Understanding Black Hole Physics: Insights and Discoveries
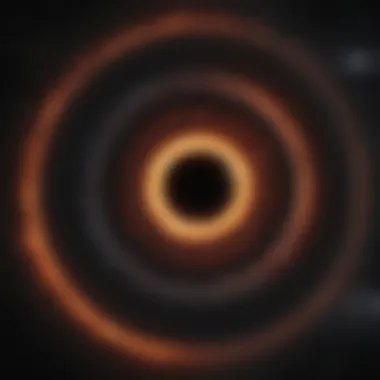
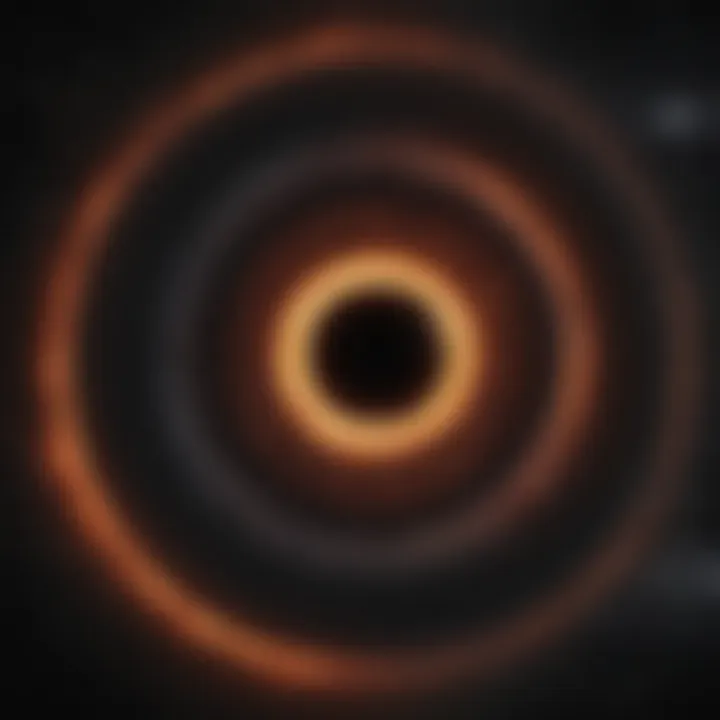
Intro
Black holes remain one of the most fascinating and enigmatic components of our universe. Understanding their physics is critical for grasping not only their nature but also the implications they hold for the fabric of spacetime itself. In this article, we will embark on a journey to explore the latest research findings, unravel complex theories, and analyze how these cosmic phenomena shape our view of the universe.
Through recent advancements in observational technology and theoretical frameworks, we can now piece together a more coherent picture of black hole formation and behaviors. This investigation will serve not only to enlighten our comprehension of black holes but also to enhance our appreciation for the vastness of astrophysics.
Key Research Findings
Overview of Recent Discoveries
In recent years, significant strides have been made in our understanding of black holes. One of the most notable discoveries is the compelling evidence for the existence of supermassive black holes at the centers of galaxies. Observations, particularly those made by the Event Horizon Telescope, have allowed scientists to capture the first image of a black hole's event horizon in the galaxy M87. This milestone not only validated theoretical predictions but also opened doors for further investigations into the nature of black holes.
Another crucial finding relates to gravitational waves. The merger of binary black holes detected by LIGO has revolutionized the field, confirming that black holes can collide and produce ripples in spacetime—evidence of their dynamic nature. These discoveries provide a rich dataset for understanding black hole population dynamics and their role in galaxy formation.
Significance of Findings in the Field
The implications of these recent discoveries are profound. They challenge long-held assumptions about the lifecycle of stars and the nature of dark matter. By understanding how black holes influence their surroundings, researchers can deepen their insights into galaxy evolution. Moreover, the data from gravitational waves contributes to astrophysical models, refining our understanding of massive stars' evolution and the role of black holes in generating heavy elements in the universe.
"The study of black holes is not just a search for these objects but an exploration of the fundamental laws that govern our universe."
Breakdown of Complex Concepts
Simplification of Advanced Theories
Black holes are often viewed through the lens of general relativity, which describes how they warp spacetime. However, understanding concepts like event horizons, singularities, and Hawking radiation can be daunting. Simplifying these ideas can make them more accessible. For instance, an event horizon can be described simply as the point of no return; once anything crosses it, escape is impossible.
Hawking radiation, on the other hand, suggests that black holes may eventually evaporate over time due to quantum effects near the event horizon. This theory connects the principles of quantum mechanics with general relativity, marking an essential intersection in theoretical physics.
Visual Aids and Infographics
Visual representations can enhance comprehension. Infographics illustrating black hole formation, types, and their effects on surrounding matter can provide valuable context. Charts showing the lifecycle of massive stars leading to black holes offer a simplified explanation of their genesis. Instructors and educators can effectively use these resources as teaching tools to facilitate learning.
By unraveling these complex subjects and translating them into more digestible formats, we can foster a deeper appreciation for black hole physics and its complexities.
Foreword to Black Holes
Understanding black holes is a crucial aspect of astrophysics that has garnered significant attention over the years. These enigmatic objects challenge our comprehension of physics, presenting both fundamental questions and remarkable phenomena. Black holes are not just theoretical constructs; they play a vital role in the dynamics of galaxies and the evolution of the universe. Their study offers insights into the laws of gravity, spacetime, and even quantum mechanics.
The exploration of black holes demands a multi-disciplinary approach. Researchers must integrate knowledge from various fields, including general relativity and quantum physics, to unravel their mysteries. Black holes also serve as laboratories for testing the limits of current theories. By examining their properties, we can probe the validity of Einstein's theories and explore new physics.
In this section, we will define black holes and highlight their basic concepts. We will also delve into the historical context, examining key discoveries that have shaped our understanding of these fascinating entities. By understanding the origins and definitions, we can appreciate their significance in modern astrophysics.
"Black holes are where God divided by zero."
— Unknown
As we embark on this journey, it is important to acknowledge the profound implications of black holes. They stretch our imagination and challenge our conceptual frameworks. Through rigorous exploration, we can hope to demystify these cosmic giants and unveil the secrets they hold.
Definition and Basic Concepts
A black hole is generally defined as a region in spacetime where gravitational pull is so strong that nothing, not even light, can escape it. The boundary of a black hole is known as the event horizon. Once an object crosses this boundary, it is irrevocably drawn into the black hole and cannot return. The concept of a black hole arises from Einstein's general theory of relativity, which indicates that massive objects warp the fabric of spacetime, creating deep gravitational wells.
Black holes can be categorized into several types based on their mass:
- Stellar black holes: These form from the remnants of massive stars that collapse under their own gravity after exhausting their nuclear fuel.
- Supermassive black holes: These reside at the centers of galaxies and possess millions to billions of times the mass of the Sun.
- Intermediate black holes: Their existence is still debated, but they are thought to form from the merging of smaller black holes.
- Micro black holes: Theoretical black holes that could form on a very small scale, potentially being created in high-energy conditions.
Each type of black hole presents unique characteristics and challenges for observational studies. Understanding these definitions and concepts is fundamental to advancing our knowledge of their nature and influence.
Historical Context and Discoveries
The concept of black holes dates back to early theorists. John Michell, in the 18th century, first proposed the idea of "dark stars," objects so massive that light could not escape their gravity. However, it wasn't until the 20th century, with the development of general relativity, that the modern concept of black holes began to take shape.
In 1916, Karl Schwarzschild derived a solution to Einstein's equations that described a black hole's structure. This solution introduced the concept of an event horizon. In the following decades, further developments occurred, leading to significant discoveries. For example, in the 1960s, the identification of quasars as supermassive black holes at the centers of galaxies transformed our understanding of these entities.
The late 20th and early 21st centuries witnessed technological advancements that provided evidence of black holes' existence. Observations of gravitational waves from colliding black holes and direct imaging conducted by the Event Horizon Telescope have consolidated their status in modern astrophysics.
Theoretical Foundations
Theoretical foundations in black hole physics form the bedrock of our understanding of these enigmatic objects. At its core, this section explores the principles that govern the behavior and nature of black holes. It highlights the crucial frameworks—most prominently General Relativity—that enable scientists to theorize about black holes. These foundations are essential not just for identifying characteristics of black holes but also for predicting their interactions and consequences in the universe. Understanding these theories can shed light on significant cosmic phenomena and the laws governing them.
General Relativity and Black Holes
General Relativity, proposed by Albert Einstein in 1915, is a cornerstone of modern physics and a fundamental aspect of black hole theory. This theory posits that gravity is not merely a force, as once thought, but a curvature of spacetime caused by mass. Black holes arise as solutions to the equations derived from General Relativity, demonstrating how massive stars collapsing under their own gravity can warp the fabric of spacetime to create regions from which nothing can escape.
The significance of General Relativity extends beyond just explaining black holes. It reshapes our entire perception of space and time. The ability to apply this theory to cosmic phenomena is key for astrophysicists in constructing models and making observations related to black holes.
Mathematics of Black Holes
The mathematical underpinnings of black holes comprise various solutions to Einstein's field equations, formulated under General Relativity. Each solution provides insight into different types of black holes, their formation, and properties. The following discussions focus on three principal solutions:
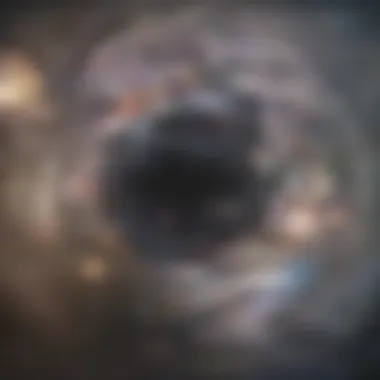
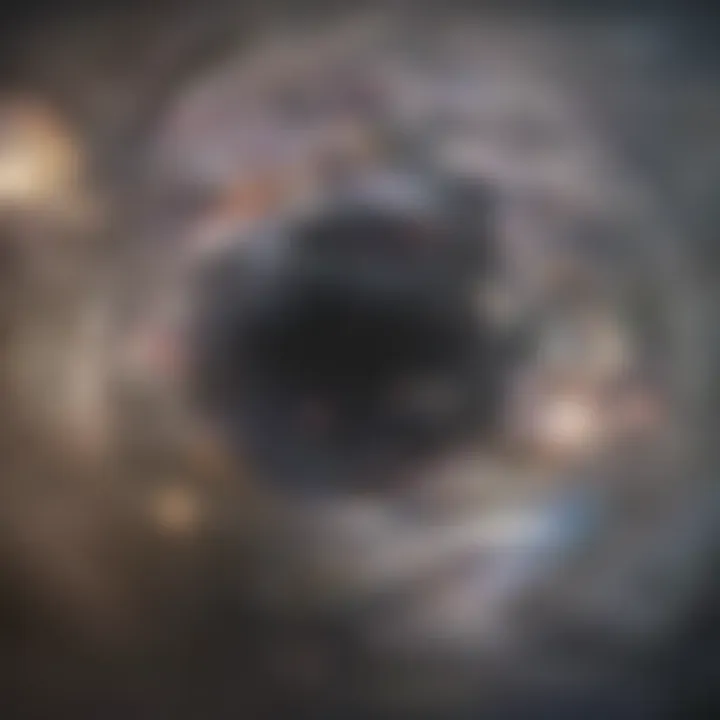
Schwarzschild Solution
The Schwarzschild solution describes a non-rotating, uncharged black hole. Its significance lies in its simplicity and elegance. This model demonstrates how mass generates gravitational wells, providing a basis for understanding more complex black holes. One key characteristic of the Schwarzschild black hole is its event horizon, a boundary beyond which no information can escape. This concept is beneficial for introductory discussions in black hole physics as it introduces foundational ideas without excessive complexity.
The unique feature of the Schwarzschild solution is its mathematical representation and the straightforward geometry it involves. However, while this model is pivotal for foundational knowledge, it has limitations; it does not account for rotating or charged black holes, which constitute a majority in the universe.
Kerr Black Holes
Kerr black holes represent a more complex solution that considers rotation. They are characterized by their angular momentum, offering a more realistic portrayal of most astronomical black holes. This model showcases how rotation increases the mass-energy and generates frame-dragging effects. This rotational aspect is crucial since many observed black holes exhibit significant spin.
The unique feature of Kerr black holes is their ergosphere, which allows intriguing phenomena, such as energy extraction. This model is a beneficial choice for its insight into real-world astrophysical observations compared to the Schwarzschild model. However, the added complexity necessitates a deeper understanding of the mathematics involved, which can pose a challenge for novices.
Reissner-Nordström Black Holes
The Reissner-Nordström solution describes charged black holes, which interact with electromagnetic fields. This solution exhibits similarities to the Schwarzschild solution but adds dimensions of complexity due to the influence of charge. The key characteristic here is its dual nature of mass and charge. Understanding charged black holes is essential since they depict the possibility that astronomical objects can interact with electromagnetic forces, not just gravitational ones.
The unique aspect of the Reissner-Nordström black holes is their ability to demonstrate how charge affects black hole behavior. This presents advantages in theoretical studies of electromagnetic phenomena in cosmic scales. However, fewer practical examples exist compared to uncharged and rotating black holes, making them less commonly discussed in basic introductions to black hole physics.
"Understanding these mathematical frameworks leads to profound insights into black holes and the universe’s fabric as a whole."
Formation of Black Holes
Stellar Evolution and Collapse
Stellar evolution is crucial in the lifecycle of stars, and it directly leads to the creation of black holes. Most stars spend a significant part of their lives fusing hydrogen into helium in their cores, producing energy that counterbalances the gravitational forces trying to collapse the star under its own weight. As the hydrogen gets exhausted, the star's core contracts, heating up until it starts fusing helium and heavier elements. This phase continues until iron forms, as iron fusion does not yield energy.
Once a massive star, typically greater than three solar masses, exhausts its fuel, it undergoes a dramatic core collapse. The core shrinks rapidly, while the outer layers may explode in a supernova, ejecting stellar material into space. This supernova leaves behind a highly dense core, which could become a neutron star or, if the remaining mass is sufficient, collapse into a black hole. This process illustrates how essential stellar life cycles are in the context of black hole genesis.
Primordial Black Holes
Primordial black holes present an intriguing concept in black hole formation. Unlike those formed via stellar evolution, primordial black holes could have formed soon after the Big Bang. This formation theory posits that density fluctuations in the early universe led to regions where matter density became so high that gravitational collapse occurred independently of stars.
These black holes could vary in size, from very small to potentially massive. Their existence remains speculative, but they could explain certain cosmic phenomena, such as dark matter. Several hypotheses suggest they would contribute to the universe's overall mass. However, proving their existence has been challenging, requiring advanced observational techniques and theories. Understanding primordial black holes enriches our conception of cosmic history and provides potential answers to contemporary questions concerning both dark matter and the overall evolution of the universe.
"The study of black hole formation is not just about black holes themselves; it's about understanding the full fabric of cosmic existence."
In summary, the formation of black holes encompasses both the terminal phases of stellar life and the early moments post-Big Bang. Both mechanisms illustrate the potency of gravitational forces and matter dynamics in shaping our universe. By studying how black holes form, we grasp fundamental astrophysical processes and deepen our knowledge of cosmic structure.
Types of Black Holes
The concept of black holes extends beyond a singular entity into various categories, each defined by unique characteristics and formation processes. Understanding the types of black holes is fundamental for comprehending their roles in the universe and their significance in astrophysics. Different types have distinct implications for the cosmic environment, stellar dynamics, and galactic formation.
Stellar Black Holes
Stellar black holes are formed from the gravitational collapse of massive stars after they exhaust their nuclear fuel. When a star runs out of energy, its core collapses under its weight. If the remaining mass is below a certain limit, it becomes a neutron star. However, if the mass exceeds this limit, it continues collapsing to form a black hole. Typically, stellar black holes have masses ranging from about three to several tens of solar masses.
Studying these black holes is crucial, as they showcase the life cycle of stars and offer insight into the processes of stellar evolution. They often reside in binary systems, where they can draw material from a companion star. This interaction leads to the emission of X-rays, providing a method for detection. The detection and study of stellar black holes have provided immense knowledge about their characteristics and formation.
Supermassive Black Holes
Supermassive black holes stand out due to their enormous mass, often found at the center of galaxies, including our Milky Way. They can contain millions to billions of solar masses. The precise mechanism behind their formation remains a subject of extensive research, but it likely involves a combination of factors, including the merging of smaller black holes and the rapid accretion of mass over time.
Their impact on galaxy formation and evolution cannot be overstated. The presence of supermassive black holes influences the orbits of stars within galaxies and affects the dynamics of their interstellar medium. Understanding these black holes is vital for uncovering the processes that govern galaxy formation and evolution.
Intermediate Black Holes
Intermediate black holes are somewhat less understood. They typically have masses ranging between a few hundred and a few thousand solar masses. Evidence for their existence comes from observations in globular clusters and some active galactic nuclei, but fewer clear examples exist compared to stellar and supermassive black holes.
The formation of intermediate black holes may occur through the merger of stellar black holes or by the direct collapse of massive gas clouds. Their study is significant because they serve as a bridge in understanding the gap between stellar and supermassive black holes. Solving the mystery of their existence can shed light on black hole growth and influence in the universe.
Micro Black Holes
Micro black holes are theoretical entities that possess much smaller mass than their stellar or supermassive counterparts, possibly as low as several times the mass of a proton. These entities are predicted to form under extreme conditions but have yet to be observed directly. They may offer insights into quantum gravity and the fundamental nature of spacetime itself. Some theories propose that they could have formed during the early moments of the Big Bang.
Exploring micro black holes raises intriguing questions regarding the very nature of black holes and their role in the universe. Their potential connection to quantum mechanics makes them a compelling area for future research and discussions in theoretical physics.
Understanding the different types of black holes is essential for decoding the behavior of our universe. Each category plays a distinct role in cosmic evolution.
Through studying these classifications, scientists gain valuable insights into the universe's architecture and the fundamental forces at play.
Black Hole Characteristics
The concept of black hole characteristics is essential in understanding their nature and their significant role in the cosmos. Characteristics such as the event horizon, singularity, and the acceleration of matter shed light on the underlying physical principles governing these regions of intense gravitational forces. By comprehensively exploring these characteristics, we can gain insights into how black holes influence surrounding environments and contribute to various astrophysical phenomena.
Event Horizon
The event horizon is arguably the most notable feature of a black hole. It is the boundary around a black hole beyond which nothing can escape, not even light. This characteristic delineates the point of no return. When matter crosses the event horizon, it is irrevocably drawn into the black hole. The significance of the event horizon extends beyond just being a boundary; it provides critical insights into the gravitational pull experienced by nearby objects.
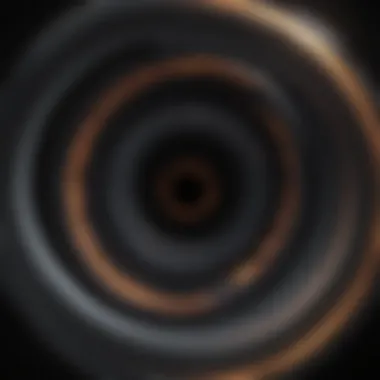
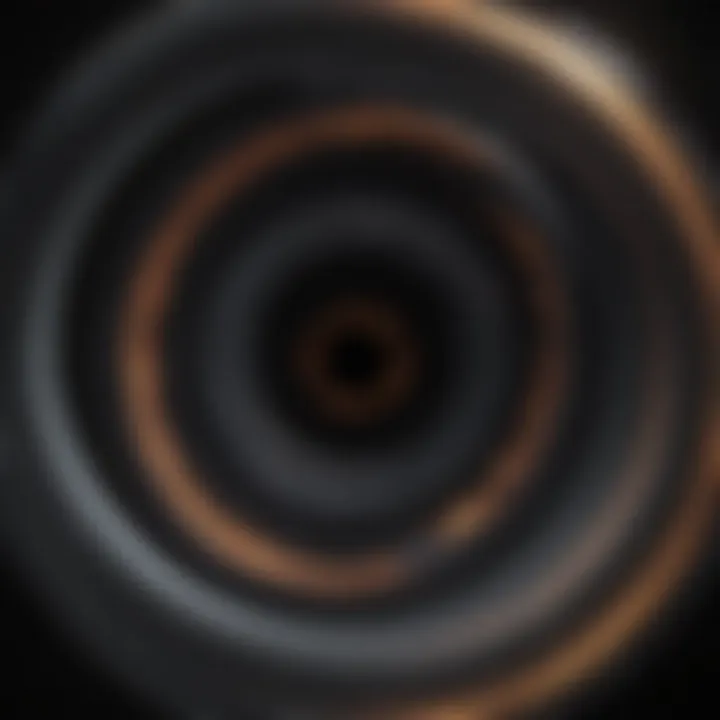
From a mathematical perspective, one can consider the Schwarzschild radius, which defines the radius of the event horizon for a non-rotating black hole. The strength of its gravitational influence becomes stronger as one approaches this radius. This leads to profound implications on the perception of time and space. For instance, observers at a safe distance perceive time moving normally, while for an object falling into the black hole, time appears to freeze at the event horizon. Such extreme conditions challenge conventional understanding and raise intriguing questions about the fabric of spacetime.
Singularity
At the core of a black hole lies the singularity, a point where gravitational forces compress matter to an infinitely small space. Within this zone, density and curvature of spacetime become infinite. Physicists face challenges in explaining the physics at this point due to the breakdown of known laws of physics, particularly general relativity.
The singularity represents a frontier of scientific inquiry. Theories such as quantum gravity aim to reconcile how quantum mechanics interacts with these extreme conditions. The study of singularity emphasizes the necessity for a unified theory that can connect general relativity with quantum mechanics. Understanding singularities also compels researchers to rethink the structure of spacetime and may shed light on the origins of the universe itself.
Acceleration of Matter
Another intriguing characteristic of black holes is the acceleration of matter in their vicinity. As objects approach a black hole, they experience intense gravitational forces that lead to rapid acceleration. This effect can be observed in the behavior of accretion disks. These disks are formed by material spiraling inward toward the black hole, accumulating in a toroidal shape.
The interaction of in-falling matter generates substantial energy, usually emitted in the form of X-rays or gamma rays. This observation provides critical evidence for the existence of black holes, as the high-energy emissions are detectable by modern telescopes. The study of matter acceleration not only enhances our understanding of black holes but also informs on how these massive objects shape their environments, influencing star formation and galactic structure.
Understanding black hole characteristics is pivotal in unraveling the complexities of the universe, as they are not mere cosmic voids but dynamic entities塑 shaping their surroundings.
Observational Evidence
Observational evidence is a cornerstone in the study of black holes. It bridges theoretical concepts with real-world implications. As concepts in black hole physics can often seem abstract, observations bolster the understanding of their existence and behavior. These empirical findings validate the predictions made by general relativity and augment our comprehension of the universe.
Astrophysicists have made significant strides in detecting black holes indirectly. They analyze their influence on surrounding matter and radiation. The methods of observation vary, tapping into various cosmic phenomena. Here, we discuss three crucial elements of observational evidence: gravitational waves, imaging techniques, and X-ray emissions.
Gravitational Waves and Merging Black Holes
In September 2015, the Laser Interferometer Gravitational-Wave Observatory (LIGO) detected gravitational waves for the first time. This landmark event confirmed a prediction made by Albert Einstein over a century ago, related to the merging of black holes. The waves originate from changes in spacetime caused by these cosmic collisions, a process where two massive black holes spiral towards each other and combine.
Gravitational wave detection has revolutionized the field of astrophysics. It offers a new tool to observe black holes that are otherwise invisible through traditional methods.
- Benefits of Gravitational Wave Observation:
- Provides direct evidence of black hole mergers.
- Allows for the study of population characteristics of black holes.
- Helps understand the dynamics of extreme gravity.
This method opens a new window into the universe, allowing scientists to explore phenomena that have cataclysmic implications, both theoretically and observationally.
Event Horizon Telescope and Imaging Black Holes
The Event Horizon Telescope (EHT) achieved a monumental feat by capturing the first image of a black hole. In April 2019, EHT unveiled a shadow of the supermassive black hole in the galaxy M87. This was made possible by linking radio dishes around the globe to function as one virtual Earth-sized telescope.
Imaging the event horizon is crucial because it provides visual confirmation of black holes and enhances our understanding of their size and structure.
- Key Aspects of EHT Observations:
- Visualizes the silhouette against the surrounding luminous gas.
- Confirms the existence of black holes in galaxies.
- Offers insights into the environment near event horizons.
These images enrich existing theories and stimulate further research into the physics of extreme gravitational environments.
X-ray Emission from Accreting Black Holes
X-ray emissions from accreting black holes are another vital line of evidence in black hole research. When matter falls into a black hole, it forms an accretion disk. This disk heats up to extreme temperatures, emitting X-rays detectable from Earth.
This form of observation allows for insights into black hole mass and spin. Astronomers track changes in the X-ray spectrum, leading to a more detailed understanding of the accretion process and the properties of the black hole itself.
- Importance of X-ray Emission:
- Identifies potential black holes by detecting their radiation.
- Reveals dynamics of matter in extreme gravitational fields.
- Provides statistical data about black hole populations across the universe.
In summary, these observational methods illuminate the otherwise hidden world of black holes. They reinforce theoretical constructs while paving the way for future discoveries. As technology progresses, our ability to perceive and understand these fascinating objects will only improve.
The Role of Black Holes in Astrophysics
Black holes play a crucial role in our understanding of the universe, influencing a variety of astrophysical processes. Their extreme gravitational fields not only challenge the laws of physics but also enhance our comprehension of cosmological phenomena. The study of black holes is not intellectual curiosity alone; it holds significant implications for galaxy evolution, star formation, and even dark matter. Thus, the exploration of black hole physics reveals intricate connections in astrophysics.
Impact on Galaxy Formation
Black holes significantly affect the formation and evolution of galaxies. Supermassive black holes often reside at the centers of galaxies, including our Milky Way. Their gravitational influence can regulate star formation in the surrounding regions. By either pulling matter towards them or ejecting energy and jets, supermassive black holes can shape the interstellar medium. This leads to environments where star formation rates are affected.
- Star Formation Suppression: When a black hole actively accretes matter, it can emit strong radiation. This can heat the surrounding gas, preventing it from collapsing into stars. The result is a dynamic interaction where star formation can be inhibited in the galaxy.
- Feedback Mechanisms: Supermassive black holes exert feedback through energetic outflows known as "AGN feedback" (from Active Galactic Nuclei). This affects the distribution of stars and gas in the galaxy, promoting or hindering further star formation.
This intricate dance between black holes and galactic structures leads to a better understanding of how galaxies change over time.
Influence on Stellar Dynamics
Black holes also exert profound influences on stellar dynamics within their vicinity. Their gravitational pull can alter the orbits of nearby stars. The presence of a black hole leads to phenomena such as the formation of binary star systems or the eventual consumption of stars by the black hole itself.
- Stellar Disruption Events: When stars venture too close to a black hole, they can be torn apart by gravitational forces. Such events, known as tidal disruption events, provide insightful data on the behavior of matter near extreme gravitational fields.
- Dynamics of Star Clusters: In dense star clusters, black holes can serve as a gravitational anchor, affecting the movement and accumulation of surrounding stars. Over time, this can lead to the formation of gravitational waves when stars collide or when black holes themselves merge.
Understanding these interactions deepens our insight into how stars are born, live, and die in the context of the greater universe. The interplay between black holes and stars presents a continuously evolving narrative in astrophysics.
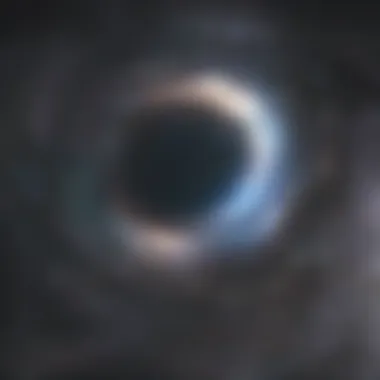
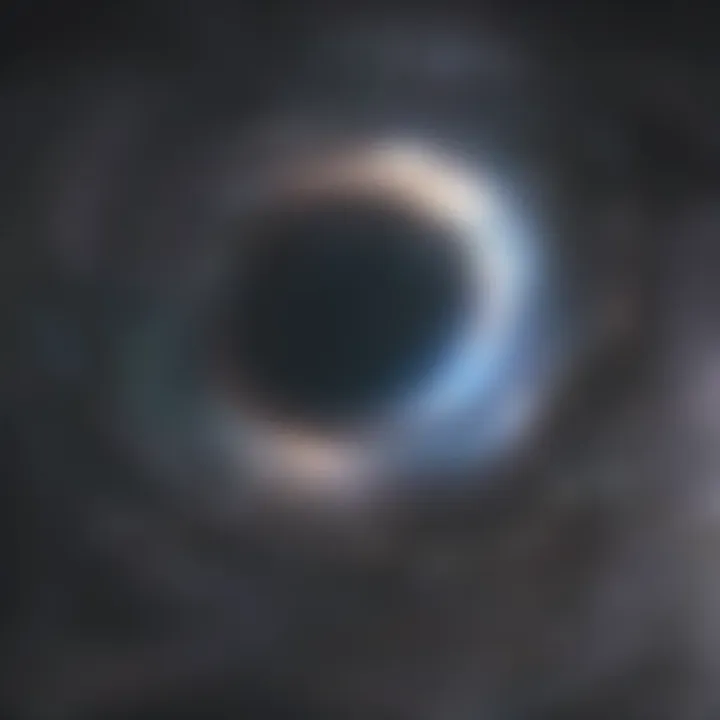
Black holes are not simply cosmic vacuum cleaners; they are architects of the universe, shaping the very fabric of space and time.
Through rigorous investigation of these dynamics, researchers can uncover the mysteries of the universe and contribute to the broader discussion on gravitational forces. Black holes remain pivotal in the quest to understand cosmic history and structure.
The Information Paradox
The Information Paradox poses significant questions about the nature of black holes and the fundamental principles of quantum mechanics and general relativity. Within this article, the discussion of the Information Paradox is essential, as it touches on the implications for our understanding of information conservation in the universe. Concepts related to the paradox challenge existing theories and push scientific inquiry further.
The paradox arises primarily from the observation that black holes seem to obliterate information about the matter that falls into them. According to classical physics, this information should not be lost, a principle rooted in quantum mechanics where information is preserved. Thus, the Information Paradox highlights a conflict between the predictions of general relativity and quantum mechanics.
Overview of the Paradox
The Information Paradox stems from Stephen Hawking's groundbreaking work on black hole thermodynamics. When a black hole evaporates due to Hawking radiation, it appears that the information about the matter that created the black hole is lost forever. This stands in direct opposition to quantum mechanics, which dictates that information can never truly be destroyed.
Many physicists grapple with the implications of this difference. A popular analogy illustrates the paradox: if a physical book is incinerated, the information it contained would be presumed lost. But in the quantum realm, this notion of loss conflicts with the idea that information can be recreated if it is fundamentally retained in the system.
Proposed Resolutions
Hawking Radiation
Hawking Radiation is a vital concept in black hole physics and provides a potential resolution to the Information Paradox. According to this theory, black holes can emit radiation due to quantum effects near the event horizon. As energy is released, the black hole loses mass and can eventually evaporate completely.
The key characteristic of Hawking Radiation is its prediction that black holes do not behave as absolute voids, instead, they slowly radiate energy away. This implies that information could be embedded in the radiation, hinting at a way to possibly retrieve what went into the black hole. This aspect makes Hawking Radiation a key theory in the resolution of the Information Paradox, as it provides a more optimistic viewpoint on the conservation of information.
Despite its potential, Hawking Radiation faces criticism. One disadvantage is that it operates on a level that is difficult to observe directly, which makes confirming its effects challenging. Additionally, if the radiation contains all the information when black holes evaporate, it raises further questions about how that information is stored and whether it maintains coherence with the quantum aspects of reality.
Black Hole Complementarity
Black Hole Complementarity is another intriguing perspective aimed at resolving the Information Paradox. This theory suggests that observers outside the black hole will see different information than those inside it. From an external viewpoint, the information is preserved on the event horizon, while for the infalling observer, it is subject to the extreme gravitational pull and massive time dilation.
The fundamental characteristic of Black Hole Complementarity is its duality concerning the perception of information. This makes it a conceptually powerful and widely discussed alternative solution to the paradox. It has gained attention because it allows for consistent interpretations from differing perspectives on the black hole's structure.
However, Black Hole Complementarity is not without its own complications. Its implications suggest a breakdown of locality and potentially further unresolved conflicts in understanding the fabric of spacetime. Critics argue that this idea challenges classical definitions of perception and reality in quantifying the information inherent within black holes.
In summary, the Information Paradox highlights crucial challenges in reconciling our understanding of black holes with fundamental laws of physics. It opens avenues for significant research and discussion. Hawking Radiation and Black Hole Complementarity are just two paths scientists are exploring in the quest to understand this profound issue.
Quantum Mechanics and Black Holes
Black holes present an intriguing intersection of general relativity and quantum mechanics, challenging our comprehension of the universe. This relationship between the vast gravitational forces of black holes and the often counterintuitive principles of quantum mechanics leads to some fascinating implications. Understanding this interplay is essential in grasping how we investigate the fundamental workings of nature.
Quantum mechanics introduces a set of principles that govern the behavior of matter and energy at the smallest scales. Integrating these principles within the context of black hole physics helps address many questions about the universe and the fate of information.
Hawking Radiation Explained
In 1974, Stephen Hawking introduced an idea that fundamentally transformed our understanding of black holes. His theory proposed that black holes are not entirely black. They can leak radiation, known as Hawking radiation, due to quantum effects near the event horizon. This radiation results from particle-antiparticle pairs that emerge just outside the black hole's event horizon.
When this happens, one particle may fall into the black hole, while the other escapes into space. The escaping particle appears as radiation. It implies that black holes can lose mass, leading to a phenomenon known as black hole evaporation. Over immensely long periods, a black hole could theoretically evaporate entirely.
Some significant aspects of Hawking radiation include:
- It suggests that black holes are not permanent fixtures in the universe.
- It raises fundamental questions about the conservation of information in the universe.
- This phenomenon has implications for the potential detectability of black holes through their radiation emissions.
Hawking’s insight forced physicists to reconsider the dynamics of black holes and the laws of thermodynamics applied to these cosmic objects. It also opened a debate around whether black holes can store information or simply erase it from existence.
Quantum Black Hole Models
Numerous theoretical frameworks aim to describe black holes through the lens of quantum mechanics. These models represent an effort to reconcile the apparent conflict between general relativity’s description of gravity and the inherently probabilistic nature of quantum mechanics. A few notable quantum black hole models include:
- Loop Quantum Gravity: This approach suggests that space-time itself has a quantized structure at the Planck scale. In this model, black holes do not represent an endpoint but rather, a process of transformation, possibly leading to new forms of matter and energy.
- String Theory: String theory posits that fundamental particles are one-dimensional objects called "strings." In this framework, black holes can be viewed as configurations of strings, which may help unify gravity with other fundamental forces.
- Black Hole Information Paradox: This paradox, stemming from Hawking radiation, questions whether the information that enters a black hole can ever be retrieved. Various models and hypotheses are under investigation to resolve this issue, which remains a significant question in theoretical physics.
Quantum black hole models offer potential pathways to understand the universe's deepest mysteries. Whether through predictions of particle behavior near a black hole or entirely new insights into the nature of space-time, these discussions are pivotal in modern physics.
The exploration of quantum mechanics and black holes not only deepens our understanding of the universe but also challenges the existing boundaries of scientific thought.
Future Directions in Black Hole Research
The study of black holes sits on the cutting edge of modern physics. Understanding black hole research is vital for several reasons. Firstly, it offers unique insights into the fabric of spacetime itself. Secondly, it may clarify fundamental aspects of quantum mechanics. Finally, it proposes to illuminate the mysteries surrounding cosmic evolution.
Emerging Technologies in Astronomy
The advancement of technology in astronomy is reshaping our understanding of black holes. Several innovative tools are now available to researchers. One significant development is the use of gravitational wave detectors like LIGO. This technology has already resulted in the first direct observation of a black hole merger, fundamentally changing our comprehension of the universe.
Additionally, observatories such as the Event Horizon Telescope (EHT) are creating unprecedented images of black holes. This collaborative effort bridges various disciplines, employing techniques from radio astronomy. The relevance of these technologies cannot be overstated. They are enabling scientists to gather data that was previously unattainable, leading to new theories and validation of existing ones.
Emerging technologies also include advanced computational models. Simulations can now create detailed predictions of black hole behavior under different conditions. These models are critical for understanding complex phenomena like accretion disks and relativistic jets. Given the rapid development pace, it seems highly likely that future innovations will further expand our knowledge.
Open Questions and Hypothetical Scenarios
Despite progress in understanding black holes, many questions linger in the scientific community. What happens at the core of a black hole? Is there a way to escape a black hole’s grasp? These questions prompt various hypothetical scenarios that drive research forward.
For instance, some scientists propose the existence of wormholes, which could serve as shortcuts in space-time that connect distant regions. Others are investigating the role of black holes in the early universe. These scenarios are more than mere speculation; they inspire rigorous theoretical work and experimental designs aimed at answering them.
Furthermore, the reconciliation of general relativity with quantum mechanics remains a significant challenge. If successful, it would not only solve many paradoxes related to black holes but could also lead to a fundamental shift in how we view the universe.
In summary, the future of black hole research relies heavily on technology and the exploration of uncharted scientific territories. As methods improve, so will our ability to unlock the secrets that black holes hold, reshaping our understanding of cosmic phenomena.