Exploring the Second Law of Thermodynamics
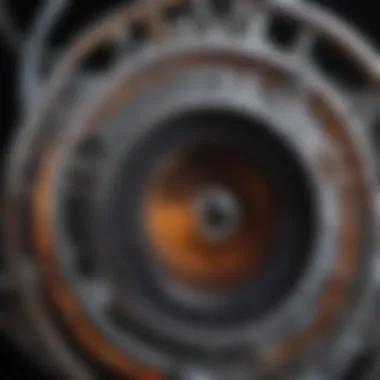
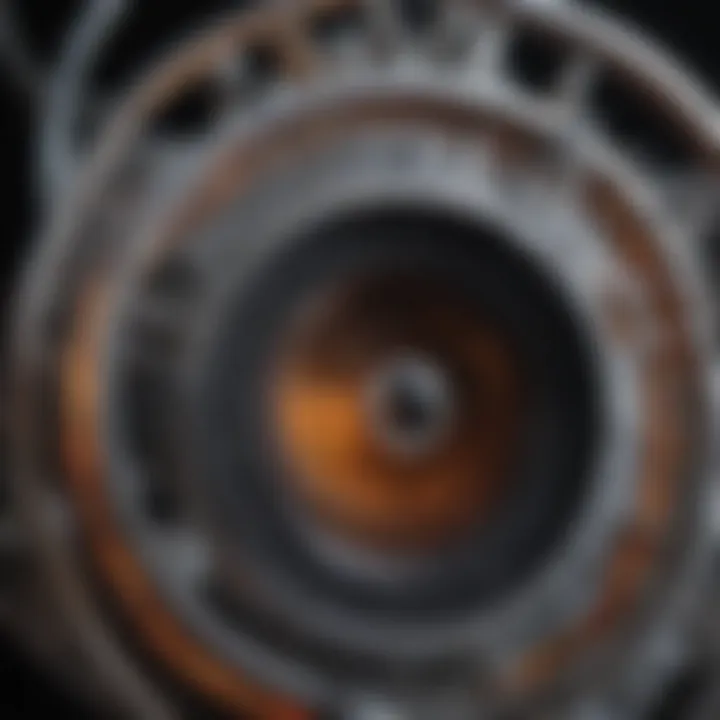
Intro
The Second Law of Thermodynamics stands as a pivotal concept in understanding the natural world. At its core, this law governs how energy is transformed and flows through systems. It introduces the idea of entropy—a measure of disorder that tends to increase over time in isolated systems. This principle not only affects the realm of physics but extends its tentacles into other fields like chemistry, biology, and even economics. In light of this, it is crucial to dig into what these concepts mean and how they apply in real-world settings.
This article aims to break down the complexities of the Second Law, transforming what may seem like an abstract theoretical concept into something tangible and practical. Whether you are dipping your toes into this subject out of sheer curiosity or are a seasoned scholar seeking deeper insights, you'll find valuable information tailored just for you.
Let’s embark on this journey by discussing key research findings that have shaped our understanding of the Second Law.
Prologue to Thermodynamics
Thermodynamics isn’t just a branch of physics; it’s the backbone of understanding how energy interacts within our universe. From the engines that run our cars to the processes that sustain life itself, the principles of thermodynamics are everywhere. This section aims to lay a solid foundation for those diving deeper into the fascinating realm of energy transformations and their implications.
One cannot underestimate the importance of mastering the fundamentals of thermodynamics. This knowledge serves as a stepping stone not only for advanced scientific inquiry but also for practical applications in engineering, environmental science, and even philosophy. Consequently, this article will unravel the intricacies of the Second Law of Thermodynamics, providing a comprehensive perspective on its relevance and applications.
Understanding Thermodynamic Laws
Thermodynamics consists of a series of laws that govern energy and its transformations. Here are the primary laws to consider:
- Zeroth Law: Defines thermal equilibrium and compels us to think about temperature in a more consistent way.
- First Law: States that energy cannot be created or destroyed; it can only change forms—a fundamental concept known as the law of conservation of energy.
- Second Law: Addresses the direction of energy transfers and the concept of entropy.
- Third Law: Discusses absolute zero and how entropy behaves when approaching this temperature.
Understanding these laws provides a structured backdrop for analyzing more complex phenomena in science and engineering. Especially, the Second Law surfaces as a pivotal element in discussions about energy dissipation and the irresistible rise of entropy.
The Role of Energy in Thermodynamics
Energy is the linchpin in thermodynamic systems. Without it, there would be no heat engines, no chemical reactions, and certainly no biology. Here are some key elements to note:
- Forms of Energy: Energy exists in various forms—kinetic, potential, thermal, chemical, and more. Each form plays a unique role, contributing to how processes unfold in nature.
- Energy Transfer: Energy can flow from one body to another, be it through heat transfer, work done, or wave propagation. The manner in which energy is exchanged can radically alter a system’s state.
- Efficiency: The efficiency of any thermodynamic system is shaped by optimizing energy transfers, keeping in mind that some energy will always dissipate—commonly as heat due to the Second Law.
Understanding these dynamics is crucial as it directs our approach to everything from crafting better energy-efficient devices to contemplating broader environmental impacts. The intricacies of energy within thermodynamics set the stage for a multitude of real-world applications, as explored further throughout this article.
The Second Law Unpacked
Understanding the Second Law of Thermodynamics is like opening a door to a grand hall filled with the intricacies of energy flow and transformation. This law is essential not just in physics but resonates across various fields—from chemistry to engineering and even biology. Its implications stretch far beyond academic circles, prompting us to ponder fundamental questions about existence and the nature of our universe.
The importance of unpacking this law lies in its ability to inform us about natural processes. While energy can change forms, it does not create or destroy itself. This law emphasizes the inherent constraints on energy transformations, shaping how we design systems and understand phenomena around us. Comprehending these principles is vital, especially in today's sustainability-driven world.
Defining the Second Law
When we talk about the Second Law of Thermodynamics, we’re tackling a concept that relates to the direction of heat transfer and the spontaneity of processes. It states that in any energy exchange, if no energy enters or leaves the system, the potential energy will always increase. In more plain terms, heat will not flow spontaneously from a colder body to a hotter one.
The law also correlates with the idea of entropy, a measure of disorder or randomness in a system. As energy transitions occur, systems lean toward greater disorder. This means that while energy may change forms, its quality diminishes, and unusable energy increases. This is evident in natural processes like the formation of ice cubes floating in a warmer glass of water: inevitably, the symmetry of the ice succumbs to the chaos of surrounding thermal energy.
Key Concepts of Entropy
Entropy is often the star of the show when discussing the Second Law. It's a central theme that reflects how energy systems evolve over time. The idea of entropy can feel a bit abstract, yet it has concrete manifestations. For example, consider your room when left unattended; over time, it may become cluttered and chaotic without any external effort to organize it.
Here’s what you need to grasp about entropy:
- Definition: Entropy quantifies the amount of disorder within a system.
- Irreversibility: Once entropy increases within a closed system, it’s challenging, if not impossible, to revert to a lower state of disorder without external intervention.
- Statistical Mechanics: Entropy can be understood through macrostates and microstates, where a macrostate may represent a condition of greater disorder with many possible microstates corresponding to it.
Entropy doesn't just apply to systems in isolation but influences everything around us—decay of organic matter, aging of living organisms, and even human-made processes such as manufacturing. It pushes us to consider that energy transformations are indeed directional, firmly rooted in statistical probability.
Reversible and Irreversible Processes
The concepts of reversible and irreversible processes are fundamental when delving into the practical implications of the Second Law. A reversible process is one where both the system and surroundings can return to their original states without any net change in the universe. Sadly, in practice, these processes rarely occur, chiefly because real-world systems have friction, turbulence, and other inefficiencies—all elements that disrupt reversal.
Irreversible processes are more commonplace. When you mix cream into coffee, for instance, the process is irreversible since you cannot un-mix them without some elaborate method. This isn’t just about mixing; it’s reflective of the continual increase in entropy and inevitable degradation of energy quality.
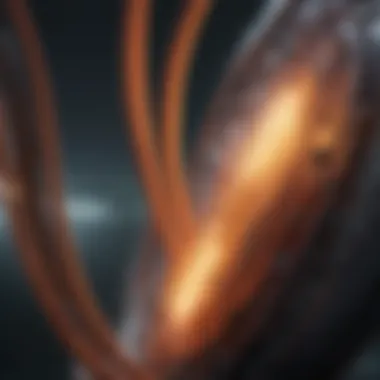
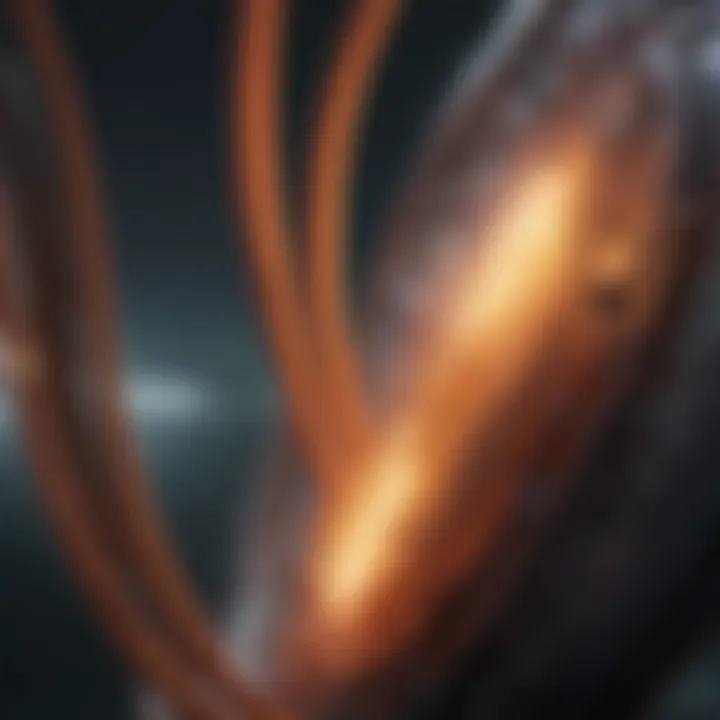
To summarize:
- Reversible processes are ideally efficient, theoretically allowing energy to preserve its utility.
- Irreversible processes reflect real-world interactions, resulting in a net increase of entropy and energy dispersal.
In essence, the Second Law encapsulates a fundamental aspect of nature: the journey towards disorder and energy dissipation is not just a possibility; it's the rule.
Grasping the Second Law and its associated concepts serves as groundwork not only for students and scholars but for anyone curious about the cosmic dance of energy and entropy that fundamentally shapes our universe.
Entropy: A Central Theme
In the realm of thermodynamics, entropy stands as a linchpin, a concept that captures the essence of disorder and randomness within physical systems. Its significance stretches far beyond mere equations; it is a concept rooted deeply in the fabric of how matter and energy interact. Understanding entropy is crucial when examining the second law of thermodynamics, as it delineates what is possible within a system and constraints closure on energy interactions.
Entropy operates like a compass, guiding researchers and professionals across various fields. From physics to engineering, and even environmental science, the implications of entropy are profound. It shapes the way we comprehend everything from the heat engines that drive our cars to the biological processes essential for life. By evaluating the changes in entropy, one can derive critical insights regarding the efficiency and feasibility of processes.
Entropy in Isolated Systems
In the context of isolated systems, where no energy or matter is exchanged with the environment, entropy has a rather intriguing behavior. As per the second law, the total entropy of an isolated system can only remain constant or increase over time; it never decreases. This characteristic serves as a clarion call for researchers attempting to understand the directionality of physical processes.
When considering a cup of coffee left out to cool, a clear example emerges. At first, the coffee is hot; however, over time, heat dissipates into the surroundings. This leads to an increase in the entropy of the system, demonstrating how the energy becomes more dispersed. For an isolated system, this transformation is irreversible, reflecting the inherent order transitioning into disorder – a principle that shares profound implications not only in everyday life but also in various natural phenomena.
"The entropy of an isolated system tends to increase over time, leading to a state of thermodynamic equilibrium, where no net energy flow occurs."
To break it down further:
- Irreversibility: The natural trend towards higher entropy reflects the preferred direction of time – a simple yet powerful notion.
- Equilibrium: Once a system reaches maximum entropy, it is deemed to be at equilibrium. This state reflects a balance that results in no changes over time.
Macrostates and Microstates
Delving into macrostates and microstates enriches our understanding of entropy further. A macrostate describes the macroscopic properties of a system, such as temperature, pressure, and volume, while microstates represent the different ways these macroscopic states can be realized at the particle level.
Imagine a box filled with gas molecules. The arrangement and movement of these molecules can lead to countless microstates, each contributing to the same macrostate of pressure and temperature. Hence:
- A macrostate can encompass many microstates. For instance, a balloon filled with air can be in various configurations at the molecular level while maintaining a constant internal pressure.
- The more microstates available for a given macrostate, the higher the entropy. This explains why gases, having abundant microstates, possess higher entropy compared to solids, where particle arrangement is much more rigid.
To summarize, acknowledging the distinction between macrostates and microstates provides a deeper appreciation for entropy. These concepts not only inform theorists and experimentalists alike but also clarify everyday observations in thermodynamics by framing our understanding of disorder on both large and small scales.
Applications of the Second Law
The Second Law of Thermodynamics is more than just a scientific principle; it plays a pivotal role in the practical applications that influence our daily lives. Understanding this law enables us to optimize energy efficiency, innovate in technology, and appreciate the natural world. By recognizing the implications of the Second Law, we can comprehend not only how energy transitions between forms but also the consequences of these transformations.
Thermodynamic Cycles
Thermodynamic cycles are crucial applications that exemplify the Second Law in action, especially in engines and refrigeration processes. A thermodynamic cycle involves a series of transformations where a working substance moves through various states, absorbing and releasing energy in the process. The most common examples include the Carnot cycle, Rankine cycle, and Otto cycle.
- Carnot Cycle: The epitome of efficiency. This theoretical model sets the upper limit of efficiency for any thermodynamic cycle, emphasizing the importance of temperature differences and reversible processes. Although unattainable in real-world scenarios, it serves as a benchmark for evaluating real engines.
- Rankine Cycle: Predominantly used in power plants, this cycle includes boiling the working fluid to create steam that drives a turbine, then condensing the steam back into a liquid. Each phase reflects changes that align with the Second Law, where entropy tends to increase within the system.
- Otto Cycle: This cycle is crucial for gasoline engines, featuring both adiabatic and isochoric processes. Despite its widespread use, losses such as heat dissipation limit its efficiency, aligning perfectly with the law's implications on inevitable energy waste.
Using these cycles gives engineers and designers tools to develop highly efficient machines. Lessons learned from enhancing these cycles reflect directly back to the core concepts of energy conservation and entropy management, demonstrating their importance in modern technology.
Heat Engines and Refrigerators
Heat engines and refrigerators are two sides of the same coin when utilizing the principles of the Second Law. On one hand, we have heat engines, which aim to convert heat energy into mechanical work. The very nature of these engines is rooted in the fact that not all input energy can be transformed into useful work. The waste heat generated during the process is a necessary consequence of the Second Law, which states that entropy will always increase in a closed system.
- Heat Engines are commonly found in our everyday lives—from cars to power plants. These machines follow the thermodynamic cycles mentioned earlier to produce work, often using fuels or renewable sources. Their efficiency is a key area of research, as society aims for sustainable energy solutions.
Conversely, refrigerators work by extracting heat from a cold reservoir and transferring it to a hot reservoir, contrary to the natural flow of heat dictated by the Second Law. They consume energy to accomplish this, and their operational efficiency hinges on minimizing energy input while maximizing heat removal. Refrigerators, air conditioners, and even heat pumps employ similar principles but in reverse, showcasing the versatility of thermodynamic principles in maintaining desired conditions in various environments.
"The Second Law governs our perceptions of energy flow, shaping how we design and utilize technology to meet modern needs."
In essence, the applications of the Second Law extend far beyond theoretical frameworks. They influence sustainable practices and technological advancements that ultimately lead to better energy management in diverse fields. Understanding thermodynamic cycles and their implications in heat engines and refrigerators equips us with invaluable insights into energy use, conservation, and overall efficiency.
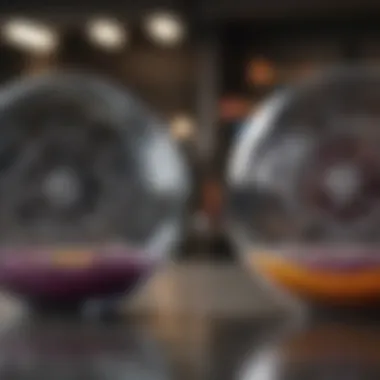
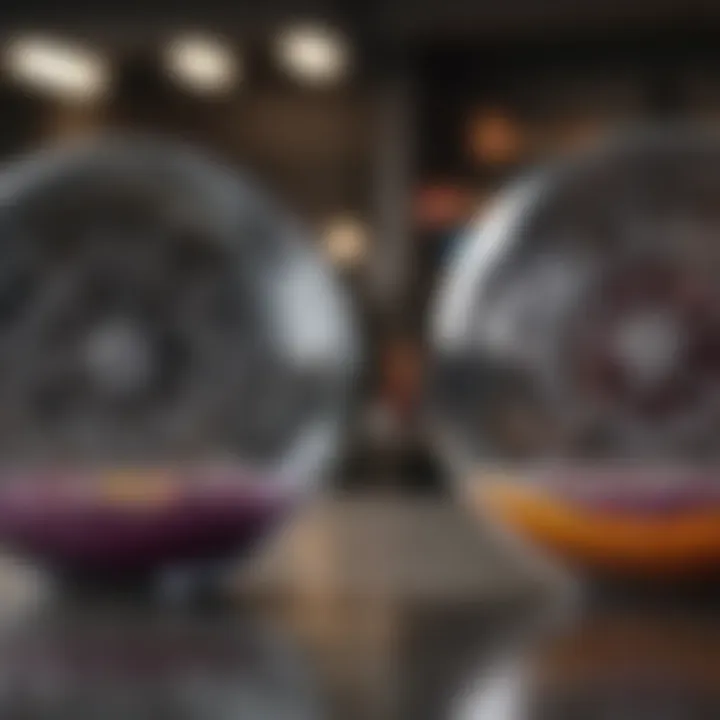
Real-World Examples
Understanding the Second Law of Thermodynamics through real-world examples is not merely an intellectual exercise. It demonstrates its pervasive influence on everyday phenomena and the natural world. This section sheds light on how entropy and energy transformations manifest in practical situations, fostering deeper comprehension of the law's far-reaching implications.
Entropy in Everyday Life
Acting as a touchstone for the Second Law, entropy features prominently in our daily routines. Consider, for instance, a simple cup of hot coffee left unattended on a table. Initially, the liquid is steaming, brimming with heat energy. However, over time, the coffee cools down. The heat gradually disperses into the surrounding environment, signifying an increase in the system's entropy. This gradual loss of usable energy reflects a fundamental principle: systems invariably move towards greater disorder.
Examples abound beyond our kitchens. When you leave your clothes out on a line to dry, they can collect dust and attract insects, leading to a state that’s less orderly than when you first hung them. Every time you mix paint colors, the original pure hues mix into a murky shade. In essence, these everyday occurrences illustrate a core tenet of the Second Law: it’s easier for things to become disordered than to organize themselves back into a neat state.
Biological Implications
The implications of the Second Law extend far into the realm of biology, offering insight into life’s complex processes. Living organisms are not exceptions to thermodynamic rules; rather, they exemplify entropy management through intricate systems. Consider plants as an example. They harness sunlight through photosynthesis, a process that converts sunlight into chemical energy. Here, plants create organized structures from less orderly forms of energy—in this case, light. The plant absorbs sunlight, water, and carbon dioxide, using them to build glucose and oxygen. Yet, while plants create order on a local scale, they ultimately contribute to the universe's overall entropy through respiration and decomposition.
In more complex systems, multicellular organisms navigate vast arrays of chemical reactions, maintaining homeostasis. This balance is fundamental to life but requires continuous energy inputs, underscoring the cyclical nature of energy and entropy where higher organization comes at the cost of increased disorder elsewhere. When organisms die, their biological integrity breaks down, eventually leading to total entropy—a clear demonstration of the Second Law in action.
"In the complexities of life, the constant interaction between energy and entropy is not just a philosophical musing, but rather a fundamental reality that shapes every living system on Earth."
Ending
By connecting the Second Law of Thermodynamics to everyday experiences and biological systems, we can appreciate its importance in shaping our environment and lives. Understanding these examples enriches our grasp not just of thermodynamics as a scientific principle but as a lens through which we can better comprehend the world around us.
The Second Law and Time
The intersection of the second law of thermodynamics and the concept of time presents a fascinating landscape in physics. This connection not only highlights how energy transformations proceed in one direction but also digs deeper into the understanding of natural phenomena. One could say it conjoins the physical realm with our everyday experience of time, making it critical in numerous scientific discussions.
Thermodynamic Arrow of Time
In the realm of thermodynamics, the arrow of time is an essential notion that helps in understanding why certain processes occur in a particular order. Essentially, it implies that time has a direction. When systems undergo changes, they tend to evolve from an ordered state to a more disordered one, often quantified by entropy.
Imagine a room filled with initially neatly stacked boxes, then picture the effects of a sudden gust of wind. The boxes scatter across the floor, leading to a state of disorder. Here, the orderly arrangement transforms into chaos as time progresses. This analogy illustrates the thermodynamic arrow of time, signifying that the process is irreversible.
- Key Concept: The second law states that the total entropy of an isolated system can never decrease over time. If this were to change, it would contradict our experience of time flowing only in one direction.
- Applications: This principle is used extensively in various fields, from understanding how hot coffee cools down to the life cycle of stars. It puts a timestamp on events, defining how they culminate through entropy increases.
"Entropy is often perceived as a measure of randomness. However, it encodes the direction and chances of processes over time."
Causal Relationships in Physics
Causality, at its core, refers to the relationship between causes and effects. Within the scientific context, particularly when discussing thermodynamics and time, the second law plays a pivotal role in defining these relationships. Essentially, if one well-defined cause leads to a specific effect under predictable circumstances, then we can discuss these elements coherently amid conversations about energy and time.
- Energetic Interactions: In any thermodynamic system, every action tends to produce an effect that is measurable through energy changes. For instance, heating a substance typically results in increased molecular motion, which then affects its state.
- Chains of Events: In complex systems, the second law suggests that the entropy or disorder will increase along with time. This interconnectedness serves to illustrate that outcomes in physical systems are not merely arbitrary but predisposed to act under the guidance of the second law, promoting understanding of why events unfold as they do.
Ultimately, the relationship between entropy, energy transformations, and the arrow of time underpins not just physical processes but our broader understanding of the universe. The principles encapsulated in the second law challenge our perceptions and deepen the exploration of causal relationships in the tapestry of reality.
Philosophical Considerations
The exploration of the Second Law of Thermodynamics delves beyond the confines of physics and engineering, reaching into the realms of philosophy, especially when pondering concepts like entropy and its implications. This particular discourse allows us to engage with profound questions regarding the nature of reality, time, and the universe itself. The philosophical implications highlight the interplay between physical laws and the broader human experience, illustrating how scientific principles can shape our understanding of existence.
Entropy and Information Theory
Entropy, as articulated by the Second Law, signifies not just a measure of disorder within a system, but intriguingly, it intersects with the field of information theory. The connection between entropy and information challenges us to reconsider how we view knowledge and uncertainty. In classical thermodynamics, higher entropy corresponds to a more disordered state; conversely, within information theory, entropy quantifies the amount of uncertainty present in a dataset.
- In essence, the more randomized the information, the higher the entropy.
- This asserts a direct relationship between physical states and the information we can derive from them.
This perspective leads to thought-provoking inquiries about whether information itself can ever be lost. Some argue that while physical systems may evolve toward greater entropy, the information that describes the system’s previous state cannot simply evaporate; it exists in some form, reshaping our understanding of reality. It reminds us of the butterfly effect, where small changes can have significant ramifications, asserting that information is persistent even amid chaos.
The Second Law in Cosmology
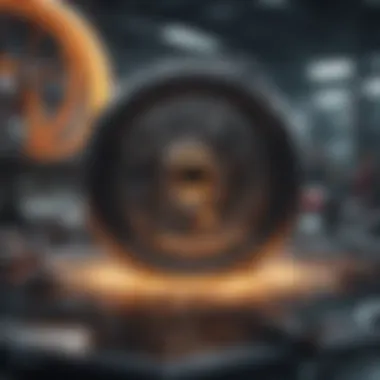
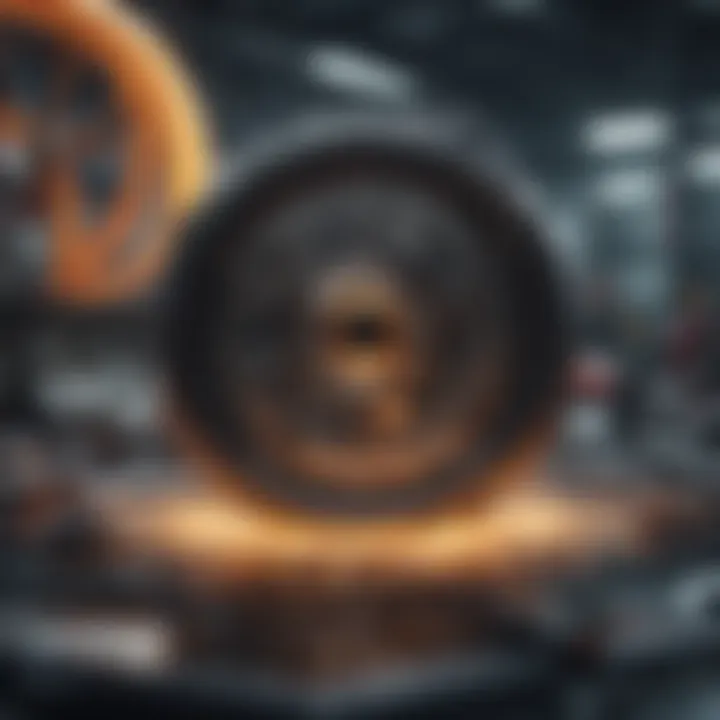
When we shift our focus to cosmology, the Second Law of Thermodynamics illustrates a framework through which we can view the evolution of the universe. The law implies that as the universe expands, it moves toward a state of higher entropy. This leads to two essential considerations:
- The Fate of the Universe: The notion of a heat death emerges—a scenario where the universe reaches a state of maximum entropy, rendering it inhospitable for any processes we recognize as life or structured phenomena. This raises philosophical questions about the meaning of existence in such a distant future.
- Causality and Time: The direction of entropy provides us with an arrow of time. As we observe sequences in which events unfold, this temporal directionality is intrinsically linked to the increase in entropy. The perception of past and future, intertwined with physical laws, guides our philosophical musings about existence, causality, and what it means to experience time.
In sum, the philosophical dimensions tied to the Second Law of Thermodynamics enrich our understanding of not just scientific principles but also the very fabric of reality we inhabit. They encourage an introspection regarding knowledge, existence, and the universe's ultimate fate while stimulating meaningful conversations grounded in both science and philosophy.
Debates and Controversies
The Second Law of Thermodynamics stands as a pillar in the realm of physics, yet it is not without its share of debates and disputes. These discussions often stem from the complexities and nuances of the law as it intersects with various fields, leading to differing interpretations and applications. Understanding these controversies enriches our perspective on the law itself and pushes the boundaries of scientific inquiry. This section delves into the significant arguments surrounding the Second Law, revealing its intricate tapestry through challenges to its basic tenets and the intriguing relationship between entropy and life.
Challenges to the Second Law
The premise of the Second Law revolves around the idea that in any energy transfer or transformation, some energy becomes less useful, a phenomenon widely associated with entropy increasing over time. However, there are notable challenges raised against this law, predominantly focusing on its absolute nature. Critiques often arise in discussions concerning the behavior of systems at microscopic scales, especially in quantum mechanics. Some researchers argue that energy transformations at this level do not always comply with the conventional interpretations of thermodynamic laws, leading to microscopic reversibility where entropy may not exclusively increase.
Moreover, consider the debates stemming from theories like those surrounding Maxwell's Demon. This thought experiment posits a hypothetical creature that could seemingly allow for the reduction of entropy in a closed system, thereby challenging the notion that entropy must always increase. Herein lies the question: does this observation imply that our understanding of thermodynamics may require a reevaluation? While mainstream science largely refutes these anomalies as theoretical curiosities rather than practical realities, they serve as crucial inspiration for theoretical discourse.
"The discussion around the Second Law is not merely academic; it pushes us to expand our comprehension of nature itself."
Negative Entropy and Life
Another layer to the ongoing conversation is the notion of negative entropy, which often crops up in biological contexts. Organisms seem to exhibit the ability to maintain order, creating structures and processes that contradict the overall entropy increase observed in isolated systems. This phenomenon emerges prominently in the study of life, where organisms take in energy and material to grow, reproduce, and maintain homeostasis. The concept of negative entropy suggests that living systems can extract order from their environment, seemingly fighting against the trend dictated by the Second Law.
The way life thrives under the influence of thermodynamic principles raises philosophical and scientific dilemmas. Do we consider living systems as exceptions to the Second Law, or do they embody it under a different light? Understanding this relationship requires a nuanced approach, blending insight from biology, thermodynamics, and even chemistry. This line of inquiry might not only shift the focus of the Second Law but also challenge how we define life and its processes.
In summary, the debates surrounding the Second Law of Thermodynamics encapsulate a dynamic intersection of ideas, challenging our long-standing beliefs and inviting innovative perspectives. As science progresses, so too must our interpretations, ensuring that we keep pace with the complexities of the universe.
Future Perspectives
As we peer into the future of thermodynamic studies, particularly through the lens of the Second Law, it’s paramount to consider how advancements in technology and theoretical physics will shape our understanding of this foundational principle. The exploration of emerging research directions offers a glimpse into the intricate web of discoveries awaiting us in different scientific domains. Furthermore, understanding the implications and nuances of how the Second Law operates at the quantum level opens up paths for groundbreaking breakthroughs and applications across various fields.
Emerging Research Directions
Currently, researchers are diving deep into several emerging directions that could redefine our comprehension of thermodynamics. Here are a few noteworthy areas:
- Nanotechnology: The manipulation of materials on a molecular scale is increasingly relevant. Researchers are investigating how entropy behaves differently in nanoscale systems compared to macroscopic ones. The behavior of entropy at tiny scales could reveal new phenomena that challenge existing thermodynamic principles.
- Biological Systems: There’s a growing interest in how living organisms maintain order and transfer energy, seemingly violating the Second Law. Investigating these systems provides insights into negative entropy and how life sustains itself in complex environments.
- Sustainable Energy Technologies: With the relentless push for cleaner energy sources, scientists are exploring how to optimize thermodynamic cycles for renewable energy systems. Understanding the Second Law could lead to inventions that maximize efficiency in solar panels or wind turbines, minimizing waste in the process.
- Information Theory: There's burgeoning interaction between thermodynamics and information theory, particularly concepts like 'Maxwell's Demon,' which challenges our interpretations of information and entropy. Researchers are perplexing over how information processing affects energy states, raising philosophical questions and enhancing the interplay between these fields.
This exploration of emerging directions emphasizes humanity's unyielding quest for knowledge and innovation, reinforcing the idea that while the Second Law sets boundaries, it also fosters creativity and ingenuity in humanity’s understanding of the universe.
The Second Law in Quantum Mechanics
In the realm of quantum mechanics, the Second Law of Thermodynamics takes on a nuanced role that continues to stir debates and intrigue. Here, a few significant points come into play:
- Quantum Entanglement: In classical thermodynamics, entropy tends to increase in an isolated system, suggesting a one-way street towards disorder. However, in quantum mechanics, entangled particles defy classical notions of independence, leading to questions about how their entangled state contributes to the overall entropy of a system. This has immense implications for our understanding of information transfer and energy states.
- Thermodynamic Behavior of Quantum Systems: Researchers are tasked with understanding how thermodynamic laws function on the quantum scale, where particles exhibit probabilistic behavior. Studies show that quantum systems can achieve states of low entropy that classical systems cannot, providing fertile ground for future work.
- Heat Engines on a Quantum Scale: The concepts of the Second Law are being tested in the design and optimization of quantum heat engines. This represents not only technological advancement but also a prime example of how quantum mechanics reshapes our views about thermodynamics.
Through these explorations, we pose deeper questions about the relevance of thermodynamic laws in realms once thought to be exclusively classical. Are these laws flexible? Could they adapt as we decode nature's intricacies at a more microscopic level?
As we continue to explore these dimensions, one thing remains glaringly clear: the Second Law of Thermodynamics isn’t just a relic of classical physics; it’s a living principle entwined with frontier research.
The journey of scientific inquiry often leads us back to the foundation, urging us to reconcile classical understanding with quantum realities.
The End
In wrapping up our exploration of the Second Law of Thermodynamics, it's vital to underscore several critical points that have emerged throughout the discourse. This law, with its implications stretched across various scientific realms, serves as a bedrock for our understanding of energy interactions and transformations. Moreover, it introduces the concept of entropy—a measure of disorder that governs not only physical systems but hints at deeper philosophical and cosmological considerations as well.
Summarizing Key Takeaways
- Entropy as a Measure of Disorder: The key takeaway from this article is the role of entropy in the Second Law, emphasizing that in any energy transfer or transformation, disorder tends to increase over time. This fundamental insight lays the groundwork for understanding processes in both closed and open systems across numerous fields.
- Relevance in Real-World Applications: It’s also noteworthy how the Second Law governs not just theoretical concepts, but tangible applications. From designing efficient heat engines and refrigerators to understanding biological systems, the implications of this law are omnipresent.
- Philosophical and Temporal Insights: Beyond science, the discussions on entropy lead to intriguing philosophical questions regarding the nature of time and our universe. The notion of a temporal arrow defined by entropy change offers a unique perspective on the universe's evolution.
- Future Research Directions: Emerging research points toward the intersection of the Second Law with quantum mechanics, challenging existing paradigms and potentially reshaping our understanding of thermal systems at their most fundamental levels.
The Ongoing Relevance of the Second Law
The Second Law of Thermodynamics is more than a set of theoretical principles; it is a living framework that continues to evolve as humanity delves deeper into the laws of nature. Its principles remain fundamental in areas like physics, chemistry, and engineering, prompting both academic and industrial advancements.
- In environmental science, understanding energy transfer and entropy can inform sustainable practices and renewable energy solutions.
- The biological implications of the Second Law provide insights into life processes, emphasizing resilience and adaptation in the face of the inevitable drift toward higher entropy.
- In cosmology, the Second Law serves as a crucial element in theories regarding the fate of the universe, influencing our comprehension of cosmic evolution.
Thus, as we look to the future, the Second Law of Thermodynamics will undoubtedly continue to inspire curiosity, debate, and innovation across disciplines. Its persistent relevance reflects not just the simplicity of its fundamentals but the profound complexities it encompasses.