Understanding the Higgs Boson: A Comprehensive Overview
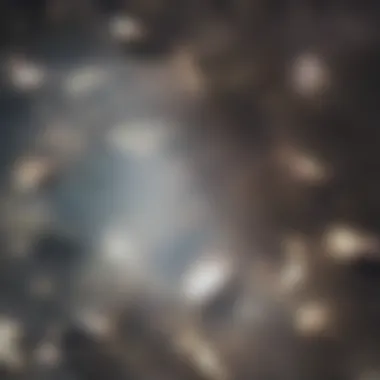
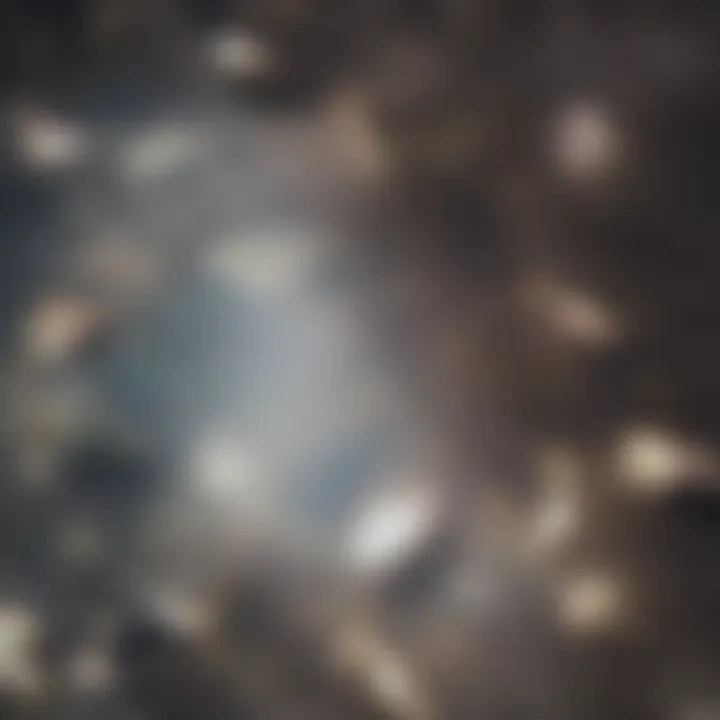
Intro
The Higgs boson is central to the Standard Model of particle physics. It is a particle that is responsible for giving mass to other elementary particles. The discovery of the Higgs boson has far-reaching implications for modern physics. Theoretical predictions, experimental challenges, and subsequent discoveries have built a profound understanding of this elusive particle.
In this article, we will explore its theoretical background, the mechanisms surrounding its existence, and the ongoing research that continues to unfold in this field. This narrative aims to bridge intricate concepts with clear explanations, catering to students, researchers, educators, and professionals eager to deepen their knowledge on this pivotal subject.
Key Research Findings
Overview of Recent Discoveries
The journey to confirming the existence of the Higgs boson culminated at CERNโs Large Hadron Collider in 2012. Experiments revealed strong evidence of a particle consistent with the predicted properties of the Higgs boson. The significance of this discovery cannot be understated, as it validates the mechanism proposed in the Standard Model for mass generation. Various research studies since then have focused on understanding its properties and interactions.
- Mass Measurement: The Higgs bosonโs mass was measured to be approximately 125 GeV.
- Couplings: Ongoing experiments assess how strongly the Higgs boson couples to other particles, like leptons and quarks.
- Decay Channels: Investigations into its decay into different particles provide insight into its behavior.
Researchers continually refine techniques to enhance data collection and analysis, which improves the discernment of subtle signals from potential new physics beyond the Standard Model.
Significance of Findings in the Field
The implications of confirming the Higgs boson extend beyond mere validation of theoretical frameworks. Its discovery prompts further questions about fundamental forces and particles. Understanding the Higgs boson contributes to:
- Unifying Forces: The search for a deeper connection among fundamental forces.
- Beyond Standard Model Physics: Identifying phenomena that current theories do not explain would inform new theories and models.
- Cosmology: Understanding the evolution of the universe and mass distribution informs complex cosmic questions.
"The Higgs boson discovery marks a milestone in particle physics, opening new avenues for research and discovery."
Breakdown of Complex Concepts
Simplification of Advanced Theories
The Higgs mechanism is often considered complex. Yet, at its core, it can be broken down into simpler components. The Higgs field permeates the universe, and its interaction with particles bestows them mass.
- Field vs. Particle: The Higgs field exists everywhere and gives rise to the particle when excited.
- Mass Origin: Different particles encounter varying strengths of the Higgs field, resulting in different masses.
This democratization of mass generation is fundamental to understanding fundamental particles.
Visual Aids and Infographics
Several visual aids effectively illustrate the complexities of the Higgs mechanism and its role in particle physics. These include:
- Diagrams of particle interactions with the Higgs field.
- Infographics depicting the Higgs boson production processes at the LHC.
- Graphs showcasing decay channels and their associated probabilities.
These tools enable a more tangible grasp of how the Higgs boson integrates into the framework of known physics.
This foundational understanding bridges theoretical and experimental physics and emphasizes the ongoing nature of research in this critical area. Further investigations into the Higgs boson promise to enhance our understanding of the universe and its fundamental laws.
Prolusion to Particle Physics
Particle physics is an essential field that explores the most fundamental components of matter and the forces that govern their interactions. Understanding this domain is crucial for unraveling the complexities of the universe. The topic of particle physics not only establishes a foundational framework for scientific inquiry but also ties into various branches of physics, cosmology, and even philosophy. It serves as the backbone for significant discoveries, including the Higgs boson, which is the focus of this article.
In this section, we will examine the basic concepts that underpin particle physics and provide a comprehensive overview of the Standard Model. This framework has been pivotal for advancements in our comprehension of mass, energy, and the interactions between subatomic particles. By delving into the principles and theories governing particle physics, one can appreciate the significance of the Higgs boson and its role in shaping modern physics.
The study of particle physics allows scientists to probe deeper into the fabric of reality, illuminating the creation and evolution of the universe itself.
Basic Concepts of Particle Physics
At the core of particle physics lies the concept of elementary particles, which are the building blocks of matter. These particles include quarks, leptons, and bosons, each exhibiting unique properties. Quarks combine to form protons and neutrons, while leptons include electrons and neutrinos. Bosons, on the other hand, are particles that carry forces, such as photons and the Higgs boson.
The interactions between these particles are governed by four fundamental forces:
- Gravitational Force: The weakest force, influencing the macroscopic structure of the universe.
- Electromagnetic Force: Responsible for electric and magnetic interactions, governing chemistry and atomic structure.
- Weak Nuclear Force: Facilitates processes like beta decay, affecting particle interactions on a subatomic level.
- Strong Nuclear Force: The most potent force, binding quarks together within protons and neutrons.
Understanding the properties and behaviors of these particles and forces lays the groundwork for the broader theories that define particle physics. This knowledge is essential for contextualizing the Higgs boson within the framework of the Standard Model.
The Standard Model Explained
The Standard Model of particle physics is a theoretical construct that describes the known particles and their interactions through three of the four fundamental forces. It provides a detailed account of how particles like the quarks and leptons function, while also integrating the bosons as force carriers.
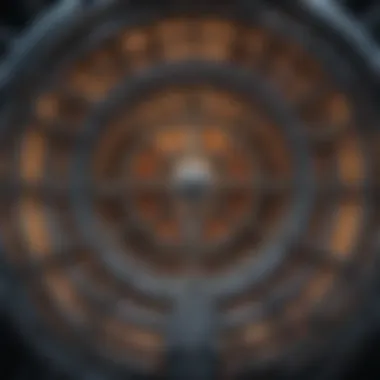
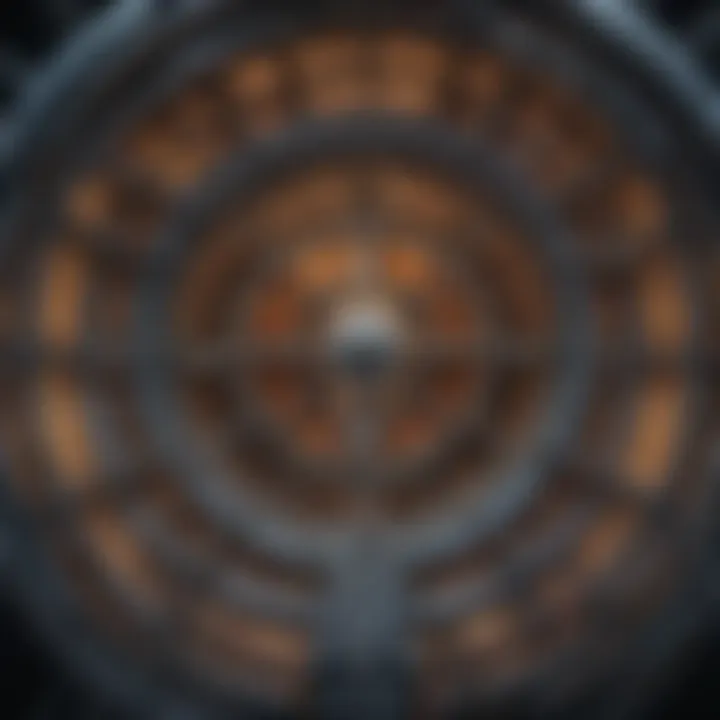
Key features of the Standard Model include:
- Particle Classification: It categorizes fundamental particles into fermions (matter particles) and bosons (force carriers).
- Symmetry Principles: The model is grounded in gauge symmetries, which describe how particles interact and transform.
- Higgs Mechanism: This is a critical component of the model that explains how particles acquire mass through the Higgs field, a concept central to our understanding of the universe's composition.
The successful predictions made by the Standard Model and its experimental verifications, including the discovery of the Higgs boson, are a testament to its robustness.
While the Standard Model effectively illustrates the behaviors of particles and forces, it does not address all phenomena. Questions remain about dark matter, dark energy, and gravity, which geometry and topology might resolve on a deeper level. The ongoing research seeks to expand our knowledge beyond current theories, continuing the pursuit of understanding in particle physics.
Theoretical Foundations of the Higgs Boson
The theoretical foundations of the Higgs boson are essential for understanding its significance in the realm of particle physics. This section will explore early predictions, the development of the Higgs mechanism, and the implications that arise from these theoretical advancements. The Higgs boson is not merely a particle; it is a cornerstone that illustrates how particles acquire mass and form the very fabric of the universe. Understanding these foundational theories reveals much about the current state of physics and guides future inquiries into the nature of matter and the cosmos.
Early Theoretical Predictions
The journey to the Higgs boson began long before it was discovered experimentally. In the 1960s, several physicists, including Peter Higgs, proposed theoretical frameworks that required additional particles to explain the behavior of fundamental forces. One pivotal concept introduced was the idea of symmetry breaking in gauge theories.
The notion was that elementary particles should be massless in their fundamental states, but interactions with a pervasive field would provide them with mass. This idea was radical yet alluring because it connected elegantly with existing theories of particle physics.
Key aspects of these early predictions include:
- Spontaneous Symmetry Breaking: Particle masses arise when the symmetric state of a field changes, akin to a phase transition.
- The Higgs Field: An omnipresent field that interacts with particles, leading them to acquire mass depending on their coupling with the field.
- Mass Generation: A theoretical mechanism that imparts mass to elementary particles without violating gauge invariance.
Higgs and others postulated that the Higgs boson would be a manifestation of this field, a critical evidence for the validity of their models.
Higgs Mechanism and Mass Generation
The Higgs mechanism operates on a principle that turns theoretical constructs into tangible outcomes. Central to this mechanism is the process of mass generation, which is vital for understanding the cohesion of matter. The Higgs field permeates the universe and interacts with particles differently based on their inherent properties.
The relationship between the Higgs field and particle mass can be summarized as follows:
- Coupling Strength: Particles with stronger interactions with the Higgs field gain more mass, while those with weaker interactions remain lighter.
- Gauge Symmetry: The underlying symmetry of the system dictates how particles interact with the Higgs field, which is crucial for maintaining the principles of the Standard Model.
The process is mathematically complex yet conceptually straightforward. The predictions about the Higgs boson highlight the interaction between the field and the types of elementary particles. In this sense, the Higgs boson acts as the visible echo of the mechanisms at play.
"The Higgs mechanism provides a profound understanding of the mass of elementary particles, connecting deep theory with experimental evidence."
The Large Hadron Collider
The Large Hadron Collider (LHC) acts as a cornerstone in the quest to understand fundamental particles and forces in the universe. It has played a crucial role in particle physics and specifically in the study of the Higgs boson. The significance of the LHC goes beyond its sheer size and complexity; it embodies a collective effort of the global scientific community to probe the mysteries of the subatomic world.
The LHC, located at CERN near Geneva, Switzerland, opened its doors in 2008. It is the world's largest and most powerful particle accelerator. Charged particles, primarily protons, are propelled to near light speed and directed into head-on collisions. These interactions create conditions similar to those just after the Big Bang, allowing physicists to examine the resulting particles and phenomena.
Design and Functionality
The design of the LHC is both intricate and innovative. Spanning approximately 27 kilometers in circumference, it utilizes over 1,200 superconducting magnets to steer the particles around the ring. The acceleration process occurs in two main stages: pre-acceleration and main acceleration.
In the pre-acceleration phase, particles are initially accelerated through smaller accelerators before entering the LHC. Once inside, these protons are accelerated to energies exceeding 7 TeV (terelectronvolts) for collisions.
Moreover, the LHC is equipped with multiple detectors, including ATLAS and CMS, which capture and record the debris from particle collisions. Each of these detectors is engineered to observe different types of particles and interactions. The data from these experiments is then analyzed to identify rare events, including the potential signatures of the Higgs boson.
Key functionalities of the LHC include:
- High-energy collisions: These collisions offer insights into the properties of fundamental particles.
- Data collection: The LHC generates massive amounts of data daily, crucial for discovering new particles.
- Multidisciplinary collaboration: Scientists from various fields work together, enhancing the research scope.
Experiments Leading to Higgs Discovery
Several experiments conducted at the LHC were pivotal in confirming the existence of the Higgs boson. Among these, the most notable were carried out by the ATLAS and CMS collaborations. Both teams experimented with the data produced from collisions and identified a bump in their data at approximately 125 GeV (giga-electronvolts), suggesting the presence of a new particle.
"The discovery of a new particle consistent with the Higgs boson was a monumental moment in our understanding of particle physics."
The experiments involved:
- Collecting collision data: Nearly a billion collisions were studied to find rare events.
- Analyzing decay patterns: The Higgs boson can decay into various particles, making detailed study necessary. The most common decay modes included pairs of photons, Z bosons, and W bosons.
- Statistical analysis: Advanced statistical methods were employed to differentiate between background noise and meaningful signals.
In July 2012, CERN announced the discovery of a particle consistent with the Higgs boson, around the anticipated mass, which confirmed decades of theoretical predictions. The analysis of this particle's properties continues as scientists refine their measures and deepen the exploration of its implications.
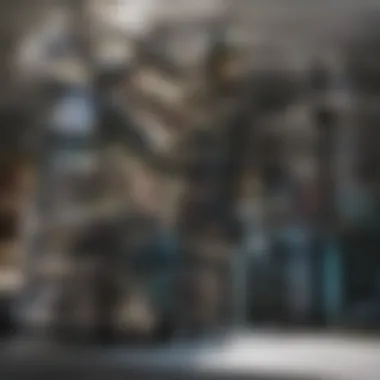
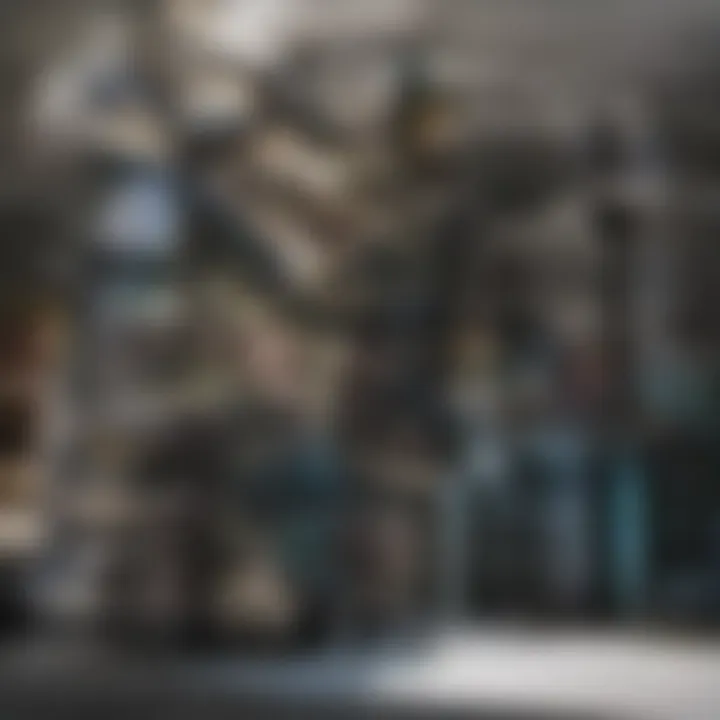
The contributions of the LHC transcend the discovery of the Higgs boson; they affect cosmology, the understanding of dark matter, and theories beyond the Standard Model. As research progresses, the LHC remains a pivotal institution for understanding our universe.
Discovery of the Higgs Boson
The discovery of the Higgs boson marks a pivotal moment in the field of particle physics. It confirms a core component of the Standard Model, specifically the Higgs mechanism, which explains how particles acquire mass. This section will explore the key experiments that led to this groundbreaking finding and examine the implications of the announcement for the scientific community and society at large.
Key Experiments and Findings
The journey to discover the Higgs boson involved several key experiments conducted primarily at the Large Hadron Collider in CERN. The LHC, being the world's largest and most powerful particle accelerator, plays a critical role in high-energy physics research.
- ATLAS and CMS Collaborations: These two large detectors at the LHC focused on analyzing collision data. The ATLAS experiment searched for signs of the Higgs boson in various decay channels, while CMS used similar methodologies. Both collaborations aimed to capture the elusive particle's existence through indirect methods.
- Collision Data Analysis: Scientists collected and analyzed vast amounts of data from proton-proton collisions. The data showed a significant excess of events consistent with the Higgs boson signature, particularly in the mass range of about 125 GeV/cยฒ.
- Statistical Significance: Using sophisticated statistical techniques, the teams assessed the likelihood that the observed events were not mere coincidences. A statistical significance of 5 sigma was achieved, which is considered the gold standard for claiming a discovery in particle physics.
- Final Results: On July 4, 2012, both the ATLAS and CMS collaborations announced their independent findings, providing strong evidence for the existence of a new particle consistent with the predicted properties of the Higgs boson. This momentous occasion marked the culmination of decades of theoretical and experimental research in particle physics.
"The discovery of the Higgs boson is a triumph of human ingenuity and collaboration, a testament to the relentless pursuit of knowledge in understanding our universe."
Announcement and Its Impact
The announcement of the Higgs boson's discovery generated immense excitement and intrigue within the scientific community and beyond. It did not just validate previous theoretical work but raised several important points.
- Validation of the Standard Model: The observation of the Higgs boson solidified the Standard Model as a robust framework for understanding particle interactions and fundamental forces.
- Stimulating Further Research: This discovery opened new avenues for research. Scientists began exploring the boson's properties, such as its coupling to other particles and its role in the early universe.
- Funding and Public Interest: The announcement attracted significant media attention, resulting in increased funding opportunities for particle physics research. Public interest surged, highlighting the importance of fundamental science in addressing profound questions about existence and the universe.
- Philosophical Discussions: Beyond the technical aspects, this discovery led to philosophical discussions about the nature of reality and the limits of human knowledge. The Higgs boson, as a fundamental entity, raises questions about what we know and what lies beyond the observable universe.
In summary, the discovery of the Higgs boson is not only a scientific milestone but also a catalyst for ongoing inquiry into the nature of the universe. The experiments leading to this discovery have profoundly impacted both theoretical and experimental physics.
Current Research and Implications
The study of the Higgs boson continues to be a focal point in modern physics. The implications of its discovery are vast, impacting theories of mass and the fundamental forces that shape our universe. Current research is essential for several reasons, chiefly to understand the nuances of the Standard Model, probe for new particles, and investigate phenomena beyond established theories.
Beyond the Higgs Boson
Current investigations extend well beyond the Higgs boson itself. Researchers are interested in how this particle fits into the broader context of particle physics. Some of the specific areas of focus include:
- Supersymmetry: A proposed extension of the Standard Model, hypothesizing a mirrored counterpart to each known particle.
- Dark Matter: Understanding if there are connections between the Higgs boson and the elusive dark matter that makes up a significant part of the universeโs mass.
- Neutrino Mass: Investigating the role of the Higgs mechanism in explaining the small masses of neutrinos.
Research efforts mainly concentrate on enhancing particle detectors and improving data analysis techniques. Scientists constantly strive to refine knowledge and test predictions derived from theoretical frameworks. These studies help reveal whether the Higgs boson holds clues to a deeper understanding of the universe.
Higgs Boson in Cosmology and Beyond
The implications of the Higgs boson extend into the realm of cosmology. It plays a pivotal role in accounting for the mass distribution observed in the cosmos. Some key points include:
- Cosmic Inflation: The Higgs field might have influenced the rapid expansion of the universe just after the Big Bang.
- Formation of Structures: Understanding mass generation helps explain how galaxies and cosmic structures formed over time.
- Accelerating Universe: Exploration is ongoing to understand the interplay between the Higgs boson and dark energy, which drives the universe's acceleration.
These discussions lead to significant questions in physics, such as the fate of the universe and how different forces interlink.
"The discovery of the Higgs boson is one of the greatest achievements in particle physics, but it serves only as a stepping stone toward broader understanding and discovery."
As research progresses, physicists anticipate not just answers, but more questions revealing the complexity of the universe. The Higgs boson might be just the beginning of a more intricate tapestry in theoretical physics.
Challenges in Higgs Boson Research
The research surrounding the Higgs boson is not without its obstacles. Recognizing these challenges is crucial, as they can impact the future trajectory of particle physics. Addressing both technical issues and theoretical uncertainties provides a roadmap for understanding the ongoing complexities in the exploration of the Higgs boson. This section discusses the significant technical challenges faced at the Large Hadron Collider (LHC) and the open questions that continue to perplex physicists.
Technical Challenges at the LHC
The Large Hadron Collider, located at CERN, is the most powerful particle accelerator in the world. Its primary aim is to investigate the fundamental forces and particles that make up our universe. However, operating such a colossal machine comes with numerous technical challenges. Key areas of concern include:
- Data Volume: The LHC generates an enormous amount of data with each collision, often reaching petabytes on a daily basis. This presents significant challenges in data storage, processing, and analysis. The sheer volume requires robust computing resources and advanced algorithms that can efficiently sift through data for valuable insights.
- Detector Precision: The experiments conducted at the LHC utilize complex detectors like ATLAS and CMS to capture collision events. Achieving high precision in these measurements is vital. Any misalignment or malfunction in these detectors can lead to inaccurate results, complicating the task of confirming the properties of the Higgs boson.
- Experimental Design: Designing experiments to maximize the likelihood of observing rare phenomena, such as the Higgs boson production, is inherently difficult. The experimental setups must be finely tuned to distinguish the Higgs signature from the vast background events occurring in high-energy collisions.
- Theoretical Models: Theoretical predictions need to align with experimental observations. Some models may predict various scenarios regarding the Higgs boson properties, which introduces ambiguity in obtaining a definitive understanding. Resolving this requires continuous refinement of both theoretical and experimental techniques.
Overcoming these technical challenges is crucial for the sustained success and credibility of Higgs boson research.
Open Questions in Particle Physics
Despite the successful discovery of the Higgs boson, numerous open questions remain in the field of particle physics. Some of the fundamental inquiries include:
- Dark Matter: What is the nature of dark matter? Its composition is unknown and it does not interact with ordinary matter in ways that are easily observable. Understanding its potential relationship to the Higgs boson could illuminate many aspects of the universe.
- Neutrino Mass: Neutrinos are fundamental particles that were once believed to be massless. Their discovery to have mass raises questions about the mechanism behind it. Does the Higgs mechanism apply here?
- Hierarchy Problem: Why is the Higgs boson mass so much lighter than the Planck mass? This discrepancy leads to deep theoretical issues about the stability of the standard model and the fundamental forces.
"The quest to answer these questions pushes the boundaries of current understanding and shines a light on the pathways for future research."
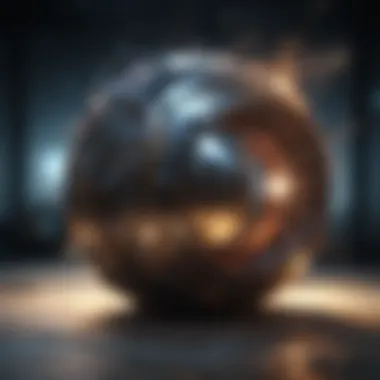
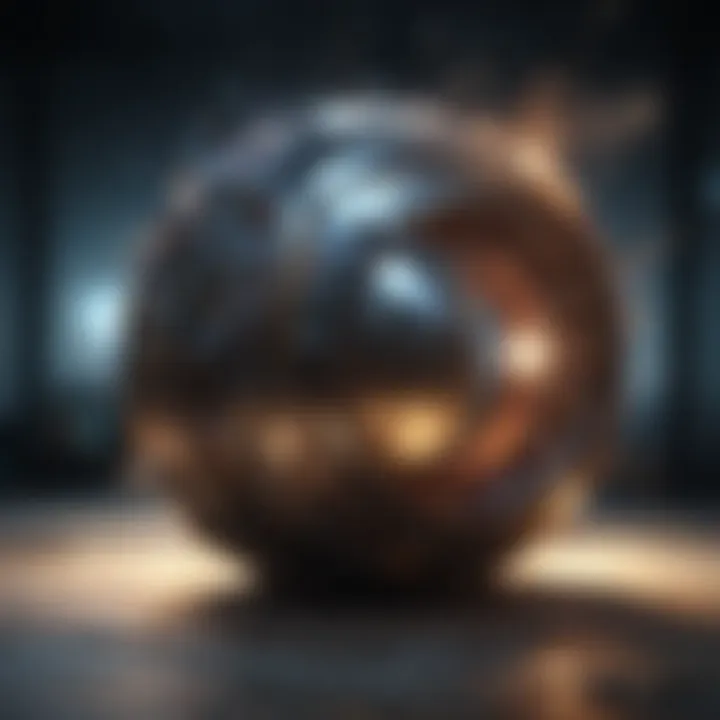
Understanding these challenges, both technical and theoretical, is essential for students, researchers, educators, and professionals engaged in this field. Future advancements in Higgs boson research will depend on addressing these questions and building a more coherent narrative within particle physics.
Philosophical and Ethical Considerations
The intersection of philosophy and ethics with particle physics, particularly concerning the Higgs boson, is a profound area that invites contemplation and critical thought. This section discusses the importance of such reflections. It delves into how scientific inquiry shapes our understanding of reality and the implications of research funding in driving scientific progress.
The Nature of Scientific Inquiry
Science relies fundamentally on inquiry. Inquiry into the fundamental nature of reality, including particles like the Higgs boson, raises significant philosophical questions. These questions not only probe the mechanisms of the universe but also examine the underlying motivations and methodologies of scientific practice.
In particle physics, the pursuit of understanding how elementary particles interact, exist, and derive mass leads to a thought-provoking exploration of what constitutes knowledge. As scientists formulate hypotheses and conduct experiments, they engage with the very fabric of existence. This engagement often challenges previous notions and requires a willingness to alter oneโs perspective.
Furthermore, scientific inquiry is intrinsically iterative. Each discovery, including that of the Higgs boson, is built upon prior knowledge. The process involves questioning established theories while simultaneously supporting or refuting them through empirical evidence. The philosophical implications extend to how we perceive our place in the universe and what it means to seek knowledge. Science is not merely a catalog of facts; it is a dynamic, evolving conversation about understanding reality.
The Role of Funding in Physics Research
Funding plays a critical role in the advancement of scientific research, including studies of the Higgs boson. The significant costs associated with particle physics experiments, particularly at facilities like the Large Hadron Collider, necessitate substantial financial backing. Understanding the ethical implications of funding sources is vital for ensuring that research objectives align with broader societal values.
The allocation of resources can influence which questions scientists pursue and how studies are designed. This often results in a dichotomy between commercially motivated research and open inquiry driven by the quest for knowledge. Ethical considerations arise when funding comes from sources with vested interests, which may impact objectivity.
Additionally, public funding for physics research reflects societal priorities. Decisions made by governments and institutions speak to what is deemed essential in scientific endeavor.
- Key considerations include:
- The balance between theoretical exploration and practical applications.
- The transparency of funding sources and their influence.
- The ethical questions surrounding the commercialization of research findings.
The health of scientific inquiry relies on rigorous ethical standards in funding practices, ensuring that diverse questions are explored without bias.
Future Directions in Higgs Research
The pursuit of knowledge about the Higgs boson continues with numerous inquiries and experiments aiming to extend our understanding of its properties and role in the universe. These future directions are crucial for both experimental physics and theoretical frameworks. Delving into the implications of the Higgs boson could yield new insights into fundamental questions about mass, the forces of nature, and the behavior of particles at both extremes of energy.
Next-Generation Particle Colliders
Next-generation particle colliders are central to advancing Higgs research. Current facilities, like the Large Hadron Collider at CERN, have made significant strides. However, they are approaching their energy limits. New colliders promise higher energies, which could reveal phenomena beyond the Standard Model. Projects such as the International Linear Collider (ILC) and the Compact Linear Collider (CLIC) are among the proposed facilities.
These colliders will not only allow for more precise measurements of the Higgs boson but could also enable the exploration of potential new particles and forces. As we probe deeper into the fundamental structure of matter, these advanced colliders stand as vital tools to test hypotheses about dark matter and other unanswered questions in particle physics.
- Higher energy levels will facilitate the discovery of rare processes involving the Higgs boson.
- Enhanced luminosity will improve the accuracy of Higgs decay studies.
"Next-generation colliders are not just instruments of discovery; they are gateways to understanding the very fabric of our universe."
Interdisciplinary Approaches
The complexity of Higgs boson research encourages interdisciplinary collaboration. Physicists can benefit from insights derived from fields like computer science, mathematics, and even philosophy. This cross-pollination of ideas is essential for tackling the multifaceted challenges presented by high-energy physics.
Researchers are utilizing advanced computational techniques, such as machine learning, to analyze vast amounts of data generated in particle collisions. This approach streamlines the identification of rare Higgs events and enhances the overall efficiency of experiments.
Moreover, the philosophical implications of our understanding of particles, mass, and the universe's origin guide researchers in few more general topics about existence and research methodology. Bridging gaps between these disciplines not only enriches our conceptual groundwork but also encourages a deeper questioning of our scientific practices.
By integrating various disciplines, we can unlock innovative methodologies that may drastically improve our comprehension of the Higgs boson and its repercussions in a broader cosmological context.
Ending
In the realm of particle physics, the Higgs boson stands out as a significant breakthrough. Its discovery not only confirms the theoretical predictions of the Standard Model but also deepens our understanding of the fundamental forces governing the universe. The conclusion of this article serves to encapsulate key insights discussed throughout and reflects on the implications of these findings.
Summary of Key Insights
The Higgs boson is central to our comprehension of mass generation in elementary particles. Understanding its properties and behaviors has led to several important conclusions:
- Mass Generation: The Higgs mechanism explains how particles acquire mass. Without it, the universe would be made up of massless particles, failing to form structures we witness today.
- Experimental Confirmation: The Large Hadron Collider has played a crucial role in confirming the existence of the Higgs boson through a series of experiments. The successful detection of this particle in 2012 was a landmark achievement for particle physics.
- Future Research: The study of the Higgs boson is ongoing. There are numerous open questions regarding its properties and its implications for theories beyond the Standard Model. Each discovery leads to new avenues of inquiry.
The implications of these insights extend beyond theoretical physics. They challenge existing paradigms and inspire new research directions. Understanding the Higgs boson bolsters our grasp on the universe's fundamental nature.
The Ongoing Quest for Knowledge
Science does not stand still. The journey into particle physics and the nature of the Higgs boson is a continuous pursuit of knowledge. Numerous factors contribute to this ongoing quest:
- Technological Advances: Innovations in detector and computational technologies at facilities like CERN allow for deeper exploration of the Higgs boson and its properties.
- Interdisciplinary Approaches: Collaboration across various scientific disciplines fosters a richer understanding of complex concepts associated with the Higgs boson. From cosmology to mathematics, the insights gained are invaluable.
- Philosophical Inquiry: The nature of science prompts us to ask deeper questions about the fabric of reality. Each discovery invites debate and reflection on the implications for human understanding.
As researchers delve deeper into the mysteries of the universe, the Higgs boson remains a focal point. Its study signifies not just the search for understanding but also embodies the scientific spirit. In the quest for knowledge, every answer paves the way for more questions, promoting growth in our collective intellect and insight into the cosmos.