers, and professionals alike. Understanding these fundamental principles will not only enrich academic pursuits but also foster innovative applications in technology and industry.
Intro to Magnetism
Magnetism is an essential aspect of physics that influences various facets of our daily lives and technological advancements. Understanding magnetism equips individuals with the knowledge to grasp not only natural phenomena but also the underlying principles that drive countless technological applications. This section serves as a gateway to the broader discourse on magnetism, outlining its significance within the context of the article.
As we begin to explore magnetism, it is crucial to recognize its relevance. From navigation to data storage, the effects of magnetic forces are pervasive and vital. By investigating how magnetism operates, we gain insight into its fundamental principles, which directly impact our technological landscape and interaction with the environment.
Definition of Magnetism
Magnetism refers to a physical phenomenon produced by the motion of electric charge, which results in attractive and repulsive forces between objects. It occurs in certain materials and can affect other materials at various distances. This phenomenon can manifest in various ways, such as in the attraction between magnets or the alignment of magnetic domains within ferromagnetic substances.
In essence, magnetism is a force that operates through magnetic fields. These fields extend around magnets and certain materials, defining the spatial influence of magnetic forces. Understanding this definition is foundational as it lays the groundwork for deeper exploration into the complex behaviors associated with magnetism.
Historical Context of Magnetism
The study of magnetism can be traced back to ancient civilizations. The earliest recorded observations of magnetism began with the discovery of lodestone, a naturally magnetized mineral found in iron ore. Ancient Greeks, notably Thales of Miletus, explored the peculiar properties of lodestone and its ability to attract small iron objects.
Over centuries, various cultures adopted these findings. The Renaissance period led to significant advancements in the understanding of magnetism. Figures such as William Gilbert made strides in the scientific examination of magnets. He proposed that Earth itself is essentially a giant magnet, a concept that later influenced navigational technologies.
In modern times, the industrial revolution brought about the practical use of magnetism in electric motors and generators. Today, ongoing research in areas like quantum mechanics continues to expand our understanding of magnetism, revealing its fundamental complexities. This historical overview underscores the importance of magnetism, emphasizing its evolution from a mere curiosity to a cornerstone of modern technology.
Fundamental Principles of Magnetism
The fundamental principles of magnetism serve as the foundation for understanding how magnetic fields and forces interact within various materials and systems. This section is crucial for grasping how magnetism functions, impacting both theoretical studies and practical applications.
Magnetic Fields Defined
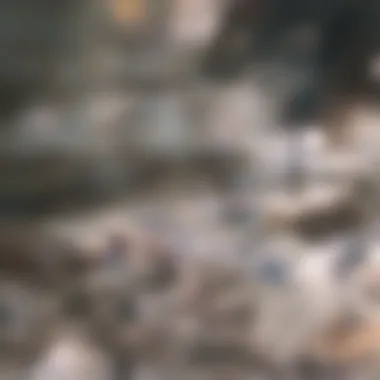
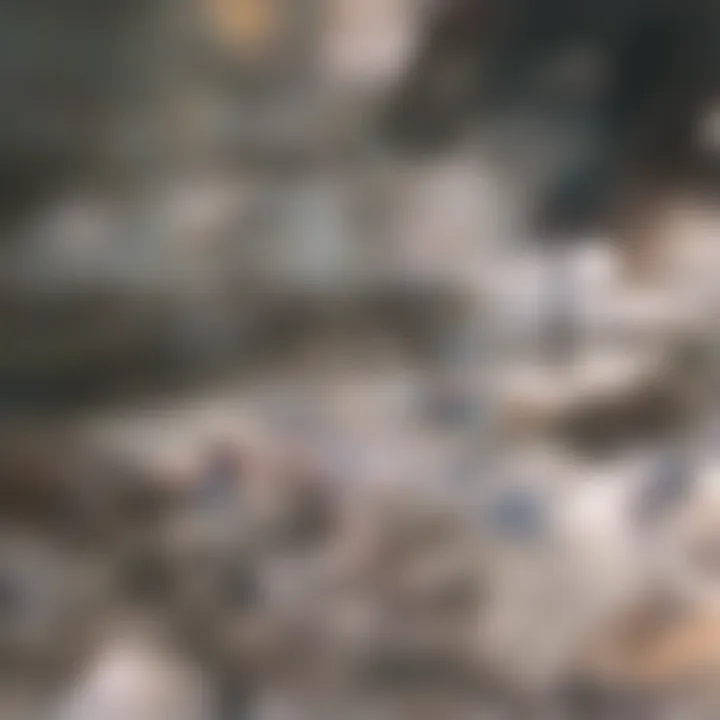
A magnetic field is an invisible force field surrounding magnets and electric currents. It is the region where magnetic forces can be experienced. The concept was first clearly defined by scientists such as Andrรฉ-Marie Ampรจre and Michael Faraday. The magnetic field is represented by magnetic field lines; these lines show the direction and strength of the magnetic force. The density of the lines indicates the strength of the field.
Areas where these lines are dense indicate stronger magnetic fields, while sparse lines show weaker fields. The unit of measure for magnetic fields is the Tesla, named after Nikola Tesla. Understanding magnetic fields is vital in many technological contexts, such as in electric motors and transformers.
Magnetic Forces and Interactions
Magnetic forces arise due to the movement of electric charges. When charges move, they create a magnetic field, and an interaction occurs when this magnetic field meets another charge or magnetic field. The phenomena of attraction and repulsion are the most common interactions that occur in magnetism. Opposite poles attract, while like poles repel. This principle governs the behavior of magnets in everyday life.
The force between two magnetic objects can also be calculated using formulas derived from theoretical principles. For instance, the Lorentz force law illustrates how charged particles move through magnetic fields, a concept essential in the design of many electronic devices.
The Nature of Magnetic Poles
Every magnet has two poles โ a north and a south pole. These poles are where the magnetic field is strongest. The north pole is defined as the pole that points towards the Earth's North when suspended freely. One important property of magnetic poles is that they cannot exist independently. Cutting a magnet in half does not produce a separate north or south pole; instead, two new complete magnets are formed, each with its own north and south pole.
This intrinsic nature of magnetic poles is foundational in physics. It reinforces the idea that magnetism is a dual system, where magnetic forces are a result of interactions between these opposing poles. This concept has significant implications in magnetic materials and devices.
"Magnetism is a fundamental force that governs the interaction of materials and fields, essential for numerous technologies in modern society."
Types of Magnetism
Understanding the various types of magnetism is essential for grasping the full scope of magnetic phenomena. Each type exhibits unique properties and behaviors that are crucial to applications in both industrial and scientific contexts. Moreover, recognizing these types allows for a better appreciation of their significance in technology and nature. There are three main categories of magnetism: ferromagnetism, paramagnetism, and diamagnetism. Each of these categories presents distinct characteristics that influence material behavior in magnetic fields.
Ferromagnetism
Ferromagnetism is perhaps the most familiar form of magnetism. It occurs in materials such as iron, cobalt, and nickel. These materials can be permanently magnetized, giving rise to strong magnetic fields. The key feature of ferromagnetic materials is their ability to retain magnetization even after an external magnetic field is removed. This happens because of the alignment of magnetic moments within the material.
In ferromagnetic materials, regions called magnetic domains exist. Within these domains, the magnetic moments of atoms align in the same direction. When exposed to an external magnetic field, these domains can grow and align with the field, leading to an overall magnetization of the bulk material. This alignment is maintained unless significant energy, such as heat or physical impact, disrupts the domains.
Ferromagnetism plays a critical role in a variety of applications, including:
- Electric Motors: The interaction of ferromagnetic materials with magnets leads to efficient motor operation.
- Magnetic Storage: Hard drives utilize ferromagnetism to store data in a stable format.
- Transformers: Ferromagnetic cores optimize the efficiency of transformers in power distribution.
Paramagnetism
Paramagnetism occurs in materials that have unpaired electrons, resulting in a net magnetic moment. Unlike ferromagnetic materials, paramagnetic materials do not have a spontaneous magnetization. Instead, they only exhibit magnetic properties in the presence of an external magnetic field. When such a field is applied, the magnetic moments tend to align with the field, thus enhancing the overall magnetic influence.
The alignment of magnetic moments in paramagnetic materials is weak and disappears once the external field is removed. Common examples of paramagnetic materials include aluminum and platinum. These materials are characterized by:
- Weak Magnetic Response: The induced magnetization is quite small compared to ferromagnetic materials.
- Temperature Dependence: The magnetic susceptibility of paramagnetic materials increases with temperature, following Curieโs Law, indicating that lower temperatures yield higher magnetic susceptibility.
Diamagnetism
Diamagnetism is a form of magnetism that is present in all materials, but it is generally quite weak. In diamagnetic materials, the magnetic moments of atoms tend to oppose the external magnetic field. This results in a very slight repulsion from the magnetic field. This behavior is due to the principle of Lenz's law, which states that changes in magnetic fields induce currents that create opposing magnetic fields.
Notable characteristics include:
- Universal Presence: Diamagnetism exists in every material to some extent, but its effects are only noticeable in non-ferromagnetic and non-paramagnetic materials.
- Examples of Diamagnetic Materials: Common materials such as copper, bismuth, and graphite display significant diamagnetic behavior.
"In a strong magnetic field, a diamagnetic material may experience a force that lifts it against gravity, demonstrating the subtlety of magnetic interactions."
In summary, the types of magnetismโferromagnetism, paramagnetism, and diamagnetismโeach have unique properties and applications. Knowledge of these categories not only enriches our understanding of magnetic materials but also aids in innovative technology development. Recognizing the distinctions between them enables researchers and professionals to choose appropriate materials for specific applications in technology, engineering, and beyond.
Magnetic Materials
Magnetic materials are critical for understanding magnetism. They are the backbone of many technologies and natural phenomena. Their unique properties allow them to interact with magnetic fields in specific ways. Variations in these materials lead to different magnetic behaviors. Understanding them is essential for both theoretical and practical applications.
These materials have numerous benefits. They enhance performance in devices like motors, memory storage, and transformers. They also play an important role in medical devices, such as MRI machines. Thus, the exploration of magnetic materials is vital for advancement in various scientific fields.
Characteristics of Magnetic Materials
Magnetic materials possess distinctive characteristics. These properties govern how the material behaves in a magnetic field. Common characteristics include permeability, saturation magnetization, and coercivity.
- Permeability refers to how easily a material can become magnetized. High permeability indicates that a material can support a magnetic field much more effectively.
- Saturation magnetization defines the maximum magnetic moment per unit volume that a material can achieve. When a material reaches this point, it cannot hold any more magnetic field.
- Coercivity describes a material's resistance to becoming demagnetized when an external magnetic field is removed. A material with high coercivity maintains its magnetization, which is important in applications where permanent magnets are crucial.
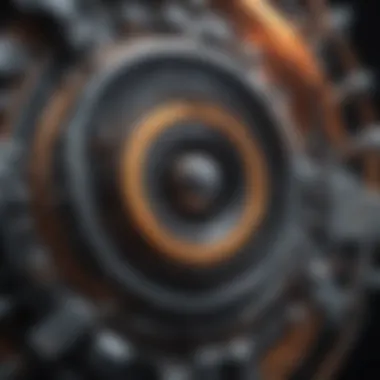
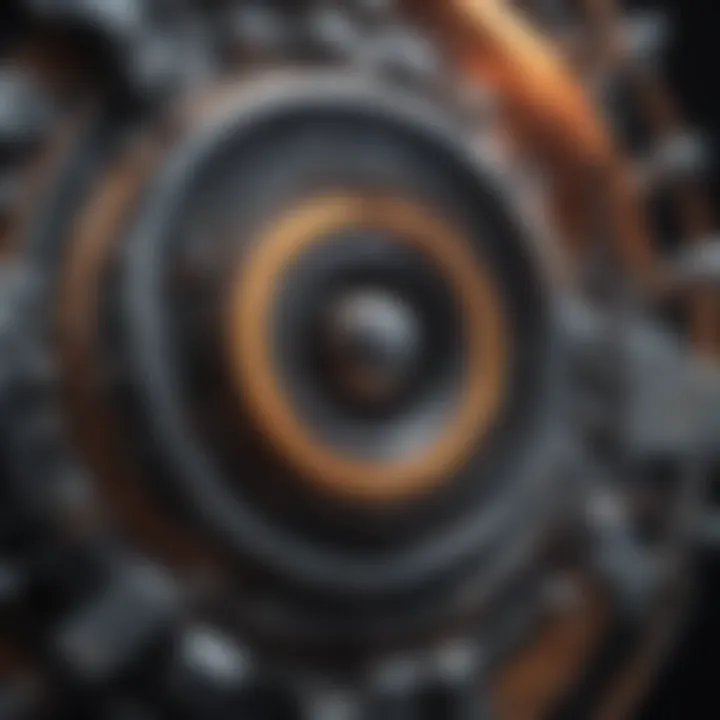
Understanding these characteristics allows scientists and engineers to select the right material for specific applications. This knowledge can drive innovation in various technologies.
Classification of Magnetic Materials
Magnetic materials can be classified into several categories based on their magnetic properties. The most common classifications include ferromagnetic, paramagnetic, and diamagnetic materials.
- Ferromagnetic materials like iron and cobalt exhibit strong magnetic behavior. They can be magnetized easily and retain their magnetization.
- Paramagnetic materials such as aluminum have weaker interactions with magnetic fields. Though they become magnetized in an external field, their magnetization disappears once the field is removed.
- Diamagnetic materials like bismuth exhibit a negligible response to magnetic fields. They are weakly repelled by magnets and are characterized by a negative susceptibility.
Understanding these classifications helps determine the suitable magnetic materials for diverse applications. Different situations call for specific materials based on the required performance and characteristics.
Applications of Magnetism
The applications of magnetism span various fields, highlighting its immense importance in modern technology and nature. The utilization of magnetic principles has transformed countless industries, from electronics to medicine. Understanding how magnetism is applied helps to comprehend its role in scientific innovation and everyday life. The benefits include enhanced efficiency in technology and better comprehension of natural phenomena.
Use in Technology
Electronics and Computing
The role of magnetism in electronics and computing is foundational. Devices like hard disk drives, magnetic tape, and other storage mediums utilize magnetic fields to read and write data. This characteristic of using magnetism for data storage is crucial, as it allows for high capacity and longevity of information storage.
The unique feature of electronics based on magnetic principles is their ability to manage information at speeds that surpass other forms of storage, such as optical drives. While beneficial, these technologies require meticulous calibration to avoid data corruption.
Data Storage Solutions
Data storage solutions leveraging magnetism encompass a variety of technologies, like Solid State Drives (SSDs) and traditional hard drives. Magnetic storage offers high data density. This feature is valuable as it allows for compact devices with ample storage.
The advantage of magnetic data storage lies in their cost-effectiveness compared to non-magnetic solutions. There are disadvantages too, mainly concerning speed and durability. They can be susceptible to physical shocks, leading to data loss.
Medical Imaging Technologies
Medical imaging technologies, such as Magnetic Resonance Imaging (MRI), showcase another vital application of magnetism. MRI uses strong magnetic fields and radio waves to generate detailed images of organs and tissues. This characteristic provides a non-invasive method for diagnosing medical conditions, which is highly beneficial in clinical settings. The unique feature of MRI technology is its ability to produce high-resolution images without exposure to ionizing radiation. However, the reliance on powerful magnets can lead to challenges, such as the cost of devices and the need for specialized locations for implementation.
Magnetism in Nature
Earth's Magnetic Field
Earth's magnetic field plays a critical role in protecting the planet from cosmic radiation and charged particles from the sun. This field is crucial for maintaining the atmospheric conditions necessary for life.
The key characteristic of the Earth's magnetic field is its ability to deflect solar wind, which otherwise would erode the atmosphere over time. This protection is a remarkable benefit, helping sustain life on Earth. However, changes in the magnetic field can have impacts on weather patterns, which is a concern for scientists studying climate change.
Animal Navigation
Animal navigation displays an intriguing aspect of magnetism in nature. Many species, including birds and sea turtles, rely on Earth's magnetic field for migration and orientation. This characteristic allows animals to travel vast distances with precision.
The unique feature of this navigational ability is unparalleled in nature, providing animals with a natural means to determine their position relative to the planet. However, this phenomenon raises questions about how environmental changes may affect migratory patterns and animal behavior.
Understanding the applied aspects of magnetism is essential for grasping how this fundamental force interweaves into numerous facets of technology and nature.
Theoretical Framework
The theoretical framework is essential for understanding magnetism. It provides the underlying principles and equations that describe magnetic phenomena. By examining this framework, readers can grasp the mathematical and conceptual foundations of magnetism. This understanding is crucial for both academic research and practical applications in technology.
Maxwell's Equations
Maxwell's Equations form the cornerstone of electromagnetism. They describe how electric fields and magnetic fields interact. These four equations give a comprehensive view of how charged particles generate electromagnetic fields. Each equation has a specific role:
- Gauss's Law for Electricity: Describes how electric charges create electric fields.
- Gauss's Law for Magnetism: States that there are no magnetic monopoles; magnetic field lines are continuous loops.
- Faraday's Law of Induction: Explains how a changing magnetic field can induce an electric current.
- Ampรจre-Maxwell Law: Relates magnetic fields to the current producing them and accounts for changing electric fields.
Understanding these laws is vital for grasping how magnetism underpins various technologies such as motors, generators, and transformers. They illustrate the interplay of electric and magnetic fields, which is crucial in many scientific and engineering disciplines.
Quantum Mechanics and Magnetism
Quantum mechanics provides another layer of understanding when it comes to magnetism. At the atomic level, particles exhibit behaviors governed by quantum effects. One key aspect is spin, an intrinsic form of angular momentum. Spin gives rise to magnetism in materials, particularly in ferromagnetic substances.
In quantum theory, the behavior of electrons and their arrangements significantly affect magnetic properties. For example, in ferromagnetic materials like iron, the alignment of electron spins leads to a net magnetic moment. This phenomenon cannot be explained solely by classical physics.
Exploring this topic requires understanding concepts such as:
- Exchange Interaction: A quantum phenomenon that influences magnetic ordering.
- Quantized Energy Levels: In magnetic fields, these levels split, leading to observable magnetic properties.
This intersection of quantum mechanics and magnetism has practical implications too, such as the development of quantum computing and spintronic devices, which exploit electron spin for data processing and storage.
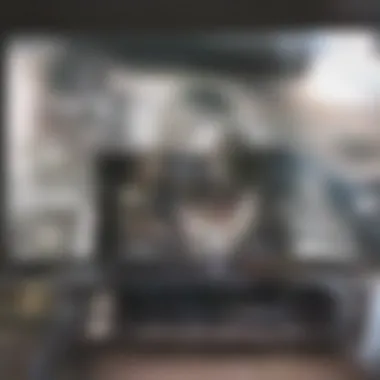
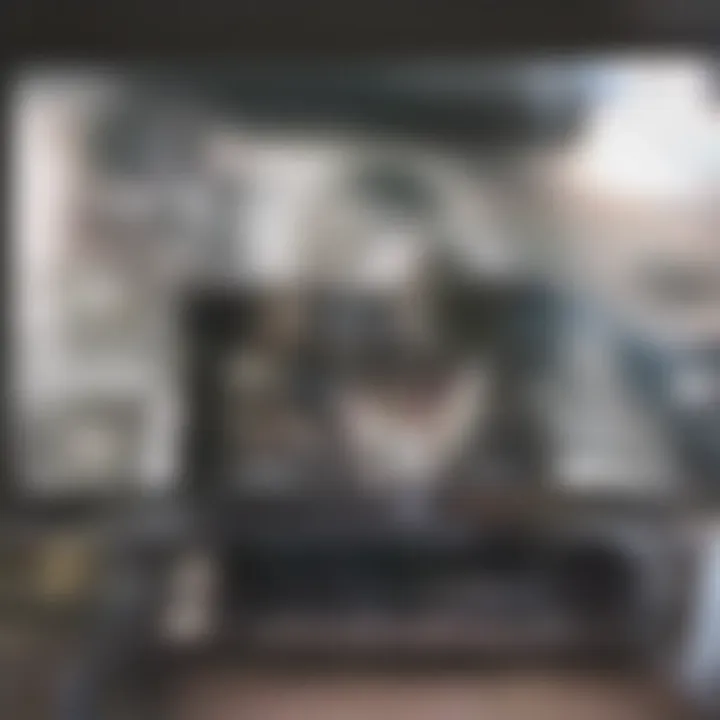
"The fusion of quantum mechanics with magnetism opens up new frontiers in technology and research."
Overall, the theoretical framework provided by Maxwell's equations and quantum mechanics enriches our comprehension of magnetism, making it applicable across various fields, from theoretical physics to practical engineering.
Recent Advances in Magnetic Research
Recent advances in magnetic research have yielded significant insights that enhance our understanding of magnetism and its applications. These developments not only demonstrate the potential of magnetism in cutting-edge technologies but also reflect ongoing efforts to overcome existing limitations in the field. As our world increasingly relies on innovations driven by magnetic phenomena, comprehending these recent advancements is essential for students, researchers, educators, and professionals alike.
High-Temperature Superconductors
High-temperature superconductors, typically defined as materials that can conduct electricity without resistance at temperatures above the boiling point of liquid nitrogen, are a focal point in recent magnetic research. Unlike traditional superconductors, which require extremely low temperatures, these new materials open pathways for more practical applications. The potential of high-temperature superconductors extends to various sectors, including power grids, transportation systems, and even magnetic levitation.
Researchers have made progress in both discovering new high-temperature superconducting materials and improving the existing ones. For instance, compounds like yttrium barium copper oxide (YBCO) have shown remarkable superconducting properties at higher temperatures. The effective use of these materials could lead to more efficient transmission of electricity, reducing energy losses significantly.
Moreover, understanding the underlying mechanisms that enable high-temperature superconductivity remains a challenge. The collective behavior of electrons in these materials interacts intricately with the magnetic fields surrounding them. Continued research is necessary to unravel these complex relationships and to find even more effective superconductors in the future.
"Advancing the field of high-temperature superconductors could transform energy technologies, providing more efficient power solutions globally."
Spintronic Devices
Spintronic devices represent another intriguing recent advancement in magnetic research. Unlike traditional electronic devices that rely primarily on the charge of electrons, spintronics harnesses the intrinsic spin of electrons, coupled with their charge. This dual functionality offers advantages such as increased data storage, faster processing speeds, and lower power consumption.
Spintronic applications can be found in data storage solutions like MRAM (Magnetoresistive Random Access Memory). MRAM is gaining momentum due to its non-volatility and performance, which surpass traditional flash memory. This technology benefits from the manipulation of electron spins to achieve reliable memory storage with high endurance.
Moreover, researchers are exploring quantum spintronic devices that could operate at even higher speeds, potentially revolutionizing computing. Such devices have the potential for quantum computing capabilities, making them crucial for future advancements in technology.
In summary, the frontiers of magnetic research, such as high-temperature superconductors and spintronic devices, showcase not only the vast possibilities of magnetism in technology but also present challenges that need addressing. Understanding these advances will help guide future research directions and applications.
Challenges and Future Directions
The exploration of magnetism holds ongoing relevance in science and technology. As we move further into the 21st century, the implications of magnetic properties become ever more significant. Here, we will discuss some pressing challenges facing current magnetic technologies and consider what the future may hold.
Limitations of Current Technologies
Current magnetic technologies encounter several limitations.
- Material Constraints: Common magnetic materials like iron and nickel have limitations in terms of availability and performance at extreme conditions. The need for new materials that can sustain high temperatures without losing their magnetic properties remains unaddressed.
- Energy Efficiency: Many systems that utilize magnetic forces, such as motors and generators, suffer from energy losses. Improvements are necessary to make them more efficient and reduce overall energy consumption.
- Scale and Integration: Integrating magnetic systems into existing technologies often proves difficult. Many designs are not scalable, limiting their widespread application in industries such as renewable energy.
These limitations must be acknowledged, as they inform the direction of future research, particularly as it pertains to innovation in material science and engineering.
Potential for Future Discoveries
Looking ahead, the potential for breakthroughs in magnetic research is profound. Several areas present opportunities for exploration and advancement:
- Advanced Materials: Research into high-temperature superconductors, such as those based on cuprates and iron, could revolutionize the field. These materials hold the promise of efficient energy transfer and unparalleled applications.
- Nanotechnology: The field of nanotechnology may provide insights into manipulating magnetic properties at the atomic level. New magnetic nanomaterials could lead to advancements in data storage and electronic devices.
- Quantum Magnetism: Exploring quantum effects in magnetic materials might unlock new paradigms for data storage and computational speed.
The potential for creativity in magnetic research is vast. Continued investment and exploration in these areas may yield technologies that exceed our current understanding.
"The future of magnetism is not merely about overcoming past shortcomings; it's about discovering uncharted scientific territories that could enhance human capabilities."
In summary, addressing the limitations of current magnetic technologies while investing in future discoveries will provide critical avenues for progress. This could enhance both scientific and practical applications of magnetism in ways we have yet to envision.
End
The conclusion of this article emphasizes the significant role magnetism plays across multiple domains including technology and nature. Understanding magnetism not only illuminates fundamental physical principles but also bridges historical advancements with modern applications. As we reviewed the various aspects of magnetism, we see its influence permeating various scientific fields.
Summary of Key Points
The key points discussed in this article provide a comprehensive overview of magnetism:
- Definition and Historical Context: Magnetism has evolved from rudimentary understanding to an intricate science interwoven with various technologies.
- Fundamental Principles: Magnetic fields, forces, and poles form the bedrock of magnetism, influencing how we understand interactions at both micro and macro levels.
- Types of Magnetism: Ferromagnetism, paramagnetism, and diamagnetism showcase the diverse range of magnetic behaviors found in materials.
- Applications: From electronics to navigation, the applications of magnetism are varied and vital, driving technological innovation.
- Theoretical Framework: Maxwell's Equations and quantum mechanics highlight the advanced understanding of electromagnetic phenomena.
- Recent Advances: High-temperature superconductors and spintronic devices represent the frontiers of magnetic research.
- Challenges: Exploring limitations in current technologies can lead to future discoveries.
The Importance of Magnetism in Science
Magnetism is not just a physical phenomenon; it is instrumental in advancing numerous scientific fields. In physics, it lays the groundwork for understanding electromagnetism, critical for both theoretical studies and practical applications. The behavior of magnetic materials is essential in developing new technologies, such as high-performance computing and efficient energy systems.
Moreover, the study of magnetism contributes to our understanding of biological systems, such as animal navigation influenced by Earthโs magnetic field. This intersection of disciplines illustrates how magnetism serves both fundamental scientific inquiry and practical human needs, underlining its importance in ongoing research and innovation.
As we contemplate the challenges and possibilities surrounding magnetism, it is clear that continued exploration in this field holds promise for future breakthroughs, emphasizing its fundamental and transformative nature in science and technology.