Understanding Cell Life: A Complex Biological Narrative
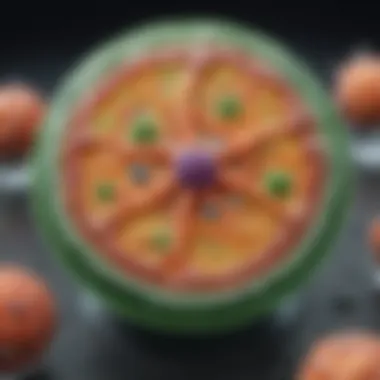
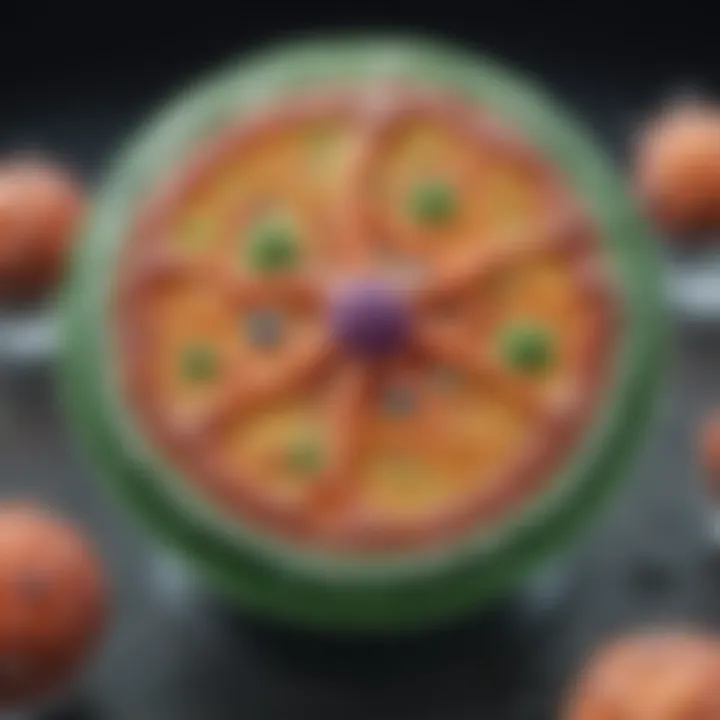
Intro
Cells are the basic building blocks of all living organisms. Their complexity is often underestimated, yet they perform an incredible array of functions necessary for life. Understanding the life of cells provides key insights into the larger biological systems at play in all forms of life. This section intends to prepare the ground for delving into the intricacies of cellular biology, underpinning its significance in various scientific fields.
In the subsequent sections, we will uncover the structure and processes that define cellular life. This narrative will take a closer look at cell theory and cellular metabolism, emphasizing how environmental factors impact cellular behavior. Moreover, insights drawn from contemporary research will be highlighted, marking the evolution of our understanding of cell dynamics.
Consequently, readers will gain a deeper comprehension of cellular mechanisms. The aim is to bridge the divide between complex scientific theories and accessible knowledge, making it relevant for students, researchers, educators, and professionals alike.
Key Research Findings
Examining key research findings allows us to understand how modern science has advanced our knowledge of cells.
Overview of Recent Discoveries
Recent studies have unveiled significant insights into cellular functions. The advent of advanced imaging techniques, such as super-resolution microscopy, has permitted scientists to observe cellular structures in real time. Researchers have also identified new cellular components, like exosomes, which play roles in cellular communication and waste disposal. Another fascinating discovery includes the role of mitochondria not just in energy production, but also in cellular signaling.
Significance of Findings in the Field
These findings carry profound implications for the fields of medicine and biotechnology. By understanding how cells communicate and interact with their environment, researchers can devise targeted therapies for diseases such as cancer and neurodegenerative conditions.
"The intersection of cellular biology and technology presents opportunities for revolutionary advancements in healthcare and therapeutics."
This newfound perspective on cellular mechanisms illuminates the path towards innovative approaches in treatment and understanding of various biological processes.
Breakdown of Complex Concepts
Reducing the complexity of cellular biology helps broaden understanding and accessibility.
Simplification of Advanced Theories
When discussing cell theory, we must break it down into accessible components:
- All living organisms consist of cells.
- Cells are the basic unit of life.
- All cells arise from pre-existing cells.
These principles underscore the universal nature of cellular life and its foundational role in biology.
Visual Aids and Infographics
To grasp cellular mechanisms better, visual aids can be immensely helpful. Infographics illustrating processes like cellular metabolism or signal transduction can reinforce understanding. By presenting data visually, learning becomes more engaging and retention improves.
In summary, this article seeks to explore the multifaceted world of cells while providing relevant insights and simplifying complex ideas for a diverse audience.
Preface to Cell Life
The study of cell life is fundamental in understanding biological principles. Cells are often regarded as the basic unit of life, forming the building blocks of all living organisms. Their study leads to insights not only about their function but also about the broader mechanisms that sustain life itself. Understanding cell biology provides a framework to explore the interactions between various cellular components and their environment.
One pivotal aspect to consider is how knowledge of cell structure and function informs various fields such as medicine, genetics, and ecology. For instance, recognizing how cells operate under different conditions can guide medical research in treating diseases. Knowing the differences in cellular responses can lead to advancements in therapies tailored to specific conditions.
In this section, we will discuss two key elements in cell life. The first is the basic unit of life, which encapsulates the essential properties that define a cell. The second focuses on cellular diversity, offering insights into the variation observed across different organisms. This discussion aims to illuminate the underlying principles that connect cellular structures and functions to the greater tapestry of life.
The Basic Unit of Life
Cells are characterized as the basic unit of life. This definition is rooted in the cell theory, which posits that all living organisms are composed of one or more cells. Each cell functions as a self-contained entity with its own processes that sustain life. It can perform essential life functions such as metabolism, growth, and reproduction.
Some key features of cells include:
- Cell Membrane: A semi-permeable barrier that regulates the entry and exit of substances.
- Cytoplasm: A gel-like substance where cellular components reside and metabolic reactions occur.
- Genetic Material: DNA is contained within the nucleus, directing cellular activities and hereditary traits.
Together, these components create a dynamic system that responds to internal and external stimuli, which is vital for survival. This intricate interplay within cells ensures they adapt to varying conditions, thus exemplifying their significance as the fundamental units of life.
Overview of Cellular Diversity
Cellular diversity refers to the vast range of cell types observed across various life forms. From unicellular organisms like bacteria to complex multicellular organisms such as humans, the variety of cell structures and functions is immense. This diversity is essential as it allows different cells to fulfill specialized roles within organisms.
For example, consider the following categories of cells:
- Prokaryotic Cells: Simple cells without a nucleus, exemplified by bacteria. They are typically smaller and less complex than eukaryotic cells.
- Eukaryotic Cells: More complex and larger, containing a nucleus and organelles. These are found in plants, animals, fungi, and protists.
Understanding cellular diversity not only enhances our grasp of biological complexity but can also lead to breakthroughs in medical and environmental research. By studying how different cells operate, scientists can develop targeted therapies for diseases or innovate sustainable practices in agriculture. The rich tapestry of cell types showcases the evolutionary journey of life, highlighting adaptation and functionality across species.
Cell Theory
Cell theory serves as a foundational pillar in biology, shaping our understanding of the structure and function of all living organisms. This theory is not just a collection of observations; it integrates historical significance and core concepts that define modern cellular biology. A robust grasp of cell theory enables researchers, students, and educators alike to appreciate the complexity of life at the cellular level.
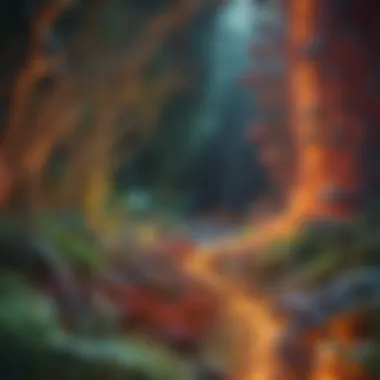
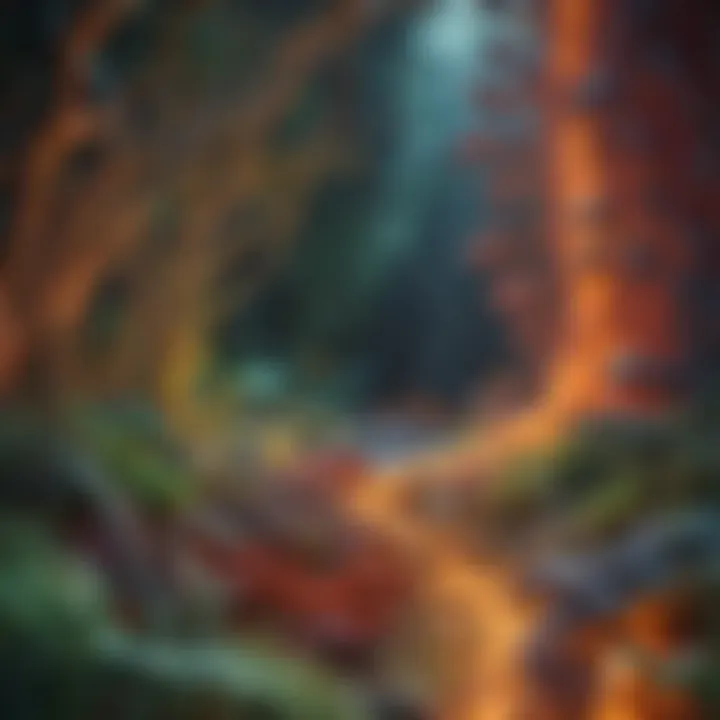
Historical Perspectives
The development of cell theory began in the 19th century, significantly influenced by pivotal figures such as Matthias Schleiden and Theodor Schwann. In 1838, Schleiden proposed that all plant tissues are composed of cells, while Schwann extended this idea to animals in 1839, suggesting that all living things are formed from cells. This marked a significant shift in biological understanding, moving away from earlier notions that life was spontaneous. Over the years, cells were studied under microscopes, revealing the diversity of cell types and their intricate structures.
As research progressed, scientists like Rudolf Virchow emphasized that all cells arise from pre-existing cells, adding depth to the theory. This historical context is critical, as it illustrates the evolution of our understanding and the cumulative nature of scientific knowledge.
"All life is cellular; without cells, life itself would not exist."
Core Principles of Cell Theory
Cell theory is underpinned by three core principles:
- All living organisms are composed of one or more cells. This principle highlights cell as the basic unit of life, underscoring that whether single-celled or multicellular, life is inherently tied to cellular structures.
- The cell is the basic unit of life. Every organism, no matter how complex, relies on the cellular processes that govern its existence. Cells perform essential functions that sustain life, from metabolism to energy production.
- All cells arise from pre-existing cells. This principle negates the idea of spontaneous generation and confirms that cell division is fundamental to growth and reproduction.
Understanding these principles enables a clearer perspective on cellular processes and their implications in various biological fields, from genetics to medicine. Cell theory not only unifies various biological disciplines but also highlights the importance of cells in life sciences, influencing ongoing research and discoveries.
In summary, cell theory is not just a historical naming; it is an ongoing narrative that clarifies our understanding of life itself. Recognizing the significance of this theory is essential for anyone engaged in the study of biology.
Cell Structure
Understanding the structure of cells is fundamental in cellular biology. The cell serves as the basic unit of life, encapsulating the myriad of processes that sustain organisms. Appreciating the intricacies of cell structure helps in the understanding of how cells interact with their environments, perform specialized functions, and ultimately contribute to the overall functionality of an organism.
Prokaryotic vs. Eukaryotic Cells
Prokaryotic and eukaryotic cells present distinctive designs that underline their functionality. Prokaryotic cells, such as bacteria, are generally simpler and smaller. They lack a defined nucleus and organelles. Instead, their genetic material floats within the cytoplasm in a region called the nucleoid. Their structure allows for rapid reproduction and adaptation to various environmental conditions. In contrast, eukaryotic cells contain a well-defined nucleus and are typically larger and more complex. This complexity allows for compartmentalization of various cellular functions. The presence of organelles enables more efficient processes, such as energy production and protein synthesis.
Key differences include:
- Size: Prokaryotic cells typically range from 0.1 to 5.0 micrometers, whereas eukaryotic cells are generally 10 to 100 micrometers.
- Nucleus: Prokaryotic cells do not have a nucleus, while eukaryotic cells have a membrane-bound nucleus.
- Organelles: Eukaryotic cells contain various organelles like mitochondria and endoplasmic reticulum, which are absent in prokaryotes.
In summary, the structural differences between prokaryotic and eukaryotic cells reflect their distinct evolutionary paths and functions in the biological world.
Organelles and Their Functions
Organelles play crucial roles in the life of a eukaryotic cell. Each organelle is specialized for specific functions, contributing to the overall health and efficiency of the cell.
Common Organelles and Their Functions
- Nucleus: Houses genetic material and coordinates cell activities, including growth and reproduction.
- Mitochondria: Often referred to as the "powerhouses of the cell," they generate ATP, providing energy for various cellular processes.
- Endoplasmic Reticulum (ER): The rough ER synthesizes proteins, while the smooth ER is involved in lipid synthesis and detoxification.
- Golgi Apparatus: Modifies, sorts, and packages proteins and lipids for secretion or delivery to other organelles.
- Lysosomes: Contains digestive enzymes to break down waste materials and cellular debris.
Understanding these organelles gives insight into how cells maintain their interior organization and respond to their external environment. This knowledge is paramount for comprehending various biological processes, including metabolism, energy production, and cellular signaling.
"The complexity and specialization of organelles illustrate the evolution of cellular functions and adaptations across different life forms."
Cell Membrane Dynamics
Cell membrane dynamics is a crucial area in cell biology. It refers to the processes and structures that govern how cells interact with their environment. This section will elaborate on the fundamental aspects of cell membrane structure and the various mechanisms of transport. Understanding these components is essential for grasping how cells maintain homeostasis and communicate with other cells. The cell membrane acts as a barrier, regulating the entry and exit of substances crucial for survival and function.
Structure of the Cell Membrane
The cell membrane, or plasma membrane, is composed primarily of phospholipids, which form a bilayer. This bilayer provides both structure and flexibility, allowing the membrane to adapt to various conditions while maintaining integrity. Embedded within the bilayer are proteins that perform various functions such as transport, signaling, and acting as receptors.
The fluid mosaic model explains the dynamic nature of the membrane. Proteins can move laterally within the lipid bilayer, which contributes to the cell's ability to respond to changes in the environment. Additionally, cholesterol molecules interspersed within the membrane help to stabilize its fluidity. This structure is essential for cell functionality and interactions, emphasizing the importance of cell membrane dynamics in cellular biology.
Transport Mechanisms
The transport mechanisms of the cell membrane are vital for maintaining cellular homeostasis. They determine how substances move in and out of the cell, ensuring the right conditions for cellular processes. There are three primary mechanisms: diffusion, osmosis, and active transport.
Diffusion
Diffusion is the process by which molecules spread from an area of higher concentration to one of lower concentration. This spontaneous movement occurs until equilibrium is reached. Diffusion is beneficial because it does not require energy, allowing for efficient exchange of substances like oxygen and carbon dioxide between the cell and its surroundings.
One key characteristic of diffusion is its reliance on concentration gradients. The greater the difference, the faster the rate of diffusion. However, this mechanism has limitations, as it primarily applies to small, nonpolar molecules. Larger or charged molecules typically cannot diffuse freely across the membrane, thereby necessitating other transport methods.
Osmosis
Osmosis specifically refers to the movement of water across a selectively permeable membrane. Water moves from areas of low solute concentration to high solute concentration, balancing solute levels on either side of the membrane. Osmosis is critical for cellular function, as it influences cell turgor and volume.
The unique feature of osmosis is its ability to affect cell shape and size through water movement. In plant cells, for example, osmosis helps maintain turgor pressure, an essential aspect of structural integrity. Conversely, animal cells may suffer under or over osmosis, leading to potential cell lysis or shrinkage.
Active Transport
Active transport is a mechanism that moves molecules against their concentration gradient, meaning from areas of lower concentration to ones of higher concentration. This process requires energy, often derived from ATP, allowing cells to intake substances necessary for survival, regardless of external concentration conditions.
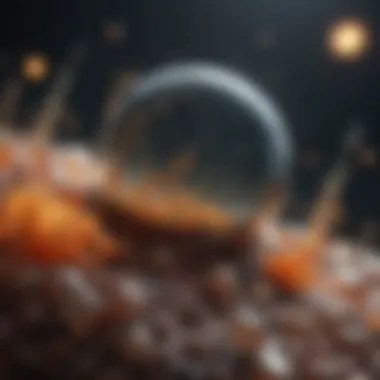
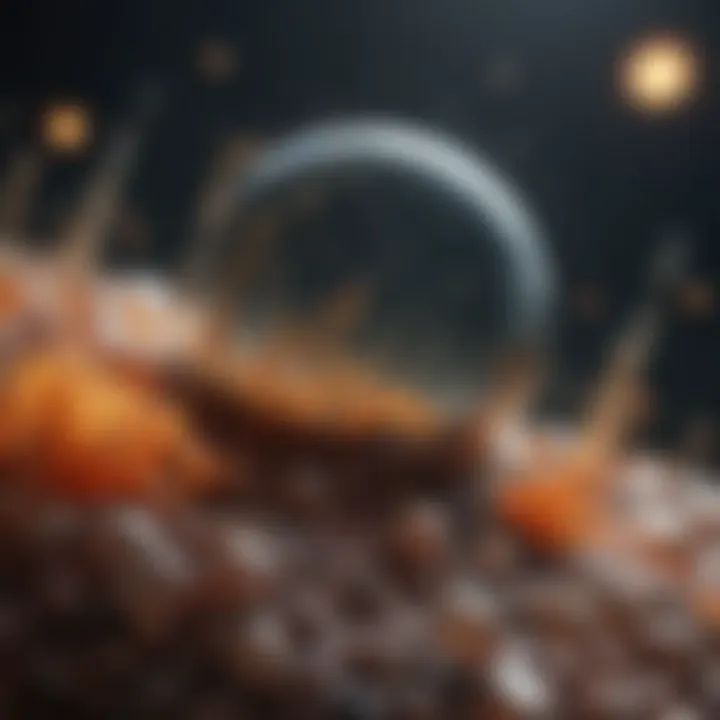
A key characteristic of active transport is its specificity. Transport proteins facilitate the movement of certain ions or molecules, ensuring that the cell obtains essential nutrients while expelling waste. An example of this is the sodium-potassium pump, which is critical for nerve impulse transmission. However, the energy requirement makes this mechanism energetically expensive compared to passive transport methods.
"Understanding cell membrane dynamics is indispensable for comprehending how cells interact and maintain their internal environment."
In summary, cell membrane dynamics plays a crucial role in regulating cellular processes. The structure and various transport mechanisms work together to ensure that cells function optimally in response to their environment.
Cellular Metabolism
Cellular metabolism is a central theme in understanding the biological narrative of life. It encompasses all the chemical reactions that occur within a cell, allowing it to maintain its structures, transport materials, and fuel its activities. The significance of metabolism lies in its ability to convert food into energy, which is essential for every cellular function. Moreover, understanding how cells extract and utilize energy can shed light on broader biological processes.
Energy Production
Aerobic Respiration
Aerobic respiration is a key process in energy production within cells. It occurs in the presence of oxygen, allowing cells to efficiently convert glucose into adenosine triphosphate (ATP), the cell's energy currency. One key characteristic of aerobic respiration is its high energy yield, producing up to 36 ATP molecules from a single glucose molecule. This beneficial aspect makes it an attractive choice for cells that thrive in oxygen-rich environments. The unique feature of this process is the use of the electron transport chain, which helps in maximizing energy extraction. However, a disadvantage is that it requires oxygen, limiting the organisms that depend on it.
Anaerobic Respiration
Anaerobic respiration, contrastingly, occurs when oxygen is absent. This process allows cells to generate energy through fermentation or similar pathways, producing either lactic acid or ethanol depending on the organism. Its key characteristic is the ability to sustain energy production in hypoxic environments, making it beneficial for organisms such as yeast and certain bacteria. The unique feature of anaerobic respiration is its speed, allowing for rapid ATP production, though it yields only 2 ATP molecules per glucose. The main disadvantage is that the byproducts can be toxic, such as lactic acid buildup in muscle cells during intense activity.
Photosynthesis
Photosynthesis is crucial for energy conversion in autotrophic organisms, primarily plants. This process transforms light energy into chemical energy stored in glucose. The key characteristic of photosynthesis is its role in producing not only energy for the plant itself but also oxygen as a byproduct, which supports aerobic life forms. It is beneficial as it forms the basis of the food chain, providing energy for herbivores, and subsequently carnivores. The unique feature is the conversion of solar energy into a usable biochemical form. However, the dependence on sunlight can be seen as a disadvantage, particularly in environments with limited light availability.
Metabolic Pathways
Metabolic pathways are interconnected networks of reactions that facilitate metabolic processes within cells. These pathways can be categorized as catabolic or anabolic. Catabolic pathways break down molecules to release energy. They are crucial for energy production and involve processes like glycolysis and the citric acid cycle.
In contrast, anabolic pathways utilize energy to synthesize complex molecules from simpler ones, supporting growth and repair. Each pathway is tightly regulated, ensuring cells can effectively respond to their internal and external environments. Research in metabolic pathways investigates how cells optimize their energy use and adapt to changing conditions, aiding in the understanding of various diseases, including cancer and metabolic disorders.
Understanding cellular metabolism is key to advancing biomedicine and biotechnology, as it reveals how energy production impacts cellular health and disease.
Through detailing energy production methods and metabolic pathways, this section underscores the significance of cellular metabolism in maintaining life, illustrating its relevance in both fundamental biology and applied sciences.
Cell Cycle and Division
The cell cycle and division are essential processes in biology, determining how cells grow, replicate, and ultimately divide into daughter cells. This subject is crucial within the context of cellular life, as it not only connects to organismal growth and development but also plays a significant role in understanding various diseases, including cancer. When we explore cell cycle and division, we can better appreciate the elegance and complexity of cellular functioning.
Phases of the Cell Cycle
The cell cycle consists of several distinct phases, each critical for ensuring the proper division of cells. These phases can generally be grouped into two major periods: interphase and the mitotic (M) phase.
- Interphase: This is the longer phase where the cell prepares for division. It is divided into three sub-phases:
- M Phase (Mitosis): This is the phase where cell division occurs, resulting in two daughter cells. Mitosis itself is subdivided into several stages: prophase, metaphase, anaphase, and telophase, followed by cytokinesisโwhere the cytoplasm divides, completing the cell division process.
- G1 Phase (Gap 1): In this stage, the cell grows and synthesizes proteins necessary for DNA replication. Cellular components are produced to support subsequent phases.
- S Phase (Synthesis): Here, DNA replication occurs. Each chromosome is duplicated, creating sister chromatids.
- G2 Phase (Gap 2): During this phase, further growth occurs along with the synthesis of proteins needed for mitosis. The cell checks for DNA errors before proceeding.
Understanding these phases helps in grasping how cells maintain their genetic integrity and function in a coordinated manner. Each phase plays a vital role in the orderly progression of the cell cycle.
Significance of Mitosis and Meiosis
Mitosis and meiosis are two key forms of cell division, serving different purposes.
- Mitosis: This is responsible for somatic cell division. Mitosis ensures that each daughter cell receives an identical set of chromosomes. This process is vital for growth, repair, and asexual reproduction in some organisms.
- Meiosis: In contrast, meiosis is a specialized type of cell division that produces gametes (sperm and eggs) in sexually reproducing organisms. Meiosis involves two rounds of division, leading to four non-identical daughter cells, each with half the original number of chromosomes. This reduction is essential for maintaining the genetic constants across generations when gametes unite during fertilization.
Importance of Understanding Mitosis and Meiosis:
- Disease Insight: Disruptions in mitosis can lead to cancer as cells replicate uncontrollably.
- Genetic Diversity: Meiosis is crucial for genetic variation, creating unique combinations of genes.
"Cells are the basic units of life, and their division is a fundamental process necessary for all organisms. Understanding these processes allows insight into both health and disease."
Cell Communication
Cell communication is a vital component of cell biology. It refers to the processes through which cells interact with each other and their environment. This interaction is crucial for maintaining homeostasis and regulating various cellular functions. Understanding how cells communicate helps to illuminate numerous biological mechanisms, including development, immune responses, and signal regulation in multicellular organisms.
Cell communication not only enables organisms to adapt to changes in their environment but also plays a role in the coordinated functions necessary for life. Disruptions in these communication processes can lead to diseases, including cancer and autoimmune disorders. Therefore, exploring cell communication is essential for comprehending the broader implications of cellular functions and relationships.
Mechanisms of Communication
Cell communication occurs through several mechanisms. The primary methods include:
- Direct Cell-to-Cell Contact: This involves physical connections between cells, often mediated through proteins on their surfaces. Gap junctions in animal cells and plasmodesmata in plant cells allow for direct transfer of ions and small molecules.
- Chemical Signaling: Cells release signaling molecules, such as hormones or neurotransmitters, which travel to target cells. This can occur over long distances, impacting physiology and behavior at various levels.
- Paracrine Signaling: In this local signaling mechanism, cells release substances that affect neighboring cells. Examples include growth factors and cytokines that regulate processes like tissue repair and immune responses.
- Autocrine Signaling: In this type, cells respond to molecules they themselves secrete. This mechanism helps regulate cell proliferation and survival, often seen in cancerous cells that exploit this process.
Effective communication is critical for cellular activities such as growth, differentiation, and response to external stimuli.
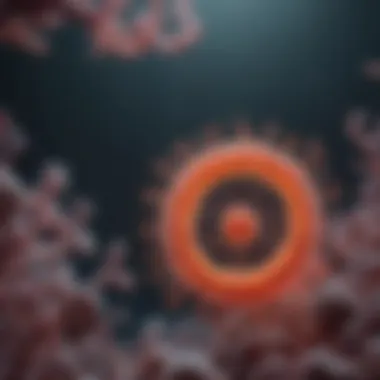
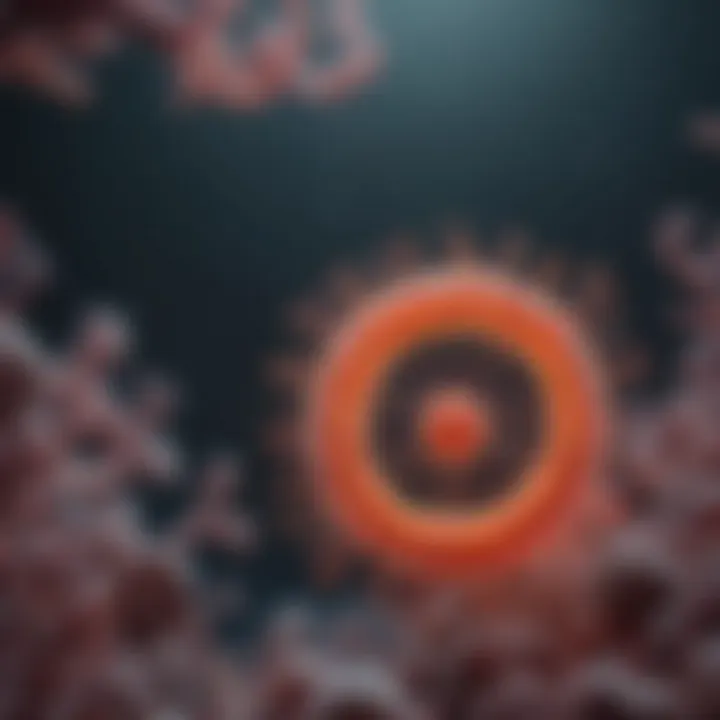
Signal Transduction Pathways
Signal transduction pathways are intricate networks that relay signals from outside the cell to internal targets. These pathways begin when a signaling molecule, known as a ligand, binds to a specific receptor on the cell membrane. Key components of signal transduction include:
- Receptors: These are proteins located on the cell surface, dedicated to recognizing and binding specific ligands.
- Secondary Messengers: Once activated, receptors often trigger the release of secondary messengers within the cell, such as cyclic AMP or calcium ions. These small molecules amplify the signal and propagate the response.
- Effectors: Ultimately, the signal must lead to a specific cellular response, often mediated by proteins that influence gene expression or enzymatic activity.
- Feedback Mechanisms: Many pathways include feedback loops that ensure signaling is appropriately regulated. Positive feedback can amplify signals, while negative feedback can dampen them, providing balance to cellular responses.
Signal transduction pathways are highly specific. This specificity ensures that cells can finely tune their response to diverse stimuli. Disruptions in these pathways can lead to various pathologies, highlighting the importance of understanding these intricate networks.
Environmental Impact on Cells
Understanding the environmental impact on cells is a critical component in cell biology. Cells exist within dynamic contexts, where fluctuations in their surroundings can significantly influence their functions. This interplay between cells and their environment is not just a matter of academic curiosity; it is vital for applications in fields ranging from medicine to agriculture. By examining the factors such as temperature, pH, and the presence of toxins, we can better understand cellular behavior and adaptation.
Influence of Temperature and pH
Temperature and pH are among the most influential environmental variables affecting cellular processes. Each organism has an optimal temperature range where cell enzymes function most effectively. When temperatures deviate from this range, cellular metabolism can falter. For example, higher temperatures might enhance enzymatic reactions up to a threshold, beyond which proteins can denature, leading to cellular dysfunction.
Similarly, pH affects the ionization of molecules essential for cellular activity. Enzymes, which are critical for Catalyzing biochemical reactions, often have specific pH ranges for optimal activity. For instance, human enzymes typically operate best around pH 7.4. Deviations can lead to reduced activity, impacting metabolic pathways.
"Cells are finely tuned instruments, sensitive to every nuance in their environment. Temperature and pH act as conductors, guiding cellular symphonies of activity or discord."
Toxicology and Cellular Response
The study of toxicology emphasizes how cells respond to harmful substances present in their environment. Toxic agents can disrupt various cellular functions, leading to detrimental effects. Cells can encounter toxins from environmental pollutants, medications, or naturally occurring compounds. Their survival often hinges on the mechanisms they employ to counteract these threats.
Cells activate stress response pathways when exposed to toxins. For instance, they may upregulate the expression of antioxidant enzymes that neutralize reactive oxygen species generated by toxic exposure. Furthermore, cellular detoxification systems like the cytochrome P450 enzymes can metabolize harmful substances, rendering them less harmful or promoting their excretion.
Understanding these responses not only enhances our knowledge of cellular resilience but also sheds light on potential therapeutic strategies. Researchers can design interventions that could either bolster these natural defenses or target specific weaknesses in cancer cells known to exploit their environmental conditions for survival.
Current Research in Cell Biology
Current research in cell biology plays a critical role in advancing our understanding of cellular mechanisms and their implications for various fields including medicine, genetics, and biotechnology. By examining the latest techniques and discoveries in cell biology, we can identify potential solutions for complex biological challenges. This deeper understanding informs development of new therapies, diagnostic tools, and enhances our grasp on cellular behavior in diverse environments.
Cutting-Edge Techniques
CRISPR Technology
CRISPR technology has emerged as a groundbreaking method in the field of genetics. It allows for precise editing of DNA, which offers immense potential for correcting genetic defects. The key characteristic of CRISPR technology is its ability to target specific sequences in the genome with high accuracy. This aspect is what makes it a popular choice in research and therapeutic applications.
A unique feature of CRISPR is that it is comparatively easier and cheaper to use than previous gene-editing methods. Its advantages include rapid development in experimental setups and the ability to address a wide array of genetic maladies. Nonetheless, there are disadvantages. Ethical concerns regarding gene editing in humans and potential off-target effects can complicate its application. Balancing innovation and safety is a persistent challenge in this area.
Single-Cell Analysis
Single-cell analysis is crucial for understanding cellular diversity and functionality at an individualized level. This technique allows researchers to investigate the differences between cells within the same tissue type, offering insights into their unique roles and behaviors. The key characteristic of single-cell analysis lies in its resolution, which can reveal heterogeneous characteristics that bulk analysis can overlook.
One prominent feature of single-cell analysis is its capacity to assess gene expression profiles from individual cells, providing a detailed view of cellular activities. Its advantages include revealing rare cell populations, which may play significant roles in disease pathways. However, the drawbacks can include high costs and the need for sophisticated equipment. These limitations may hinder accessibility for some research institutions.
Emerging Areas of Study
Emerging areas of study in cell biology are expanding our understanding significantly. Research is currently exploring novel approaches, such as synthetic biology and regenerative medicine. These areas have the potential to revolutionize traditional methods of treatment and enhance our capabilities in tissue engineering.
Additionally, the study of stem cells has gained momentum, offering insights into their ability to differentiate into various cell types. Discovering how to manipulate these cells holds promise for combating degenerative diseases. As research progresses, it is imperative to maintain a focus on ethical considerations, ensuring that scientific advancements align with societal values.
The Future of Cell Research
The future of cell research presents dynamic possibilities that could significantly reshape our understanding of biology and medicine. As we delve deeper into the workings of cells, the implications extend far beyond mere academic interest. Striding forward, we can anticipate breakthroughs that will directly impact therapeutic approaches and our comprehension of human health.
The expanding field of cell research encourages innovations that drive new techniques. With the development of high-resolution imaging and advanced genetic editing methods, such as CRISPR, researchers can investigate cellular processes with unparalleled clarity. These advancements promise to revolutionize disease treatment and prevention.
Moreover, cell research plays a crucial role in regenerative medicine, helping to create therapies that may one day allow for the repair of damaged tissues and organs. This could lead to treatments for conditions such as heart disease, diabetes, and neurodegenerative disorders like Alzheimer's. The potential to cultivate cellular models of these diseases for research enhances drug discovery, thereby increasing the likelihood of successful therapeutic interventions.
Potential Implications for Medicine
The implications for medicine stemming from future cell research are vast. Firstly, we see the promise in personalized medicine, where treatments can be tailored to individual cellular profiles. Understanding a patient's specific cellular reactions can help healthcare providers select the most effective therapies.
In addition, regenerative medicine leverages insights from cellular mechanisms to develop procedures that repair or replace damaged cells and tissues. For instance, stem cell therapy is at the forefront here, promising revolutionary treatments for conditions like spinal cord injuries or degenerative diseases.
Further advances in stem cell research could allow for organ regeneration, vastly reducing waiting times for transplants and minimizing the need for donor organs.
The integration of biotechnology with cell research also leads to the development of smart drugs that interact with specific cell types, minimizing side-effects and increasing Efficacy.
Ultimately, the trajectory points toward an era of targeted and efficient healthcare solutions that enhance patient outcomes while reducing costs.
Ethical Considerations
With the great potential of future cell research comes significant ethical considerations. As we harness the power of technologies such as CRISPR, the line between therapy and genetic enhancement becomes increasingly blurred. Issues arise about the potential misuse of gene-editing technology for non-therapeutic enhancements, raising questions about societal inequality and access to such advancements.
Furthermore, stem cell research, notably involving embryonic stem cells, presents ethical dilemmas surrounding the moral status of embryos. Different cultures and belief systems have varied perspectives on this issue, which complicates consensus on ethical guidelines.
As research continues to forge new paths, regulatory frameworks will need to adapt quickly to manage the ethical implications associated with these breakthroughs.