Exploring the Complexities of Human Neural Networks
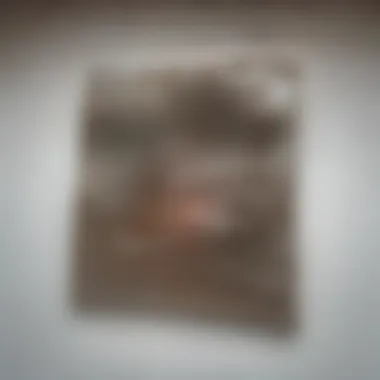
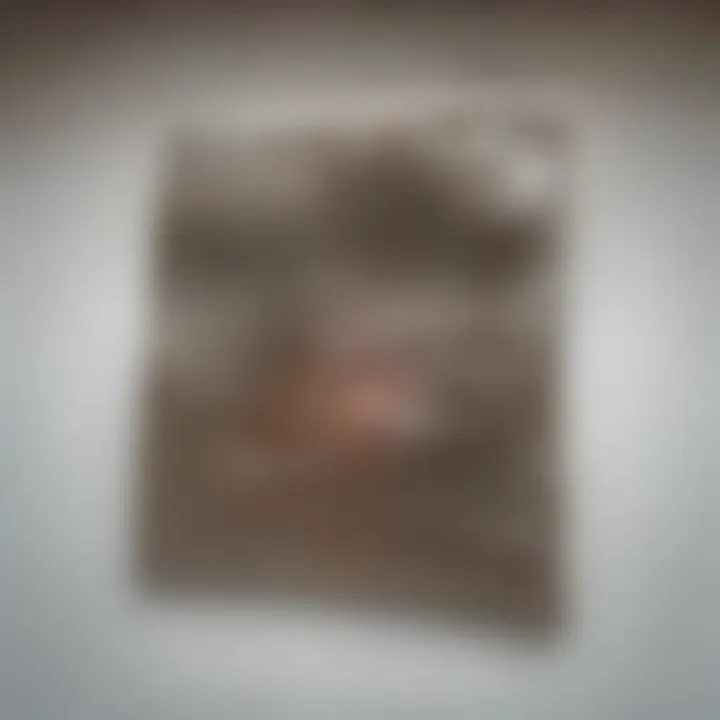
Intro
The human neural network represents one of the most intricate systems known to science. Understanding its structure and function provides insights into how we think, learn, and adapt. This section lays the groundwork for exploring the complexities of neurons, synapses, and neuroplasticity. By illuminating how these components interact, we can appreciate the broader implications this knowledge holds for fields like artificial intelligence and neurological sciences.
Key Research Findings
Overview of Recent Discoveries
Recent studies have greatly expanded our understanding of human neural networks. Researchers have revealed the dynamic nature of synaptic connections and how they adapt over time. For example, studies suggest that synaptic strength is not static, but changes in response to activity and stimuli. This phenomenon, known as synaptic plasticity, is fundamental in learning and memory processes. New imaging techniques, such as functional MRI, allow scientists to visualize brain activity in real-time, offering clearer insights into neural function and connectivity.
Significance of Findings in the Field
The implications of these findings are significant. By understanding the adaptable nature of neural networks, we can develop better educational strategies that align with how the brain learns. Additionally, this knowledge is crucial for addressing neurological disorders. Conditions such as Alzheimer's disease and depression are linked to dysfunctional neural networks. Gaining insights into these networks allows for targeted interventions and therapies. Furthermore, the parallels between biological neural networks and artificial intelligence systems have been a point of extensive exploration, leading to innovations in machine learning.
"The exploration of human neural networks not only deepens our understanding of cognitive processes but also inspires advancements in how we create intelligent systems."
Breakdown of Complex Concepts
Simplification of Advanced Theories
Understanding neural networks can seem daunting. Breaking down concepts into simpler terms can help. For instance, at its core, a neuron is like a messenger. It receives signals and transmits impulses. Neurons connect to one another through synapses, which allow communication between different parts of the brain. Neuroplasticity, the ability of these neural pathways to change, underpins our capacity to learn or recover from brain injuries.
Visual Aids and Infographics
To further grasp these complexities, visuals can be invaluable. Diagrams showing the structure of a neuron, the synaptic connections, and pathways involved in neuroplasticity can enhance understanding. For example, a simple infographic illustrating a synapse can clarify how neurotransmitters travel across the gap between neurons, embodying the foundation of neural communication.
- Diagram of a neuron showing:
- Example of synaptic transmission:
- Dendrites
- Cell body
- Axon
- Synapse
- Action potential arrives
- Neurotransmitters release
- Signal received by next neuron
In summary, comprehending human neural networks is vital for both scientific research and practical applications. It not only informs therapeutic practices in neurology but also shapes how we develop technology. The interplay between biology and technology continues to evolve as we uncover the secrets of the human mind.
Prelude to Human Neural Networks
Understanding the human neural networks is crucial as it lays the foundation for comprehending how our brain operates at both physiological and psychological levels. The complexities of these networks play a vital role in various domains including neuroscience, psychology, and artificial intelligence. This exploration is not just about brain structure, but also how that structure influences function and behavior.
As technology advances, the implications of neural networks extend beyond academia into practical applications that affect everyday life. For students, researchers, educators, and professionals alike, grasping the core principles behind these networks can lead to deeper insights into human cognition, mental health, and the development of intelligent systems that mimic human thought processes.
Definition and Relevance
A human neural network consists of interconnected neurons that communicate through synapses. These connections are responsible for transmitting information throughout the brain and body. Understanding this definition adds depth to our comprehension of neurological functions and cognitive processes.
Relevance of such networks can be observed in various fields. For instance:
- Neuroscience: Insight into brain disorders and therapeutic approaches
- Psychology: Understanding behaviors, emotions, and decision-making processes
- Artificial Intelligence: Development of algorithms based on neural structures for machine learning
Thus, recognizing the structure and function of human neural networks promotes advances not only in science but also in technological innovation.
Historical Context
The study of neural networks dates back to the early 20th century, with pioneering work by scientists like Santiago RamΓ³n y Cajal, known as the father of modern neuroscience. Cajal's meticulous drawings of neurons laid the groundwork for understanding their morphology and subsequent neural connections.
In the following decades, researchers made significant strides. The discovery of neurotransmitters in the mid-20th century by scientists like Otto Loewi demonstrated how chemical signals facilitate communication between neurons. This marked a pivotal moment in neuroscience, as it opened avenues for exploring cognitive functions and psychological phenomena based on physiological mechanisms.
The historical evolution of understanding human neural networks set the stage for contemporary research that continues to uncover the intricacies of the brain. The knowledge gained through these historical milestones is essential for modern applications in treatment strategies and advancements in related technologies.
Anatomical Structure of Neural Networks
The anatomical structure of neural networks is fundamental to understanding how the human brain processes information. It provides the physical framework that enables the functionality of various cognitive processes. By exploring this structure, we can reveal the intricate systems that allow for communication within the brain and between different body parts. A clear understanding of neural anatomy lays the groundwork for comprehending more complex topics, such as neuroplasticity and cognitive functioning.
Neurons: The Basic Building Blocks
Neurons are the essential building blocks of the nervous system. Each neuron functions as a specialized cell that transmits information through electrical and chemical signals. The basic structure includes the cell body, dendrites, and an axon, which allow neurons to communicate effectively. This communication is vital for everything from reflexes to higher-level processing such as reasoning and decision-making.
Neurons' unique structure enables the quick transmission of signals. The ability to send and receive information efficiently is crucial for the brain's functioning. Understanding neurons provides insight into how various brain regions interact and how damage to specific areas can impact overall cognition.

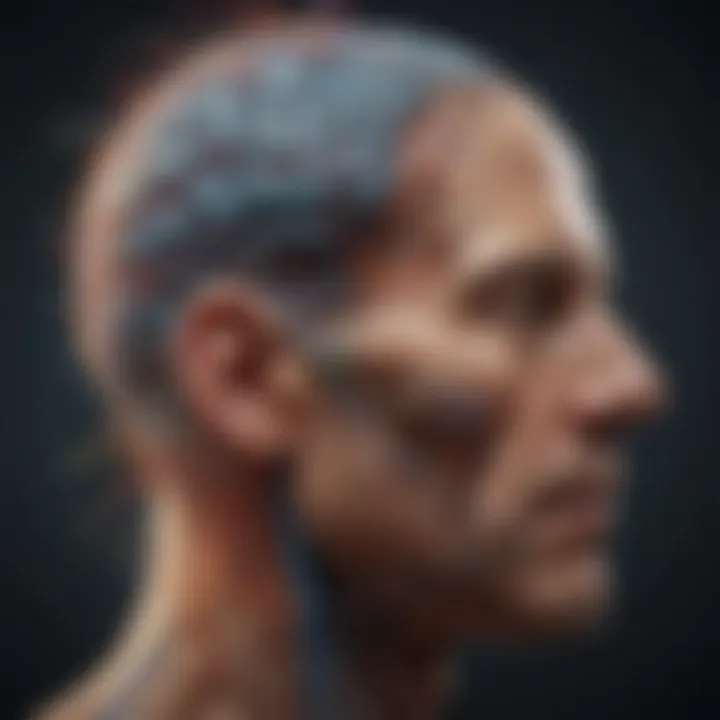
Types of Neurons
Neurons can be categorized into three main types, each serving a distinct role in the nervous system. These types are sensory neurons, motor neurons, and interneurons.
Sensory Neurons
Sensory neurons play a crucial role in relaying information from sensory receptors to the central nervous system. They are responsible for the sensations we experience, such as touch, sight, sound, and taste. Their primary characteristic is their ability to convert external stimuli into electrical impulses that the brain can interpret. This facet makes sensory neurons vital for perception and interaction with the surrounding environment.
A unique feature of sensory neurons is their varied structure, which can adapt to different types of stimuli. For instance, photoreceptors in the eyes are specialized sensory neurons that detect light. This specialization allows for precise processing of environmental cues, which benefits the article's understanding of perception.
Motor Neurons
Motor neurons are equally important as they transmit signals from the central nervous system to muscles and glands, facilitating movement and response to stimuli. A key characteristic of motor neurons is their ability to activate muscles, enabling physical actions ranging from simple reflexes to complex movements.
Their distinct feature is the long axon that extends from the spinal cord to the target muscle. This allows for rapid communication, essential during activities requiring coordination. The implications of studying motor neurons are significant. They provide insight into not only voluntary movements but also involuntary responses that are crucial in various physiological functions.
Interneurons
Interneurons serve as connectors between sensory and motor neurons. They are predominantly located in the brain and spinal cord. Their contribution is critical in processing information by integrating signals from multiple sources before relaying responses. One key characteristic of interneurons is their ability to form complex networks, allowing for sophisticated responses to sensory input.
A unique feature is that they allow for reflex arcs, which are direct pathways that enable the body to react swiftly to stimuli. This characteristic underscores the importance of interneurons in quick decision-making processes. By engaging with this type of neuron, researchers can enhance their understanding of neural circuitry and how different brain areas work together.
Synaptic Connections and Transmission
Synaptic connections represent the pathways through which neurons communicate. These connections are formed at synapses, where neurotransmitters are released and bind to receptors on the receiving neuron. This interaction dictates how impulses are transmitted and whether they result in an excitatory or inhibitory response.
Synaptic transmission is essential in maintaining the flow of information within neural networks. It allows for the modulation of signals, contributing to the dynamic nature of neural activity. Understanding synaptic connections can provide valuable insights into both normal brain function and the effects of neurodegenerative conditions.
Functional Dynamics of Neural Networks
Understanding the functional dynamics of neural networks is essential for grasping how biological systems operate. This section explores how various components interact to facilitate communication within the nervous system. By delving into neural communication and network activity, we uncover the underlying mechanisms that define behavior, perception, and cognitive function. Such a comprehension is crucial not only to neuroscience but also to advancements in artificial intelligence and related fields.
Neural Communication
Action Potentials
Action potentials are brief electrical impulses that are essential for neuronal communication. When a neuron receives a sufficient stimulus, a rapid change in membrane potential occurs. This event is characterized by the influx of sodium ions, leading to depolarization. Following this, potassium ions exit, restoring the original membrane potential. This characteristic makes action potentials an efficient means of transmitting information along neurons.
Their benefit lies in speed; action potentials propagate quickly, allowing for real-time communication across networks. This feature is vital for reflex actions and rapid responses in sensory systems, ensuring organisms can react promptly to environmental stimuli.
However, there are disadvantages. The all-or-nothing nature of action potentials means that if a stimulus does not reach a certain threshold, no impulse occurs. This can lead to variability in communication efficiency across different types of neurons.
Neurotransmitters
Neurotransmitters are chemicals that neurons use to communicate with each other at synapses. The release of these substances from an axon terminal into the synaptic cleft allows signals to traverse the gap between neurons. Neurotransmitters can be classified into categories, such as excitatory and inhibitory, depending on their effects on the post-synaptic neuron.
A key characteristic of neurotransmitters is their specificity. Different neurotransmitters interact with specific receptors, which can either enhance or dampen neural signals. This aspect makes neurotransmitters crucial for modulating a broad array of neural functions, including mood regulation and motor control.
One unique feature of neurotransmitters is their ability to lead to varied responses even in the same neuron. This adaptability supports complex behaviors and cognitive processes. However, the improper balance or malfunctioning of specific neurotransmitters can lead to disorders, such as anxiety and depression. Therefore, understanding their roles is vital for both neuroscience and therapeutic interventions.
Network Activity and Patterns
The activity of neural networks is not random; it follows patterns that reflect underlying processes. Neural patterns emerge from the synchronous firing of neurons, which often correlate with specific functions or behaviors. For example, certain rhythmic patterns are associated with states such as sleep or alertness.
Such patterns reveal insights into both normal brain function and pathological states. By examining these activities through advanced imaging techniques, researchers can monitor how different regions communicate and adapt over time. This understanding has important implications for fields like neuropsychology and artificial intelligence.
Neuroplasticity: The Brain's Adaptability
Neuroplasticity is a crucial aspect of the human neural network that underscores the brain's capacity to reorganize itself. This adaptability is essential for both recovery from injury and the acquisition of new skills. The concept of neuroplasticity is not just a theoretical idea; it has practical implications in various fields, including educational practices and rehabilitation therapies.
This section will delve into the mechanisms that facilitate neuroplasticity and its significant implications for learning and memory. Understanding these processes is vital for advancing our knowledge in neuroscience and optimizing methods for education and rehabilitation.
Mechanisms of Neuroplasticity
There are several mechanisms that contribute to the brain's ability to adapt through neuroplasticity. One of the primary mechanisms is long-term potentiation (LTP). LTP occurs when synaptic connections between neurons strengthen due to repetitive stimulation. This leads to a more efficient communication pathway within the neural network. Conversely, long-term depression (LTD) weakens synaptic connections, allowing the brain to prune unnecessary pathways, thereby optimizing its performance.
Another important aspect of neuroplasticity is the growth of new neurons, known as neurogenesis. This process primarily happens in the hippocampus, a region associated with memory and learning. Factors such as exercise, enriched environments, and cognitive challenges are known to promote neurogenesis, illustrating how lifestyle can impact brain health.
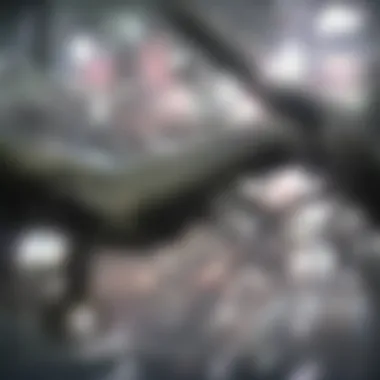
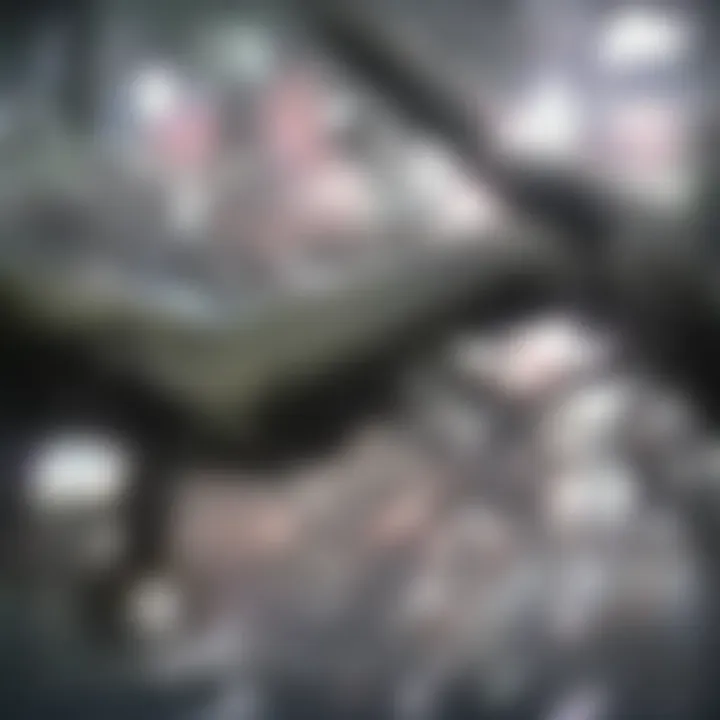
"Neuroplasticity offers an opportunity for recovery and improvement that was once thought impossible in adults."
Moreover, myelination plays a role in neuroplastic changes. Myelin is the protective sheath around nerve fibers, which enhances the speed and efficiency of signal transmission. When learning new information, myelination can be increased, making neural pathways more efficientβsomething profoundly significant in educational environments.
Implications for Learning and Memory
Neuroplasticity has significant implications for learning and memory. The ability of the brain to form new neural connections is particularly essential for lifelong learning. Educators can leverage principles of neuroplasticity to design curricula that promote engagement and adaptive learning strategies.
A key insight is that repeated practice strengthens the synapses involved in specific tasks. This understanding leads to the implementation of spaced repetition and varied practice in teaching methodologies. Both techniques effectively utilize the nature of neuroplasticity to reinforce memory retention.
In addition, neuroplasticity affects how memories are formed and retained. For individuals with learning disabilities or memory disorders, therapies aimed at enhancing neuroplasticity can foster recovery. This can include cognitive-behavioral techniques and music therapy, which have shown promise in stimulating brain regions linked to memory.
Ultimately, acknowledging the brain's adaptability can lead to innovative approaches in education and treatment paradigms. Understanding neuroplasticity not only enriches our grasp of human cognition but also equips us to better support those facing cognitive challenges.
The Role of Human Neural Networks in Cognitive Processes
Understanding the role of human neural networks in cognitive processes is essential for grasping how we perceive the world, make decisions, and regulate our emotions. Human cognition arises from complex interactions within neural networks, made possible by the intricate structure and function of neurons. Every thought, feeling, and action we perform is deeply rooted in these neural frameworks. This section will explore three critical aspects of cognitive processes: perception, decision-making, and the interplay of emotion and behavior.
Perception
Perception serves as the gateway to understanding the environment around us. Our sensory neurons collect stimuli from the external world and transmit this information to the brain. Here, neural networks engage in processing and interpretation, transforming raw data into meaningful experiences. This process is not merely passive; rather, it involves active constructions of reality influenced by past experiences, context, and cognitive biases.
For instance, the visual system relies on various types of neurons to detect edges, colors, and motion. The integration of these sensory inputs occurs in a dynamic fashion within areas like the visual cortex, where associative networks create congruent representations of objects. Furthermore, perception is influenced by cognitive states, which can modify how we interpret incoming information. The following elements highlight significant facts about the role of perception:
- Multisensory integration: The brain uses information from different senses to create a cohesive perception.
- Top-down processing: Prior knowledge and expectations shape how we perceive stimuli.
- Adaptability: Perceptual systems remain flexible, allowing adjustments based on learned experiences.
"The functioning of perception highlights the brainβs ability to adapt and reshape how reality is perceived, ultimately guiding behavior."
Decision Making
Decision making encompasses selecting one option among multiple choices based on available information and anticipated outcomes. Neural networks contribute to this process by utilizing memory, emotion, and rationality. The prefrontal cortex plays a pivotal role, integrating inputs from various regions, including those responsible for emotional processing.
Neuroscience has shown that decision-making is not purely logical. Emotions significantly impact judgments and choices. For example, elevated fear levels often lead to conservative decisions, while positivity can encourage risk-taking behavior. Neural connections involved in this aspect underpin the complexity of decision making:
- The reward system: Engages when evaluating potential benefits during decision-making.
- Conflict resolution: Neural circuits help manage competing choices and outcomes.
- Predictive modeling: The brain forecasts future results based on accumulated knowledge, leading to informed choices.
These elements weave together to facilitate the multi-faceted nature of decision-making rooted in neural dynamics.
Emotion and Behavior
Emotion and behavior are intricately linked processes, grounded in the neural substrate. The limbic system, including structures like the amygdala and hippocampus, plays a vital role in emotional processing. Neural networks involved in emotions govern responses, influencing actions and social interactions. Understanding this interconnectedness reveals how emotional states impact behaviors, shaping individual experiences and social relationships.
Several factors contribute to this relationship:
- Emotional regulation: Neural mechanisms assist in managing emotional reactions and their implications.
- Social cues: Perception of othersβ emotions can trigger empathy or defensive behaviors through neural pathways.
- Learning from experience: Previous emotional responses inform future behaviors, creating habitual patterns.
Comparative Analysis: Human Neural Networks and AI
The examination of human neural networks in relation to artificial intelligence (AI) explores parallels and divergences that offer valuable insights into both fields. Understanding this comparative framework is crucial for both enhancing our grasp of human cognition and improving AI systems. This analysis leads to critical considerations about how closely AI can mimic human processes and what that means for future technological advancements.
Artificial Neural Networks
Artificial neural networks (ANNs) are computational models inspired by the way biological neural networks operate. In ANNs, the fundamental building block is the node or artificial neuron, which receives inputs, processes them, and produces outputs. These networks are characterized by layers:
- Input Layer: This is where initial data is received.
- Hidden Layers: These layers perform various transformations and feature extractions on the input data.
- Output Layer: This generates the final outcome based on preceding computations.
The architecture of ANNs enables them to recognize patterns, make decisions, and learn from experience through training. This training usually involves using a dataset to adjust connection weights. However, while ANNs can replicate certain cognitive functions, their operations often lack the complexity and adaptability of human neural networks.
Moreover, ANNs can struggle with tasks requiring contextual awareness and emotional intelligence. Research shows that humans rely not just on objective data, but also on social context and intrinsic understanding of emotions when making decisions. Thus, while ANNs excel in statistical tasks and data-heavy applications, they fall short compared to human neural networks in nuanced tasks like reasoning, empathy, and ethical decision-making.
Learning Algorithms
The learning algorithms employed in both human neural networks and artificial neural networks exhibit fundamental differences, especially in their adaptability and efficiency. In biological systems, learning is an ongoing and highly dynamic process. Humans learn through experience, reflection, and interaction, with neural pathways continuously being refined based on new information.
In contrast, the learning algorithms in ANNs, such as backpropagation and reinforcement learning, require extensive amounts of data for effective training.
- Backpropagation: This involves a forward pass for predictions, followed by a backward pass to minimize error by adjusting weights.
- Reinforcement Learning: This technique trains models by rewarding desired actions, mimicking certain aspects of human learning through trial and error.
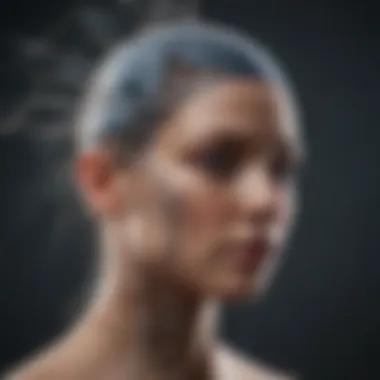
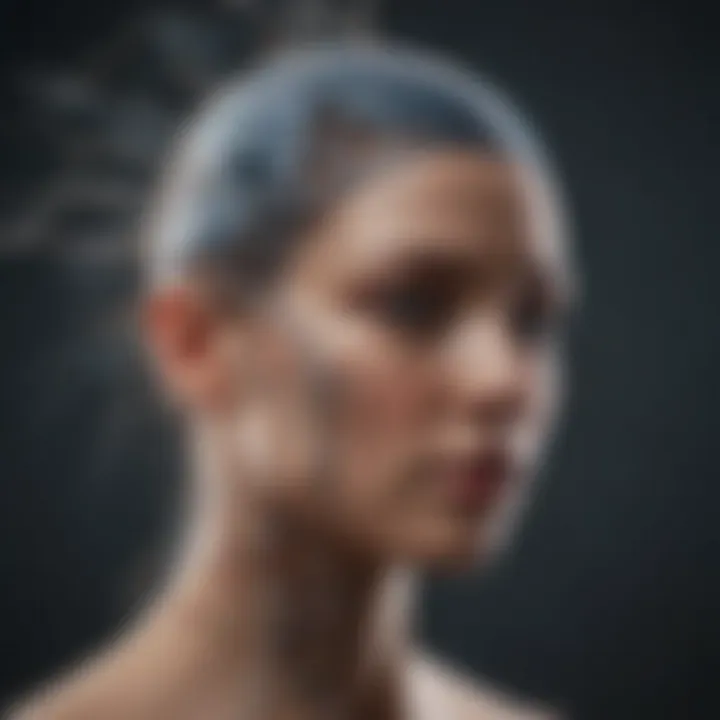
While these algorithms have made strides in applications ranging from image recognition to natural language processing, they necessitate a level of computation and resource allocation that is often not sustainable. Another significant difference is the ability of human neural networks to generalize knowledge swiftly, leveraging a few examples to understand broader concepts. ANNs typically require vast quantities of data to achieve comparable generalization, which can be a limitation in real-world applications where data may be scarce.
"While artificial neural networks can model specific functions, the overarching architecture and adaptability of human neural networks remain superior."
In summary, the comparative analysis between human neural networks and artificial intelligence presents a framework for understanding both the limitations and potentials of current AI technologies. This exploration is vital as we progress in both neuroscience and AI, shaping optimal strategies for future research and development in these intertwined domains.
Neuroscience Research: Latest Findings
Neuroscience research has seen remarkable evolution in recent years. Understanding the human neural network is crucial for several reasons. It provides deep insights into how our brain functions at various levels. Moreover, it enriches our knowledge about diseases affecting neurological systems. The advancements in neuroscience research contribute significantly to our grasp of cognitive processes and emotional regulation. This section focuses on two key areas: recent advances in neuroimaging techniques and insights gained from neurodegenerative disorders.
Recent Advances in Neuroimaging
Neuroimaging is a field that permits non-invasive observation of the brain's structure and function. Recent advances have made neuroimaging more precise and informative. Technologies like functional magnetic resonance imaging (fMRI) and positron emission tomography (PET) allow researchers to see brain activity in real-time. They provide vital data on blood flow and metabolic processes in the brain. This has significant implications for understanding various mental health disorders, including depression and anxiety.
- Enhanced Spatial Resolution: Modern neuroimaging offers better resolution, presenting clearer images of brain structures.
- Real-time Functioning: It enables the observation of brain operations while subjects engage in specific tasks.
- Cross-sectional Studies: Researchers can compare the neural activity of different populations, leading to breakthroughs in mental health understanding.
The application of neuroimaging extends beyond research. It influences clinical practices too. It helps in diagnosing conditions accurately and tailoring treatments based on individual patient needs.
Insights from Neurodegenerative Disorders
Neurodegenerative disorders present complex challenges, yet they also offer invaluable insights into the human brain. Conditions such as Alzheimer's disease and Parkinson's disease illustrate how neural networks can break down. Studying these disorders uncovers patterns of neural dysfunction.
Several important findings have emerged from research on neurodegenerative diseases:
- Biomarkers: Identifying specific neurological biomarkers helps in early diagnosis. This is crucial as early treatment can improve outcomes.
- Genetic Factors: Researchers are exploring genetic links in conditions like Huntington's disease, shedding light on inherited risks for neurological disorders.
- Connection Patterns: Understanding how connections between neurons deteriorate aids in developing potential therapies.
"The study of neurodegenerative disorders not only reveals the fragility of our brain's networks but also highlights the need for proactive care and innovative treatment methods."
As research progresses, there is hope for more effective interventions for these disorders, improving patient quality of life. Overall, recent findings in neuroscience research enhance our understanding, offering frameworks for how we can support brain health and neurological wellbeing in society.
Ethical Considerations in Neural Research
Understanding the ethical considerations in neural research is vital for advancing knowledge while safeguarding human dignity and rights. As we explore the intricacies of human neural networks, we encounter moral dilemmas that may arise from experimentation. This section delves into the implications of neural experimentation, the potential benefits to society, and the safeguarding of individual privacy.
Implications of Neural Experimentation
Neural experimentation serves as a gateway to unraveling complex neurological disorders and enhancing cognitive capabilities. Researchers can potentially make breakthroughs in treating diseases like Alzheimer's and Parkinson's. However, the methods of experimentation must be scrutinized carefully. Ethical issues arise when the boundaries of consent and manipulation are blurred. For instance, using invasive techniques can lead to significant risks for participants.
Moreover, there is a fine balance between pushing the limits of knowledge and respecting the autonomy of individuals. It could be beneficial to establish a strict regulatory framework that governs methodologies in neural research. This framework should encompass informed consent and the right to withdraw at any time. Researchers must weigh the knowledge gained against potential risks to participants, ensuring that humanity remains at the forefront of advancing science.
Privacy Concerns in Neurotechnologies
The integration of neurotechnologies into everyday life raises significant privacy concerns. With the increasing use of brain-computer interfaces and neuroimaging technologies, data generated about a personβs neural activity could expose personal thoughts and feelings. Such information, if misused, has the potential to violate privacy rights and lead to discrimination based on neurological data.
Researchers and developers must prioritize data protection and ethical standards. Individuals need transparent information on how their neural data will be used, stored, and protected. Creating robust privacy policies and implementing strict security measures are crucial steps in safeguarding sensitive information.
"Ethical neural research prioritizes informed consent, data privacy, and respect for autonomy."
Addressing these ethical considerations is not just a responsibility of researchers but also a societal imperative. Engaging in open dialogues about the ethical dimensions of neural research can lead to more responsible practices and a greater public understanding of the implications of their work. Moving forward, a comprehensive ethical approach will be essential to foster trust in neuroscience advancements.
Culmination: The Future of Human Neural Network Research
The study of human neural networks is crucial because it underscores our understanding of human cognition and behavior. As advancements in neuroscience continue to unfold, the implications of these discoveries reach far beyond the realm of biology. Exploring neural networks allows us to examine how humans process information, learn, and adapt.
The intricate architecture of neural connections offers insights into how our brains function. This knowledge not only informs education and psychological health but also influences technological development. As we better understand the brain's mechanisms, we can develop more effective and targeted interventions for neurological disorders.
Moreover, ethical considerations will play a significant role moving forward. As researchers strive to uncover more about the human mind, they must navigate the moral implications of their discoveries. This involves ensuring that advancements in neurotechnology respect individual privacy and autonomy.
Impacts on Technology and Society
The influence of human neural network research is already evident in both technology and societal structures. As we learn more about our neural circuits, developers in artificial intelligence can create systems that mimic human cognitive functions. This impacts various fields, such as education, where tailored learning experiences are being designed to align with human learning patterns.
Some key impacts include:
- Enhanced Learning Technologies: Personalized education tools that improve learning outcomes by adapting to individual cognitive styles.
- Mental Health Interventions: Developing neurotechnologies that can facilitate therapy or enable early diagnosis of mental health disorders.
- Advancements in AI: Creating more sophisticated algorithms that improve machine learning through insights gained from human cognition.
"Understanding our neural systems allows for significant advancements in technology that mirror human intelligence, creating efficiencies and possibilities that were once thought impossible."
Continuing the Journey of Discovery
As we embark on this ongoing journey of discovery, there are several aspects that merit attention:
- Interdisciplinary Collaboration: Collaboration among neuroscientists, psychologists, computer scientists, and ethicists will be vital. This approach can yield comprehensive insights that enhance our understanding of the human brain and its applications.
- Technological Integration: Integrating neuroimaging and data analytics with research will allow for deeper insights into neural functions. These technologies help visualize and interpret complex neural processes that are otherwise difficult to study.
- Addressing Ethical Challenges: The future of neural network research must prioritize ethical considerations. Balancing innovation with moral responsibility will ensure that research benefits society without compromising individual rights.
The future is one of potential. Continued investigation into human neural networks is set to yield transformative outcomes in both science and society, paving the way for new knowledge and fostering a more nuanced understanding of both human and artificial intelligence.