Exploring the Theory of Everything in Physics
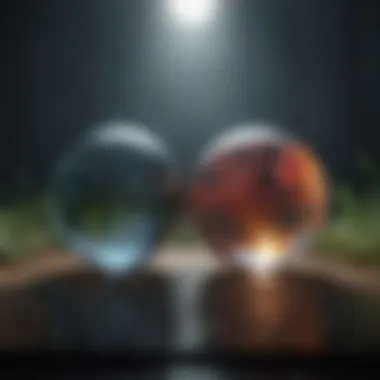
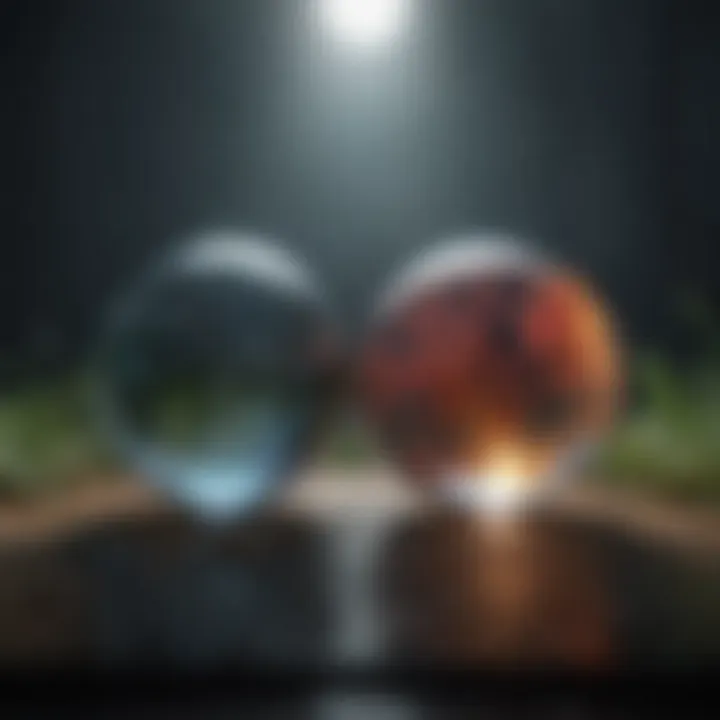
Intro
The quest for a unified understanding of the natural world leads physicists to explore the Theory of Everything (ToE). This ambitious theoretical framework aims to consolidate all fundamental forces and particles into a coherent model. It is, in essence, the Holy Grail of modern physics. Understanding the ToE has profound implications for our grasp of the universe and how the fundamental laws interact.
This article will cover key research findings, break down complex concepts, and provide an overview of the significance of the ToE. We aim to present a detailed and coherent narrative that can engage students, researchers, educators, and professionals alike in the intricate world of theoretical physics.
Key Research Findings
Overview of Recent Discoveries
In recent years, there have been substantial advancements in our understanding of fundamental forces. Researchers have made progress in areas such as string theory and quantum gravity. String theory proposes that the elementary particles we observe are not point-like dots but rather one-dimensional strings vibrating at different frequencies. This insight opens up new avenues in theoretical physics, inviting physicists to explore the connections between general relativity and quantum mechanics.
Additionally, findings from experiments at facilities like the Large Hadron Collider have provided critical data regarding the Higgs boson and its role in mass generation. Such discoveries are foundational to the broader search for a ToE.
Significance of Findings in the Field
These discoveries carry significant weight in theoretical physics. They push the boundaries of our knowledge and inspire new hypotheses. The interaction between various forces demonstrates the potential for a unified theory that could provide answers to some of the universe's most profound questions. The implications of a successful ToE extend beyond mere academic interest; they delve into philosophical considerations and the very fabric of reality.
"The search for a Theory of Everything is not just the pursuit of understanding nature but understanding our place within it."
Breakdown of Complex Concepts
Simplification of Advanced Theories
Understanding the Theory of Everything and its associated concepts can be daunting. Simplifying these ideas helps demystify the complexities involved. For instance, while string theory may sound abstract, its essence lies in recognizing that particles are manifestations of vibrating strings. Concepts such as dimensions beyond the familiar four (three spatial dimensions and one time dimension) can be grasped more easily when presented plainly.
Visual Aids and Infographics
Visual aids play a crucial role in translating complex theories into comprehensible formats. Infographics can illustrate the relationship between forces, particles, and how they interact. A diagram depicting the Standard Model of particle physics beside string theory could highlight the progression of thought in unifying theories.
To gain further insights into the Theory of Everything and recent advancements, resources such as Wikipedia's Theoretical Physics Section or Britannica's Physics Overview can provide valuable information.
Prelims to the Theory of Everything
Understanding the Theory of Everything (ToE) is pivotal for grasping the fundamental nature of the universe. This introduction serves as a gateway into a complex yet fascinating domain of physics. The very essence of the ToE lies in its ambition to combine all disparate physical phenomena into one cohesive framework. The ToE aims to provide a deeper insight into the interrelations among the forces and particles that constitute reality.
Definition of the Theory of Everything
The Theory of Everything can be defined as a theoretical framework that seeks to explain all known physical phenomena in a singular, all-encompassing model. Unlike individual theories that govern specific areas, such as general relativity or quantum mechanics, the ToE aspires to integrate these approaches into a unified description. Such a holistic understanding would not only answer lingering questions about how forces interact but also the fundamental fabric of space, time, and matter.
Historical Background
The quest for unification
The quest for unification arises from the desire to explain every aspect of physics under a single theory. Historically, physicists have noticed that the various forces in natureโgravitational, electromagnetic, weak, and strong nuclearโare interconnected. Each of these forces plays a role in the grand scheme of existence. Through time, this quest has evolved, gaining significance in the scientific community. The key characteristic of the quest for unification is its interdisciplinary nature. It draws from many fields, such as mathematics and cosmology, making it a prominent choice for this article. One unique feature is its potential to resolve longstanding contradictions between quantum mechanics and general relativity, presenting both advantages and challenges in our understanding of the universe.
Significant discoveries leading to the ToE
Various significant discoveries have paved the way for the development of the Theory of Everything. Innovations such as the discovery of the Higgs boson and advancements in string theory contribute crucial knowledge to the overarching goal of unification. The key characteristic of these discoveries is their ability to bridge gaps between different areas of theoretical physics. They are beneficial because they provide empirical evidence and theoretical tools to approach the ToE systematically. Not only do these breakthroughs deepen our understanding, but they also expose the limitations and challenges that have yet to be overcome in the pursuit of a comprehensive theory.
The value of the Theory of Everything lies in its potential to illuminate the underlying principles governing the universe.
Fundamental Forces in Physics
The concept of fundamental forces serves as a cornerstone of physics, forming the backbone of understanding interactions in the universe. These forces govern everything from the smallest particles to the largest celestial bodies. A Theory of Everything, or ToE, aims to integrate these disparate forces into a cohesive framework. Understanding these forces is crucial to unify our knowledge of physics under a single theoretical umbrella.
Key elements of Fundamental Forces:
- Gravitational Force: It is the oldest known force, governing the motion of celestial bodies.
- Electromagnetic Force: This force plays a significant role in atomic structures and chemical reactions.
- Weak Nuclear Force: It is fundamental in processes like beta decay in nuclear physics.
- Strong Nuclear Force: It binds protons and neutrons together in an atomic nucleus.
Evaluating these forces reveals both their individual significance and the potential for a unifying theory. Each force operates on different scales and manifests unique properties, influencing research and technology.
Gravitational Force
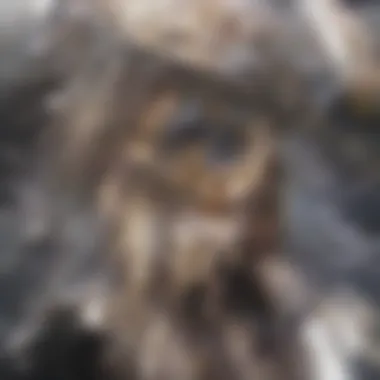
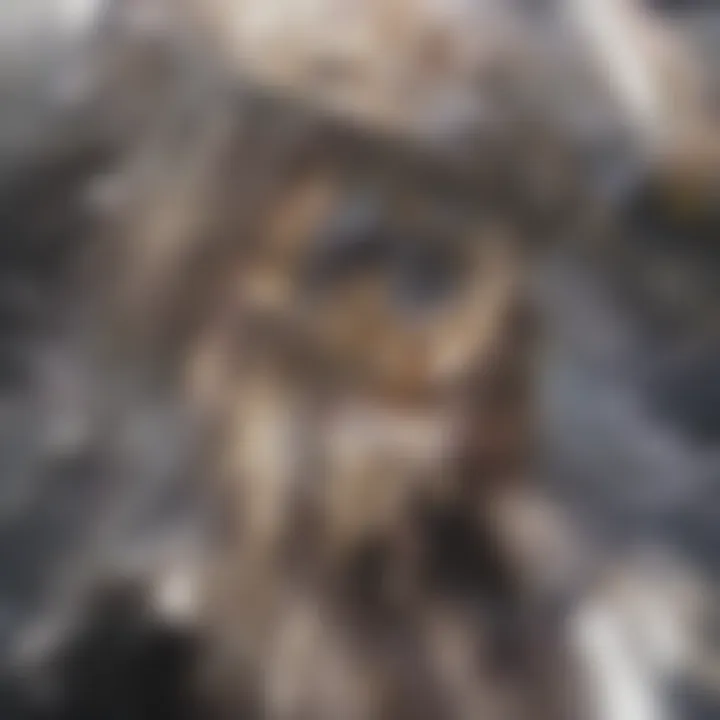
The gravitational force is often perceived as a familiar force that influences everyday life. It governs the attraction between masses, such as planets, stars, and galaxies. Isaac Newton laid the groundwork for understanding gravity by formulating the law of universal gravitation. In contrast, Albert Einstein expanded the concept through his theory of general relativity, showcasing gravity as a curvature of spacetime.
The implications of gravitational interactions present deep insights into the structure of the universe. For example, gravitational waves, predicted by Einstein, represent ripples in spacetime produced by massive objects in motion. Their detection has opened new avenues for astronomical observation, connecting this force to the greater quest for a ToE.
Electromagnetic Force
The electromagnetic force occupies a distinct role in physics, responsible for electric and magnetic interactions. It affects charged particles, playing a central part in chemical bonding and electrical phenomena. James Clerk Maxwell's equations fused electricity and magnetism into a single theory of electromagnetism, transforming our understanding of physical laws.
The electromagnetic force acts over vast distances but is much stronger than gravity at the atomic scale. It is essential in both classical physics and modern applications, including quantum mechanics. This duality is pivotal to synthesizing different areas of the ToE.
Weak Nuclear Force
The weak nuclear force operates at a very short range, crucial for processes like nuclear decay. It is responsible for interactions that change one type of subatomic particle into another. Its discovery was instrumental in developing the electroweak theory, which further unified two fundamental forces.
Understanding the weak force is necessary for exploring beyond the standard model of particle physics. It challenges our conventional views of symmetry and conservation laws, suggesting deeper layers of physical reality.
Strong Nuclear Force
Finally, the strong nuclear force holds the nucleus of an atom together, counteracting the electromagnetic repulsion between protons. This short-range force binds quarks within protons and neutrons, essential for the stability of atoms. Quantum chromodynamics describes this phenomenon, illustrating the complexity of interactions at the subatomic level.
The strong nuclear force underpins nuclear fission and fusion, processes fundamental to energy production. Its study is significant for both theoretical understanding and practical application in fields like nuclear energy.
In summary, understanding the fundamental forces is essential for anyone delving into the Theory of Everything. These forces illuminate the intricacies of reality, laying a foundation for future theoretical advancements.
The Role of Quantum Mechanics
Quantum mechanics plays a pivotal role in the ongoing quest for a Theory of Everything (ToE). It provides the foundational framework that underlines the behavior of matter and energy at the microscopic level. The principles derived from quantum theory offer insights into the interaction of particles, which is essential for integrating both quantum mechanics and general relativity into a single coherent theoretical system. Understanding these interactions is vital because it lays the groundwork for unifying the fundamental forces of nature, moving us closer to achieving a ToE.
Principles of Quantum Theory
Quantum theory operates on several core principles that challenge classical intuitions about physics. Firstly, the concept of quantization refers to the idea that certain properties, such as energy, can only take on discrete values rather than any continuous value. This principle is illustrated in the behavior of electrons within an atom; they occupy specific energy levels and can only transition between these levels by absorbing or emitting quantized amounts of energyโphotons.
Another notable principle is wave-particle duality, which asserts that particles, like electrons and photons, exhibit both wave-like and particle-like properties. This duality complicates our understanding of particles and significantly informs theories exploring unification. Furthermore, the principle of superposition states that particles can exist in multiple states at once until measured, leading to outcomes that emphasize probabilistic rather than deterministic behavior, which has deep implications for understanding reality at a fundamental level.
Lastly, the uncertainty principle, formulated by Werner Heisenberg, states that certain pairs of physical properties cannot be simultaneously known with arbitrary precision. For example, one cannot precisely know both the position and momentum of a particle. This emphasizes the limitations of observation in quantum mechanics and fundamentally challenges classical notions of determinism.
Quantum Field Theory
Quantum Field Theory (QFT) represents a significant advancement in theoretical physics. It merges quantum mechanics with special relativity, treating particles as excited states of underlying fields. Each fundamental particle is thus understood as a vibration within its respective field. For instance, photons are excitations of the electromagnetic field, while electrons are excitations of the electron field. This provides a more nuanced understanding of interactions at deeper levels.
QFT is crucial for analyzing the fundamental forces, especially electromagnetic and nuclear forces. The development of QFT has also resulted in the Standard Model of particle physics, which successfully classifies all known elementary particles and describes their interactions through force carriers, such as bosons. However, QFT does not incorporate gravity, which remains a significant gap in the journey towards a ToE.
String Theory as a Candidate
String theory is a prominent candidate in the quest for a Theory of Everything (ToE) in physics. It seeks to provide a unified framework that can reconcile the apparent discrepancies between quantum mechanics and general relativity. This approach posits that the fundamental constituents of the universe are not zero-dimensional point particles, but rather one-dimensional strings.
When strings vibrate, they produce different particles based on their vibrational modes. This is significant as it implies a deeper connection between various particles in the universe, suggesting that they may not be distinct entities, but variations of a singular underlying principle. This notion challenges conventional particle physics and encourages a re-evaluation of how we understand matter and forces.
Basic Concepts of String Theory
Three essential concepts form the foundation of string theory:
- Strings and Dimensions: In string theory, all particles are manifestations of tiny vibrating strings. The theory typically requires extra spatial dimensions, often beyond the familiar three. For instance, in many string theories, there exist ten or eleven dimensions. These additional dimensions could explain various forces and particles in our universe.
- Supersymmetry: This concept proposes a symmetry between fermions and bosons, two categories of particles. Supersymmetry predicts that for every known particle, there exists a partner particle, which has not been observed yet. This aspect of string theory draws attention, as it could explain mysterious issues such as dark matter.
- M-Theory: A proposed extension of string theory, M-theory suggests that strings are not the fundamental components but are part of higher-dimensional objects called branes. M-theory unites different string theories under one framework, offering a broader perspective on the universe's structure.
These concepts together create a more consolidated understanding of particle interactions and fundamental forces.
Implications for the ToE
The implications of string theory for the Theory of Everything are profound:
- Unification of Forces: String theory has the potential to unify all fundamental forces. Unlike traditional models that treat forces separately, string theory offers a holistic view where gravitational, electromagnetic, and nuclear forces are woven into the same fabric.
- Quantum Gravity: One of the primary challenges in theoretical physics is reconciling general relativity, which describes gravity, with quantum mechanics, which explains the behavior of particles at the smallest scales. String theory attempts to bridge this gap, providing insights into how gravity operates on quantum scales.
- Cosmic Consistency: If string theory is correct, it provides a consistent framework to describe various cosmic phenomena, including black holes and the early universe. This could lead to a more comprehensive understanding of cosmic evolution and structure.
String theory represents an ambitious attempt to decode the fundamental nature of reality through a single theoretical construct. Its implications extend well beyond particle physics.
In summary, string theory stands as a formidable candidate in the search for a Theory of Everything. By proposing radical new ideas about the universe's building blocks and their interactions, it opens pathways to unprecedented discoveries in modern physics.
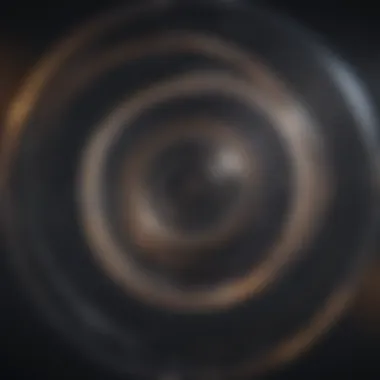
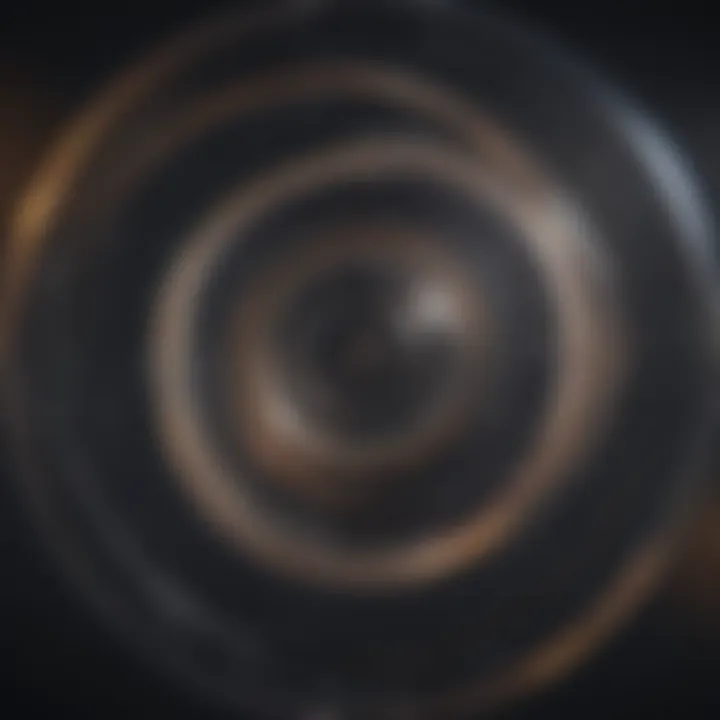
Loop Quantum Gravity
Loop Quantum Gravity (LQG) stands as a significant theoretical framework in the ongoing quest for a Theory of Everything (ToE). This approach attempts to reconcile the principles of general relativity with quantum mechanics without requiring the additional dimensions that string theory proposes. Instead, Loop Quantum Gravity works directly with the fabric of spacetime itself, suggesting that space is quantized. This means that, at the smallest scales, space is not continuous but made up of discrete units.
The importance of Loop Quantum Gravity lies in its ability to provide a potential solution to some of the most pressing problems in theoretical physics, particularly regarding black holes and the early universe. Understanding how gravity operates at the quantum level could lead to insights about the big bang and possibly the fundamental nature of reality. Furthermore, its distinct approach of focusing on the geometrical properties of spacetime differentiates it from other competing theories, notably string theory.
Overview of Loop Quantum Gravity
Loop Quantum Gravity arose from attempts to quantize general relativity while respecting its principles. It formulates spacetime not as a smooth continuum but as a network of finite, interconnected loops. These loops correspond to the quantized units of space, termed "spin networks." The dynamics are described via a mathematical framework called loop variables.
This approach suggests that spacetime possesses a granular structure, which refines our understanding of gravitational interactions. Some key features of LQG include:
- Discrete Spacetime: LQG introduces a model where spacetime is made up of discrete building blocks, altering the traditional view and introducing profound implications for cosmology and black hole physics.
- Preservation of Background Independence: Unlike string theory, which relies on an underlying geometric backdrop, LQG maintains background independence, aligning closely with Einsteinโs original formulation of general relativity.
- Predictive Power: LQG offers specific predictions about the behavior of gravitational waves and could provide insights into how black holes may ultimately evaporate as proposed by Stephen Hawking.
Comparison with String Theory
Loop Quantum Gravity and string theory represent two leading approaches to unifying physics, each with its distinct methodologies and implications. When comparing these theories, several key aspects arise:
- Nature of Fundamental Entities: In string theory, the fundamental entities are one-dimensional strings that vibrate at different frequencies, giving rise to the various particles. LQG, on the other hand, focuses on the quantized loops of spacetime.
- Dimensionality: String theory requires additional dimensions beyond the familiar fourโthree of space and one of timeโto function mathematically. LQG operates within the established four-dimensional framework, attempting to unify gravitational and quantum phenomena without the need for extra dimensions.
- Mathematical Complexity: String theory is noted for its intricate mathematical structures and wide-ranging implications, including dualities and certain symmetry properties. LQG, appearing simpler in some aspects, faces challenges in generating testable predictions, a critical factor in the scientific method.
The comparison is complex and ongoing, with proponents of each theory highlighting strengths and drawbacks. Some physicists advocate for a combined understanding that could incorporate elements from both frameworks into a more holistic unification.
"The pursuit of a Theory of Everything requires a harmony of approaches; string theory and loop quantum gravity may not be opposing views but rather complementary paths toward understanding the universe."
Current Challenges in Developing a ToE
The pursuit of a Theory of Everything (ToE) is not merely an academic endeavor; it encapsulates the very essence of physics' ambition to unify our understanding of the universe. However, the journey toward this grand unification is fraught with challenges that extend across both experimental and conceptual realms. Understanding these challenges is crucial for appreciating why physicists have yet to arrive at a universally accepted ToE. In this section, we will explore the specific obstacles that hinder progress in this field, emphasizing their significance.
Experimental Limitations
Experimental limitations present one of the most significant challenges in the quest for the ToE. Modern physics relies heavily on empirical verification of theories, yet many aspects of theoretical physics remain beyond current experimental reach. Notably, high-energy particle collisions, necessary for testing theories like string theory and loop quantum gravity, require particle accelerators of immense size and cost, like the Large Hadron Collider. The energy scales at which potential unifying forces might operate are typically much higher than what we can achieve with present-day technology.
Moreover, phenomena associated with quantum gravity, such as black holes or the early universe, are not replicable in laboratory settings. This leads to a lack of direct observational evidence that could support or refute specific theories. Consequently, the inability to perform extensive experiments limits the ability of physicists to examine the tenets of a ToE comprehensively, resulting in speculative theories that may lack the rigor demanded by scientific inquiry.
"Experimental evidence is the backbone of any scientific theory, and without it, theories about the ToE may remain mere abstractions."
Conceptual Issues
In addition to experimental hurdles, conceptual issues loom large in the theoretical landscape of physics. One prominent challenge is reconciling general relativity with quantum mechanics. General relativity describes gravity as a curvature of spacetime caused by mass, while quantum mechanics governs the behavior of particles at microscopic scales. These two frameworks, while successful within their respective domains, offer conflicting descriptions of natural phenomena, creating a fundamental incompatibility that presents a major hurdle in constructing a cohesive ToE.
Furthermore, the very nature of what constitutes a theory of everything is itself debatable. For example, various interpretations of quantum mechanics exist, each offering different views on determinism and randomness. This raises questions about whether a single, all-encompassing theory can exist or if multiple theories can coexist. The philosophical implications of such distinctions complicate the search for a unified framework, as physicists must grapple with not just mathematical consistency but the ramifications of the theories they propose.
The journey toward a ToE requires rigorous exploration of these experimental limitations and conceptual issues. Each step taken in the pursuit, despite setbacks, provides invaluable insights into the nature of the universe.
Philosophical Implications of a ToE
The Theory of Everything (ToE) has implications that extend beyond physics and into the philosophical realm. Understanding the ToE helps us reexamine fundamental questions about existence, the nature of the universe, and our place within it. This section emphasizes the intersection of physics and philosophy, offering insights into how a successful ToE could reshape our worldviews.
The Nature of Reality
One significant implication of developing a ToE is the way it could redefine our understanding of reality itself. If the ToE successfully unifies all fundamental forces and particles, this would suggest a single, cohesive reality underlying everything we observe. This concept challenges traditional notions of separateness in the universe. Instead of viewing forces and particles as disparate entities, a ToE could propose that everything is interconnected at a fundamental level.
This perspective can lead to questions about the nature of reality:
- Is reality objective, existing independently of human perception?
- Or is it subjective, shaped by our observations and thoughts?
These questions are crucial because they influence how we approach scientific inquiry and philosophical exploration.
"Understanding our universe's fundamental nature has always been intertwined with both scientific and philosophical discourse."
Determinism vs. Indeterminism
The concept of a ToE also invites a reconsideration of determinism and indeterminism in scientific thought. In classical physics, determinism suggests that every event or state of being can be precisely predicted based on prior conditions. If a ToE were to emerge from frameworks like string theory or loop quantum gravity, it could imply an underlying order governing physical phenomena.
However, quantum mechanics introduces elements of indeterminism, suggesting that not all events are predictable. The philosophical debate about whether the universe is fundamentally deterministic or indeterministic becomes central in discussions related to the ToE.
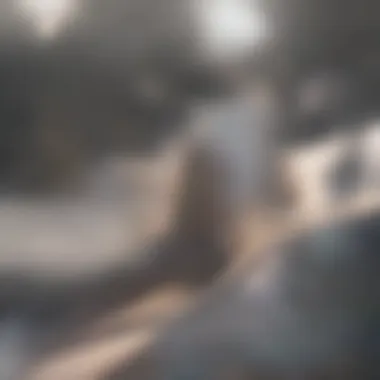
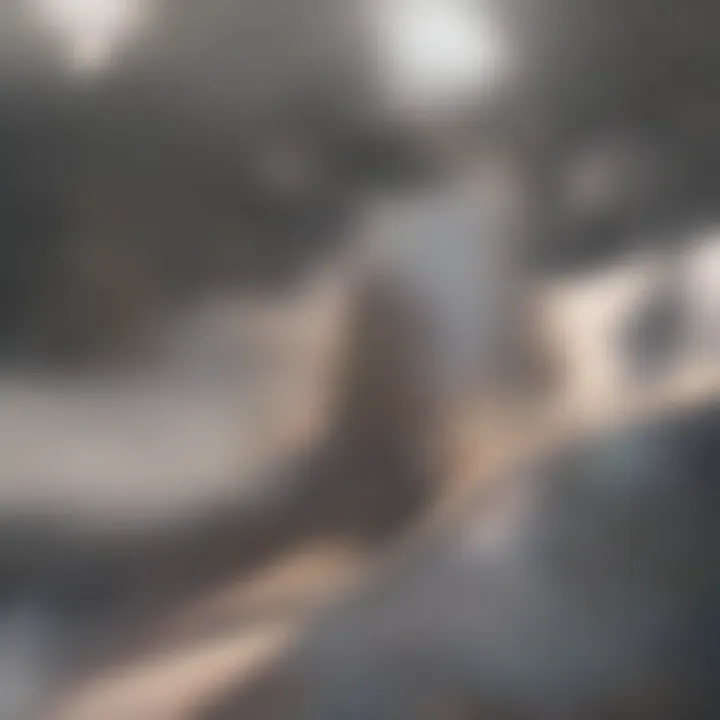
This leads to further inquiries:
- If determinism holds, what does that say about free will and our choices?
- Conversely, if indeterminism prevails, what are the implications for chaos and unpredictability in nature?
Understanding these implications is vital for those in scientific and philosophical fields, as they shape many areas of inquiry and thinking.
Potential Impact of a Successful ToE
The Theory of Everything (ToE) stands as one of the most ambitious goals within physics. This endeavor aims to forge a unified understanding of the fundamental forces and particles that constitute the universe. A successful ToE would not merely be a triumph of theoretical physics; it would have profound implications across various domains, affecting technology, philosophy, and other scientific disciplines.
Advances in Technology
Achieving a ToE would facilitate advancements in technology that are difficult to predict fully. By tying together knowledge of the fundamental forces, physicists could engineer new materials or devices that harness quantum phenomena. For instance, the insights gained from unifying electromagnetic and gravitational forces might lead to significant innovations in energy generation. Theoretical constructs could enable the development of more efficient solar panels or even new forms of energy storage.
- This potential includes:
- Enhanced computational technologies derived from quantum mechanics.
- Development of nanotechnology with applications in medicine and materials science.
- Potential breakthroughs in telecommunications through better understanding of information transfer at the quantum level.
Though these ideas are speculative, the unification of physical laws lays the foundational work necessary for future technologies.
Influence on Other Scientific Fields
A successful Theory of Everything would ripple through multiple scientific disciplines. It offers a framework that could enrich our understanding of not only physics but also biology, chemistry, and cosmology. For biology, the principles underlying fundamental forces could provide insights into processes at cellular levels and influence how we understand factors in life at a quantum scale.
- Consider the following impacts:
- Chemistry: Improved theoretical models could lead to more efficient chemical reactions and materials.
- Astronomy: Insights into cosmic phenomena could enhance our understanding of black holes and dark matter.
- Sociology: Philosophical implications regarding the nature of reality might shift discussions about determinism and free will based on scientific findings.
Overall, the connectivity between disciplines would likely lead to interdisciplinary collaboration that fosters innovation, therefore, altering our approach to science holistically.
"The pursuit of a unified theory not only sharpens our understanding of the universe but also redefines our approach to knowledge itself."
The impact of a successful ToE extends far beyond physics; it holds the potential to reshape our technological landscape and the very nature of scientific inquiry.
Future Directions in the Search for a ToE
The quest for a Theory of Everything (ToE) is a central ambition in modern physics. As we look to the future, numerous pathways for exploration and experimentation offer insight into the multifaceted nature of the universe. This section examines critical areas in the pursuit of a unifying framework, highlighting both upcoming experiments and theoretical developments that could shape our understanding of fundamental forces and particles.
Upcoming Experiments
Scientific inquiry driven by experimentation is crucial for progress in theoretical physics. Some upcoming experiments are particularly noteworthy:
- Large Hadron Collider (LHC) Upgrades: The LHC is poised for renovations that will enhance its collision energy and improve detector capabilities. This will allow physicists to conduct more detailed studies of particle interactions, which may unveil new physics beyond the Standard Model.
- Space-based Observatories: Missions such as the NASAโs James Webb Space Telescope and various gravitational wave observatories will provide data that could confirm or challenge quantum gravity theories. Understanding cosmic phenomena at larger scales helps to bridge gaps in known physics.
- Neutrino Experiments: Innovations in neutrino detectors, such as the IceCube Neutrino Observatory, could offer insights into the role of neutrinos in determining the universe's fundamental structure.
These experiments could lead to findings that fundamentally alter our understanding of nature and validate or refute existing ToE theories.
Theoretical Developments
In parallel with experiments, significant theoretical frameworks are advancing, potentially contributing to a successful ToE:
- Causality in Quantum Gravity: Researchers are exploring how spacetime itself influences quantum phenomena. A new understanding of causality may bridge the gap between general relativity and quantum mechanics.
- Emergent Gravity: Some theorists propose that gravity might be an emergent property rather than a fundamental force. This could rewrite our understanding of the universe's fabric and its fundamental interactions.
- Mathematical Innovations: The use of advanced mathematics, such as category theory, is being explored to construct more robust frameworks. These tools could synthesize previously disparate theories and lead to a unified approach in understanding fundamental forces.
The exploration of these theoretical avenues will not only inform the search for a ToE but may also redefine our understanding of physics itself.
"The true joy of discovery lies not in finding answers, but in expanding the questions that guide our inquiry."
In summary, progress toward a Theory of Everything relies on both experimental and theoretical innovations. As physicists push the boundaries of knowledge, the potential to unlock the mysteries of the universe becomes ever more attainable.
Culmination
Summary of Key Points
- Definition: The ToE seeks to provide a singular framework that explains all fundamental forces and particles.
- Historical Background: The quest for unification has its roots in early physics, with significant milestones contributing to the ongoing pursuit.
- Fundamental Forces: Gravitational, electromagnetic, weak nuclear, and strong nuclear forces are central to our understanding of physical interactions.
- Quantum Mechanics: The role of quantum theory is crucial, introducing concepts foundational to modern physics.
- String Theory and Loop Quantum Gravity: These are two leading candidates proposed as potential frameworks for a ToE, each with unique implications and challenges.
- Challenges: Experimental limitations and conceptual issues continue to hinder progress in the quest for a ToE.
- Philosophical Implications: The potential unification raises questions about reality, determinism, and the nature of knowledge itself.
- Future Directions: Ongoing experiments and theoretical developments will shape the next steps in this pursuit, with hope for breakthroughs that may fundamentally alter our understanding of the cosmos.
Final Thoughts
The implications of successfully formulating a Theory of Everything are profound. It could lead to dramatic advances in technology and influence other fields of science. The act of pursuing such a comprehensive understanding pushes the boundaries of human knowledge, compelling us to reevaluate our understanding of the universe. Ultimately, the journey towards a ToE is as significant as the goal itself, serving as a testament to human curiosity and the relentless quest for knowledge.
"The pursuit of knowledge is more precious than its possession."
As we look forward, the hope remains that one day, the complexities of our universe will be revealed in a unifying theory that explains not just how the universe works, but why it exists as it does.