Distinguishing Quantum Physics from Quantum Mechanics
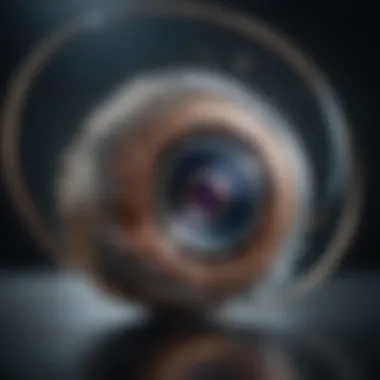
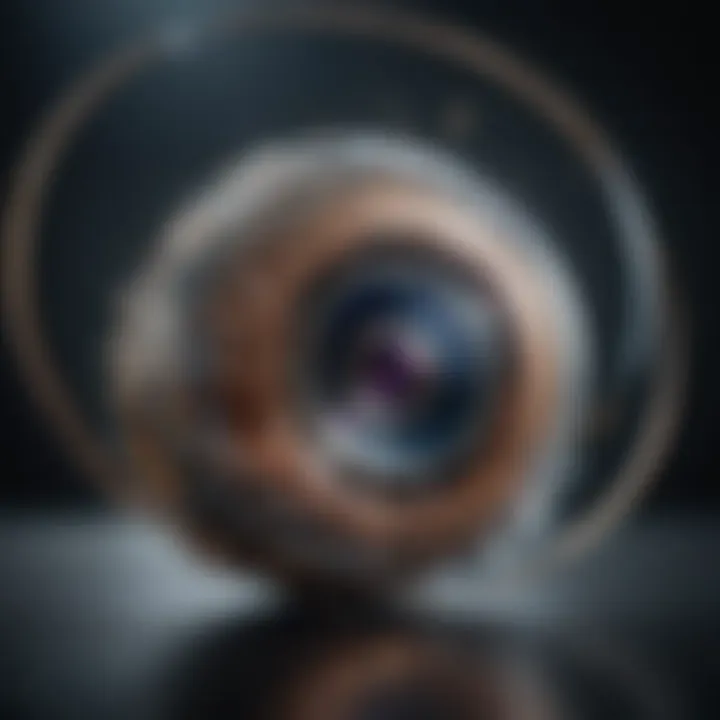
Intro
In the realm of modern physics, the terms quantum physics and quantum mechanics often float around like autumn leaves in a brisk wind. They seem similar but carry distinct meanings that hold sway over our understanding of the universe. At its core, quantum physics serves as the umbrella term encapsulating a broad field of studies, while quantum mechanics zooms in on the mathematical and theoretical frameworks that govern the microcosm of particles. This distinction is not just academic; it carries real implications in various scientific domains.
To break it down further, think of quantum physics as the entire orchestra, while quantum mechanics is a specific musician playing a unique instrument. Each plays a vital role in creating the symphony of understanding about how the universe behaves at its smallest scales.
As students, educators, and science enthusiasts venture deeper into this fascinating world, it becomes crucial to dissect these concepts for clarity. In the sections that follow, we will delve into recent discoveries, key principles, and the significance of these findings, paving a clear path for understanding how quantum physics and quantum mechanics intertwine yet diverge.
Intro to Quantum Physics and Quantum Mechanics
The terms quantum physics and quantum mechanics often dance around each other in the realm of theoretical physics. However, understanding the distinction between them is not just academic rigor; it’s crucial for grasping how they shape modern science. This section will illuminate the framework of these two fields, giving readers insight not only into their definitions, but also the implications and significant concepts that underpin them.
Defining Quantum Physics
Quantum physics refers to the overarching body of interdisciplinary science that examines the behavior of matter and energy on the smallest scales, particularly at the level of atoms and subatomic particles. It's a broad field that encompasses various principles and theories, tackling the perplexing nature of the universe. At its core, quantum physics is concerned with phenomena that classical physics can't explain—like the strange behavior of particles that can be both here and there at the same time. Think of it as the umbrella that covers the intricate patters of how particles interact.
Key aspects that define quantum physics include:
- Fundamentals of light and matter: The duality where particles can act as waves and vice versa.
- Quantization: The realization that energy levels in an atom are quantized rather than continuous, leading to various implications in electron configurations.
- Complexity of interactions: The interactions between particles often produce unexpected outcomes, challenging our classical intuitions.
These ideas are not merely theoretical; they have practical ramifications, manifesting in technologies such as lasers, semiconductors, and even the computers we use daily.
Understanding Quantum Mechanics
When we talk about quantum mechanics, we’re diving deeper into the mathematical frameworks that describe and predict the behaviors of particles at a quantum level. Quantum mechanics is a subset of quantum physics, focusing on the mathematical models and theories—or the 'how'—that drive the phenomena observed. Essentially, it provides the tools we need to unpack the intricacies of quantum systems.
Some notable components of quantum mechanics include:
- Wave functions: They describe the quantum state of a system, allowing us to predict the probabilities of a particle’s properties.
- Operators and observables: Through mathematical operations, we can extract measurable quantities from wave functions, linking theory to experiment.
- Postulates of quantum theory: These foundational rules give insight into how physical systems evolve over time and the role of measurement.
In essence, while quantum physics lays the groundwork, quantum mechanics provides the lexicon that allows physicists to articulate these complex concepts.
"Quantum mechanics offers a framework to interpret phenomena that seem utterly bizarre, yet within that complexity lies the beauty of the universe's fabric."
Together, both fields interweave a tapestry that defines modern physics, serving scholars and students a clearer canvas to paint upon as they delve into the wondrous curiosities of the microscopic world.
Historical Context of Quantum Theories
Understanding the historical context of quantum theories is essential for grasping how modern physics has evolved. The enduring journey through physics and its principles has been marked by significant milestones and paradigm shifts. The early days, rife with experimentation and discovery, laid the framework upon which contemporary quantum mechanics stands. Recognizing these developmental phases helps elucidate the complexities and interrelations between quantum physics and quantum mechanics.
Early Developments in Quantum Science
The groundwork for quantum science was laid in the late 19th and early 20th centuries when classical physics started to face challenges it couldn’t explain. One key figure in this era was Max Planck, whose work on black-body radiation led him to propose that energy could only be emitted or absorbed in discrete units, which he called "quanta." This idea, although initially revolutionary, was the seed for what would later grow into a fully-fledged quantum theory.
Another pivotal moment in the early development was Albert Einstein's 1905 paper on the photoelectric effect. Here, Einstein posited that light itself could be viewed as discrete packets of energy, which we now refer to as photons. His insights reinforced the notion that particles could exhibit both wave-like and particle-like properties, thus sowing the seeds of what we now acknowledge as wave-particle duality.
Throughout these formative years, experimental evidence was piling up, challenging long-held perceptions of a continuous physical world. As scientists such as Niels Bohr entered the scene, they began to construct models that could explain atomic structure and behavior. These evolving ideas paved the way for more sophisticated theories, revealing that the very nature of reality could be profoundly different from what was commonly accepted.
Formation of Quantum Mechanics
As the 20th century progressed, the academic landscape shifted dramatically, resulting in the formal establishment of quantum mechanics as a distinct discipline. Several minds contributed to this monumental task. Heisenberg's uncertainty principle, introduced in 1927, illustrated the limitations of measuring certain pairs of physical properties simultaneously, such as position and momentum. This principle raised fundamental questions about the nature of reality and observation, suggesting an inherently probabilistic framework.
Moreover, Schrödinger's wave equation offered a mathematical representation that described how quantum states evolve over time. It was revolutionary in establishing a relationship between observable quantities and their wave-like behavior. The collaboration of these theories, along with contributions from others such as Dirac and Feynman, solidified the notion of quantum mechanics as a robust and essential field of study.
The successful integration of these ideas not only transformed physics but also set the stage for the development of numerous applications, from semiconductors to lasers. It also delved into philosophical territories, provoking debates about determinism versus free will, the nature of reality, and the implications of human consciousness on observation.
"The universe is not only stranger than we imagine; it is stranger than we can imagine."
— Sir Arthur Eddington
In summary, the historical context of quantum theories is not merely a backdrop; it is a crucial narrative that illuminates the tides of thought and innovation that have shaped our understanding of the universe. Studying these developments helps bridge the often perceived gap between quantum physics and quantum mechanics, offering insights into how they inform and interact with one another.
Key Principles of Quantum Physics
Understanding the key principles of quantum physics is like unearthing the foundations upon which the entire field is built. These principles serve as the bedrock for both theoretical and practical applications, influencing technologies like lasers, semiconductors, and even quantum computers. Knowing these concepts also aids in demystifying the otherwise daunting nature of quantum mechanics which opts to fine-tune the details beyond the broad strokes of quantum physics. Each principle peels back a layer of reality, revealing a world that operates on rules vastly different from classical physics.
Wave-Particle Duality
The principle of wave-particle duality challenges the very notion of how we understand particles and waves. Take, for instance, the famous double-slit experiment: shining light through two closely spaced slits creates an interference pattern, characteristic of waves. However, if you observe the particles traveling through the slits, they behave like distinct particles. This means that, depending on whether we are observing or not, light can act as both a wave and a particle.
This dual nature isn't limited to light alone; matter exhibits similar behavior. Electrons, often viewed as particles, also display wave-like characteristics. To illustrate, consider a situation where the same electron behaves like a wave spreading out across space, only to 'collapse' into a specific location upon measurement. The implications of this principle stretch across various scientific domains, prompting us to rethink everything from the behavior of tiny particles to the very nature of reality itself.
Quantum Superposition
Quantum superposition is another cornerstone of quantum physics, describing how particles can exist in multiple states at once. Imagine, if you will, flipping a coin; it lands either heads or tails. In the quantum world, the coin can be both heads and tails at the same time until someone takes a look. This concept is embodied by Schrödinger's cat, a thought experiment where the cat in the box is simultaneously alive and dead until observed.
This principle is crucial for understanding how quantum computing operates. A quantum bit, or qubit, takes advantage of superposition to perform multiple calculations simultaneously. This feature equips quantum computers with the potential to solve complex problems significantly faster than classical computers. Hence, superposition extends beyond mere theory; it has profound implications for advancing technology.
Quantization of Energy
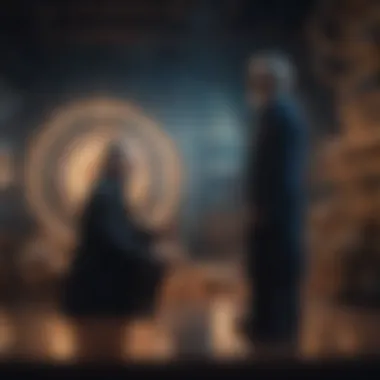
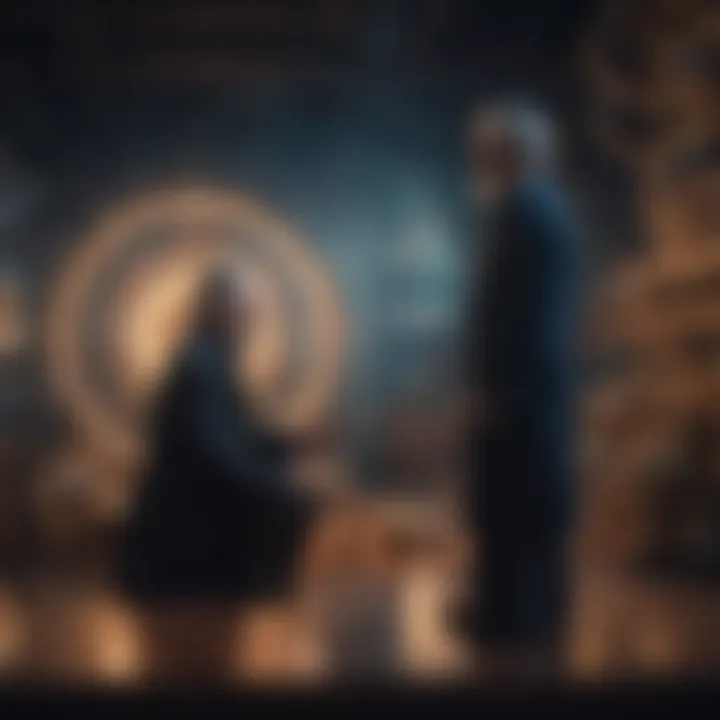
Lastly, the quantization of energy brings a significant twist to our understanding of energy levels within atomic structures. In classical terms, energy can vary smoothly—any value is possible. However, in the quantum realm, energy exists in discrete packets known as quanta. For example, electrons orbiting atomic nuclei cannot occupy just any position; they reside in specific energy levels or shells.
This principle has vast implications, particularly in fields like chemistry where the emission or absorption of light by atoms is based on transitions between these energy levels. The predictability of chemical reactions and the behavior of matter under different conditions stem largely from these quantized energy states, allowing us to grasp why certain reactions occur while others remain dormant.
Understanding these principles cultivates a clearer perspective on the quantum world, signaling a shift from classical to radical modern physics.
Fundamental Aspects of Quantum Mechanics
Understanding quantum mechanics is crucial for grasping the intricate behaviors of particles at the subatomic level. This section navigates through some of the central concepts in quantum mechanics, which lay the groundwork for modern applications and theoretical advancements in the field. The three significant aspects—The Uncertainty Principle, Quantum Entanglement, and Quantum Tunneling—play pivotal roles in not just the physics lab but also in our day-to-day technological advancements. It's like trying to make sense of puzzles that hold the key to the universe's most profound secrets.
The Uncertainty Principle
The Uncertainty Principle, introduced by Werner Heisenberg in 1927, fundamentally changes how we perceive measurement in quantum mechanics. Simply put, it states that you cannot precisely know both the position and momentum of a particle at the same time. The more accurately you know one, the less accurately you can know the other. This is not just a limitation of measurement tools, but a fundamental property of nature.
"In quantum mechanics, the more you know about the velocity of a particle, the less you know about its position."
This brings forth several enlightening considerations:
- Observation Impact: Measuring a particle alters its state, increasing the uncertainty of the complementary property.
- Quantum Realm: The principle applies at very small scales, suggesting a realm where traditional physics fails to explain behaviors.
- Philosophical Depth: It stirs philosophical debates about determinism and reality, challenging our preconceived notions about the predictability of nature.
Overall, the Uncertainty Principle reshapes our understanding of reality and has significant implications in quantum theory and applications like quantum computing.
Quantum Entanglement
Quantum entanglement is another distinctive feature of quantum mechanics, where particles become intertwined such that the state of one particle instantaneously influences the state of another, regardless of the distance separating them. It’s like having two magic coins—flip one, and the other shows heads or tails simultaneously, even if it's miles away. Albert Einstein famously referred to it as "spooky action at a distance."
The implications are vast:
- Information Transfer: This phenomenon may revolutionize communication systems by allowing instantaneous transfer of information.
- Quantum Teleportation: Quantum entanglement proves essential in processes like quantum teleportation, where a quantum state is transmitted from one location to another without moving the physical particle itself.
- Challenging Classical Physics: It raises questions about locality and causality, which are foundational concepts in classical physics.
Quantum Tunneling
Quantum tunneling is a phenomenon where particles pass through potential barriers that, according to classical physics, they should not be able to surmount. Imagine a ball trying to roll over a hill but instead simply appearing on the other side without going over the top. This may sound far-fetched, but it's a cornerstone of quantum behavior.
Its significance cannot be overstated:
- Nuclear Fusion: This process is crucial for the fusion reactions that power stars, including our sun. Without quantum tunneling, the sun wouldn't shine as it does.
- Transistors: In electronics, tunneling affects how semiconductors work, shaping the devices we rely on.
- Chemical Reactions: Tunneling can also play a role in how molecules interact and react in unpredictable ways.
Distinctions Between Quantum Physics and Quantum Mechanics
Understanding the differences between quantum physics and quantum mechanics is crucial in grasping how each field contributes to modern science. While both terms often get thrown around interchangeably, they focus on unique aspects of the quantum world. Essentially, quantum physics is the broader umbrella under which quantum mechanics sits, serving as a specific application of the principles laid out by quantum physics.
The key elements to consider in these distinctions are numerous. First and foremost is the scope of both fields. Quantum physics encompasses a range of topics, including the behaviors and interactions of matter and energy at the quantum level. In contrast, quantum mechanics hones in on the mathematical formulations and principles that explain these behaviors. Understanding these differences allows for a clearer picture of how theories evolve and intertwine, beneficial for students and scholars alike.
Scope and Scale
The scope and scale of quantum physics and quantum mechanics differ significantly. Quantum physics covers a wide array of phenomena, from the properties of photons to the structure of atoms. It sets the foundation for various branches of physics that tackle topics ranging from thermodynamics to electromagnetism, shedding light on how quantum behavior influences macroscopic systems.
In stark contrast, quantum mechanics focuses more narrowly on a precise mathematical framework that describes how particles behave. This discipline provides the tools necessary to predict outcomes in experiments. For instance, while quantum physics might discuss the photoelectric effect as a way to introduce light's particle-like nature, quantum mechanics would present the mathematical equations that describe the release of electrons when light hits a metal surface. The scope helps in elucidating general phenomena, while the mechanics delve into the nuts and bolts of physical predictions.
Mathematical Frameworks
When diving into the world of quantum mechanics, the mathematics involved becomes apparent. The use of equations is not just incidental but serves as the backbone of understanding quantum behavior. Key mathematical tools in quantum mechanics include wave functions, operators, and the Schrödinger equation. These mathematical constructs are essential for analyzing how particles behave under different conditions and for predicting the probabilities of various outcomes.
In contrast, quantum physics often presents concepts qualitatively, though it does include mathematical implications as one progresses into deeper studies. Here, some might find a steep learning curve in transitioning from the broader principles of quantum physics to the exacting demands of quantum mechanics. This fundamental difference in approach underscores the necessity of a solid mathematical foundation when studying quantum mechanics.
Philosophical Implications
Diving deeper into the intellectual realm, the philosophical implications of quantum physics and quantum mechanics give rise to fascinating discussions. Quantum physics encourages broader contemplation about the nature of reality, existence, and how we perceive the universe. This exploration leads to philosophical inquiries about determinism and the nature of particles and waves. Concepts such as wave-function collapse spark debates on whether the universe is fundamentally probabilistic or deterministic.
On the other hand, quantum mechanics, with its precise calculations and predictions, often cements a more pragmatic view of these philosophical questions. The implication is that understanding the complexities of our universe comes down to employing the right mathematical models. A better grasp of these philosophical differences can enrich the conversation amongst students and professionals, urging them to think critically about these profound implications.
"To understand [quantum physics and mechanics] is to grasp the essence of reality from the underlying principles to observable phenomena."
In summary, distinguishing between quantum physics and quantum mechanics reveals a rich fabric of interconnected ideas, principles, and implications. It allows enthusiasts and academics to engage deeply with the subject matter, setting the stage for further exploration into advanced topics. Scientists, students, and philosophers can unite in their quest for unveiling the mysteries shaped by quantum phenomena by appreciating these subtleties.
Applications of Quantum Physics
Quantum physics stretches its arms wide into various fields, influencing both the theoretical and practical realms of science and technology. These applications aren't just pie-in-the-sky ideas; they are concrete innovations that are reshaping industries and enhancing our daily lives. Let’s dive into a couple of its most prominent uses:
Quantum Computing
Quantum computing is like a magic box that can solve problems too complex for classical computers. At its core, it leverages principles from quantum mechanics, such as superposition and entanglement, to process information in a fundamentally different way. Unlike traditional bits that hold a value of either 0 or 1, quantum bits, or qubits, can represent both values simultaneously. This unique property provides immense power for parallel processing.
- Speed: Quantum computers have the potential to execute complex algorithms at speeds unimaginable with classical counterparts. Tasks like factoring large numbers or optimizing complex systems could take seconds rather than years.
- Complex Problem Solving: Industries such as logistics, pharmaceuticals, and finance are exploring how quantum computing can enhance operational efficiencies and accelerate research and development processes.
- Encryption and Security: This technological leap holds promise for creating unbreakable encryption methods, which will secure sensitive data far beyond current systems.
"Quantum computers can exist in multiple states at once, giving them a quantitative edge that feels almost sci-fi but is very much in the realm of the possible today."
Quantum Cryptography
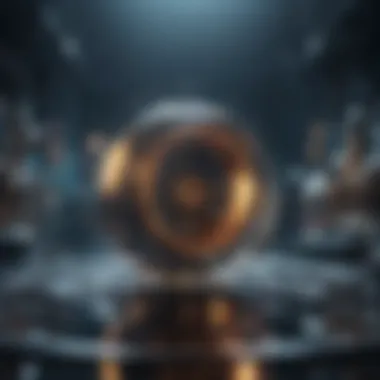
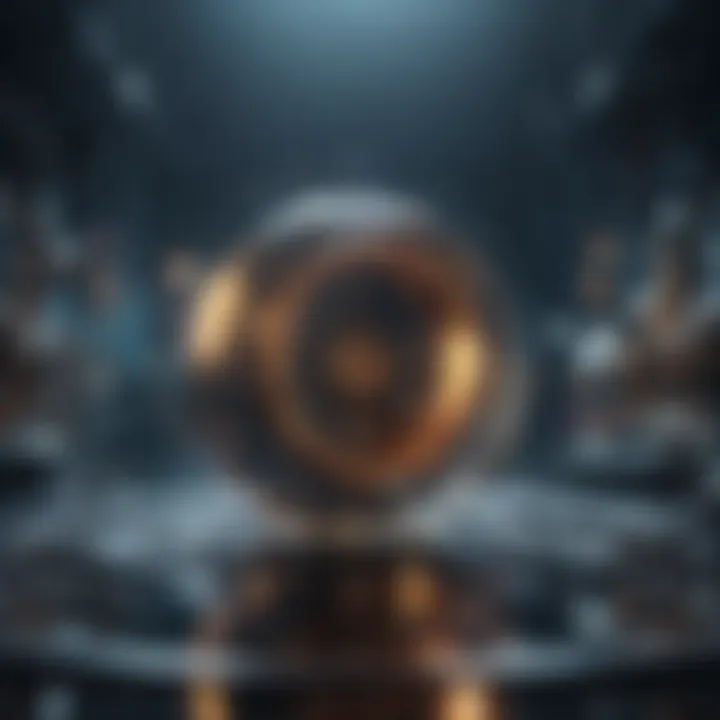
Imagine having a security system so robust that it can't be hacked—this is the pathway quantum cryptography is paving. Utilizing the properties of quantum mechanics, this method ensures secure communication by the principles of quantum key distribution (QKD).
- Unbreakable Security: The beauty of quantum cryptography lies in its principle of uncertainty. Any attempt to eavesdrop on the quantum key will disturb the quantum states, alerting the parties of a breach.
- Real-world Applications: Organizations are already implementing QKD in finance and healthcare sectors to safeguard sensitive information. Some countries are even testing national quantum communication networks.
- Future Potential: As quantum technology evolves, the integration of quantum cryptography with existing systems could redefine the cybersecurity landscape, making sensitive transactions impenetrable.
By focusing on these unique applications, quantum physics not only enriches our understanding of complex theories but also translates those theories into real-world innovations that can tackle current challenges and expand the horizons of what's possible in technology and security.
Real-world Implications of Quantum Mechanics
Quantum mechanics isn't just a concept confined to academic halls or scientific journals. Its influence permeates various aspects of our daily lives and technological advancements. Understanding the real-world implications of quantum mechanics is crucial for grasping how it shapes the world around us.
Impact on Technology
The effects of quantum mechanics on technology can be likened to ripples in a pond. Once the stone of quantum theory was thrown, the waves began spreading into various industries.
One of the most significant breakthroughs is in quantum computing. Traditional computers, based on binary systems, process data in bits. Quantum computers, however, utilize qubits which allow them to perform complex calculations much faster and more efficiently.
- Speed and Efficiency: Quantum computers can tackle problems that would take classical computers years to solve in mere seconds.
- Applications in Cryptography: Modern encryption methods can be transcended through quantum algorithms, opening avenues for secure communication that are virtually unbreakable.
Furthermore, quantum sensors are reshaping fields such as medical imaging and navigation technologies. These devices operate on principles that drastically improve sensitivity and accuracy. For instance, quantum sensors can aid in detecting gravitational waves, thus expanding our understanding of the universe.
Influences on Philosophy of Science
Beyond technology, quantum mechanics also leaves its mark on the philosophy of science. It challenges longstanding notions of determinism and reality itself, sparking debates that have profound implications for our understanding of existence.
For instance, the idea of superposition—where particles exist in multiple states simultaneously—raises questions about the nature of reality. If we delve deeper, we see that this leads to various interpretations:
- Copenhagen Interpretation: Suggests reality exists in a probabilistic state until observed.
- Many-Worlds Interpretation: Proposes that every possibility actually occurs, leading to a multitude of parallel universes.
These philosophical discussions help frame how we understand not only quantum mechanics but also our own existence and the essence of scientific inquiry. As scientists push the boundaries of what we know, the implications tread into realms that intertwine science with human philosophy.
"The more I study quantum mechanics, the more I’m convinced that we’re not merely looking at particles but peering into the very fabric of reality that connects us all."
— A Thoughtful Refleciton by a Quantum Physicist
To sum up, the implications of quantum mechanics extend far beyond the laboratory. They take root in the technological frameworks that form the backbone of modern society and bridge into philosophical realms that question our understanding of reality. Such insights resonate with students, educators, and professionals alike, reinforcing the notion that quantum mechanics is undeniably pivotal in navigating the complexities of our universe.
Noteworthy Figures in Quantum Physics and Mechanics
In the landscape of quantum science, certain individuals stand out, not just for their groundbreaking discoveries, but also for how their thoughts shaped the very fabric of modern physics. This section delves into the lifework of key figures whose contributions continue to resonate throughout both quantum physics and quantum mechanics. Understanding their roles provides valuable context for the theories and principles discussed earlier in this article; it sheds light on the evolution of ideas and assumptions that underpin contemporary scientific discourse.
Max Planck
Max Planck, often regarded as the father of quantum theory, played a pivotal part in changing our understanding of energy and radiation. His introduction of the concept of quantization in 1900 marked a fundamental departure from classical physics, challenging previously held beliefs about energy continuity. Planck proposed that energy is emitted or absorbed in discrete units, called quanta. This idea was groundbreaking and laid the foundation for the entire framework of quantum mechanics.
Planck’s constant, denoted by the symbol h, became one of the most important constants in physics, influencing countless theories and applications, from lasers to semiconductors.
"Science cannot solve the ultimate mystery of nature, and that is because in the last analysis we ourselves are part of the mystery we are trying to solve."
— Max Planck
His work was essential in later developments by physicists like Einstein and Bohr, painting a broader picture of what energy entails in the quantum realm. Without his insights, it's arguable that subsequent innovations in quantum mechanics would not have unfolded as they did.
Niels Bohr
Niels Bohr is another towering figure whose ideas contributed fundamentally to our understanding of atomic structure and quantum behavior. His model of the atom proposed that electrons occupy specific orbits around the nucleus, and these orbits correspond to defined energy levels. Bohr's work on quantized energy levels provided a method to explain the emission and absorption spectra of elements, thereby bridging the gap between theoretical physics and observable phenomena.
Moreover, Bohr introduced the principle of complementarity, which asserts that objects may have dual wave-particle features yet cannot exhibit both qualities at the same time in a single experimental setup. This principle underscored the inherent complexities and paradoxes in quantum mechanics, pushing the boundaries of philosophical inquiry into the nature of reality.
Albert Einstein
While often associated with relativity, Albert Einstein made significant contributions to quantum science that should not be overlooked. His 1905 paper on the photoelectric effect was pivotal in demonstrating that light can behave as both a wave and a particle. By introducing the idea that light consists of quanta, or photons, he provided empirical support for Planck’s earlier formulations, opening new avenues in quantum theory.
Einstein famously struggled with the implications of quantum mechanics, particularly its probabilistic nature, famously stating, "God does not play dice with the universe." His disagreements and insights helped to spur critical discussions that advanced the field, highlighting both the power and limitations of quantum theories.
In summary, these notable figures not only shaped the course of quantum physics and mechanics through their groundbreaking theories but also invited future scholars to grapple with complex ideas that still influence modern scientific thought today.
Current Research Trends
In the fast-paced realm of science, current research trends in quantum studies reveal a lively and evolving landscape. These trends are not merely academic pursuits; they hold the key to unlocking profound understanding and real-world applications of quantum physics and mechanics. As researchers dive deeper, they navigate through uncharted territories with the potential for groundbreaking discoveries.
Advancements in Quantum Theory
Advancements in quantum theory illustrate the ongoing quest to unearth deeper truths about the universe. Recently, scientists have made strides in areas such as quantum information theory, leading to innovative applications like quantum computing. Understanding how quantum states can be manipulated has implications that extend well beyond theoretical work; they are shaping future computing technologies. Moreover, researchers are exploring new paradigms such as quantum thermodynamics, which investigates thermodynamic laws at quantum scales.
Furthermore, concepts like quantum gravity are gaining traction. Scholars are finding ways to reconcile quantum mechanics with general relativity, a challenge that has puzzled physicists for decades. These advancements signal not just progress in understanding but potentially transformative shifts in how we perceive time and space itself.
Interdisciplinary Approaches
The field of quantum research is increasingly intermingling with other disciplines, showcasing the rich tapestry of scientific inquiry. Interdisciplinary approaches blend insights from physics, biology, and even philosophy to enhance our understanding of quantum phenomena. For instance, quantum biology investigates the role of quantum effects in biological processes such as photosynthesis and enzyme catalysis. The synergy between these fields offers a more holistic view of nature's mechanisms.
On another front, collaborations between physicists and computer scientists are leading to innovations in data processing and cryptography. The rise of quantum cryptography highlights how quantum principles are being harnessed to create unbreakable codes, a game changer for secure communications.
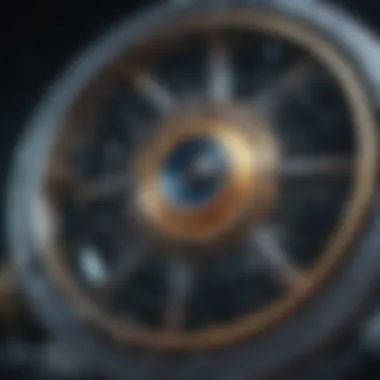
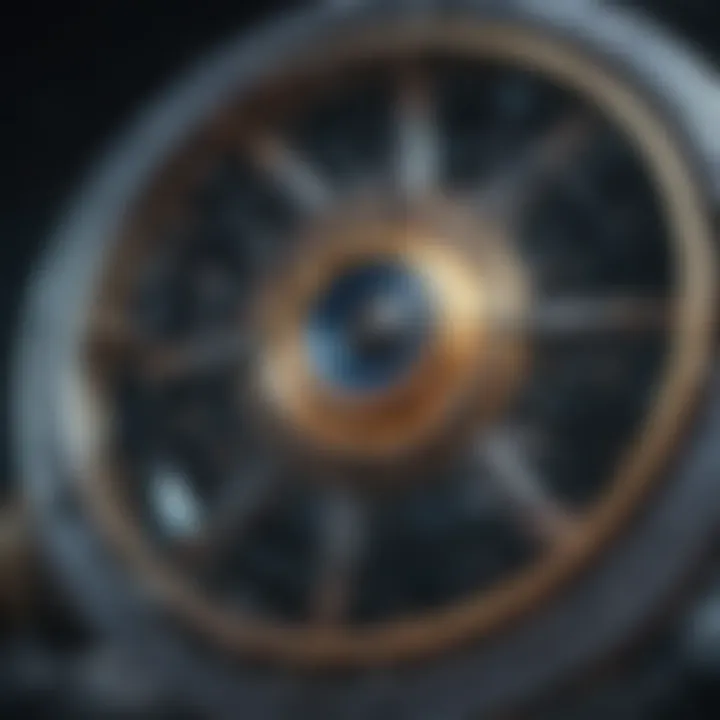
In summary, current research trends engage an expansive range of ideas, reflecting a dynamic interplay between theoretical and applied science. As boundaries dissolve between disciplines, research becomes enriched with diverse perspectives, highlighting the integral role of both quantum physics and mechanics in addressing complex, modern challenges.
"The science of today is the technology of tomorrow."
Understanding these research trends better prepares students, educators, and professionals alike to navigate the intricate landscape of quantum studies and their far-reaching implications for society.
Future Directions in Quantum Studies
The future directions in the realm of quantum studies represent a vibrant tapestry of possibilities, heavily influencing not just theoretical frameworks, but practical applications as well. As we unravel the threads connecting quantum physics and quantum mechanics, it becomes clear that these pathways not only guide research but also pave the way for innovations that could reshape our understanding of the universe.
Potential Breakthroughs
When discussing potential breakthroughs, one can’t help but feel a current of excitement palpable in the air. One area poised for remarkable progress is quantum information science, particularly in quantum computing. Current quantum processors are often limited by the phenomenon of decoherence. However, researchers are exploring novel materials and error correction methods aimed at improving reliability and efficiency. Achieving stable qubits could lead to computational speeds previously thought unattainable, revolutionizing industries from pharmaceuticals to cryptography.
Some added realms of focus include quantum networks, which aspire to establish qubits interlinked over long distances. Such developments may lead to a safer form of communication, where information is transmitted with near absolute security, exploiting the principles of quantum cryptography. The fusion of artificial intelligence with quantum technologies is another exciting frontier, potentially enhancing the ability to process vast amounts of data in ways we cannot yet fully grasp.
- Increased reliability in quantum systems
- Enhanced communication security
- Dramatic acceleration in computational speeds
- Blending AI and quantum theories for data processing
Challenges to Overcome
While the potential is abundant, the road ahead is not without bumps. Among the most pressing challenges is the issue of scalability. Current quantum systems, while impressive in small-scale experiments, are not yet suitable for widespread practical use. Engineers are grappling with the practical intricacies of creating systems that can operate efficiently at larger scales, overcoming not just technical boundaries but economic ones as well.
Furthermore, there’s a growing need for a comprehensive education in quantum theory. The interdisciplinary nature of this field often leads to jargon-heavy discussions that can alienate newcomers. Fostering a broader understanding among enthusiasts and professionals alike will be key to nurturing future innovations. Improving funding and support for collaborative projects will also facilitate significant advancements.
"The challenge is not merely the complexity of quantum theories but also the intricacies involved in communicating these ideas effectively."
In summary, the trajectory of quantum studies is a rich landscape filled with potential breakthroughs and significant challenges. The quest for scalable, reliable systems coupled with a broader and deeper understanding of quantum theories will drive the next wave of innovations, influencing lives and industries in profoundly transformative ways.
Misconceptions About Quantum Physics and Mechanics
Understanding the realms of quantum physics and quantum mechanics can be quite the brain teaser. Many individuals, even those somewhat familiar with the topics, harbor significant misconceptions about what these terms truly signify. This section is pivotal as it seeks to unravel the common misunderstandings and provide clarity. Grasping these nuances is essential for appreciating the vast implications of quantum theories in our modern scientific landscape.
Common Misunderstandings
There are several persistent myths surrounding quantum physics and mechanics that can muddle one's comprehension of these complex fields:
- Particle vs. Wave Duality: A prevalent belief is that particles are either waves or particles. However, they can exhibit both behaviors depending on the conditions of observation. This nuanced behavior reflects the fundamental concept of wave-particle duality.
- Instantaneous Action: Many assert that quantum entanglement implies instantaneous communication between particles over vast distances. This misunderstanding can lead to the misleading notion of faster-than-light communication, which violates established principles of relativity.
- Relation to Classical Physics: Some people think quantum mechanics is the ultimate replacement for classical physics. While classical theories can indeed fall short in certain scenarios, they remain highly effective for macroscopic phenomena, acting almost like a specialized case within the broader quantum framework.
These misunderstandings do not just create confusion but also hinder further exploration of quantum concepts.
Clarifying Terms
To aid better understanding, some crucial terms associated with quantum physics and quantum mechanics should be clarified:
- Quantization: Refers to the idea that certain properties, such as energy, can only occur in discrete levels. It’s a fundamental tenet in quantum mechanics that sets it apart from classical theories where properties are treated as continuous.
- Superposition: This term describes a system’s ability to exist in multiple states simultaneously. A commonly used analogy is how a coin can be both heads and tails before it is flipped, which may help illustrate the concept without diving into complex mathematics.
- Entanglement: This is the phenomenon where two or more particles become interlinked in ways such that the state of one particle can instantaneously affect the state of another, regardless of distance. Understanding entanglement is crucial for grasping modern quantum theories and their applications in fields like quantum computing and cryptography.
"Misconceptions about quantum theories often stem from attempts to reconcile them with classical interpretations. This simplicity can be misleading and overshadows the intricacies involved."
By dispelling these misconceptions and clarifying terminology, one embarks on a more informed journey through the intriguing worlds of quantum physics and mechanics. A solid grasp of these ideas not only enhances academic dialogue but also opens up channels for deeper exploration into the workings of our universe.
Educational Pathways in Quantum Science
In the rapidly evolving field of quantum science, understanding the available educational pathways is crucial for students and professionals alike. A robust education in quantum physics and mechanics not only equips individuals with the knowledge necessary to engage with cutting-edge research but also enhances their problem-solving skills, analytical thinking, and innovative capabilities. As the importance of quantum technologies grows, so does the need for well-trained individuals ready to tackle complex challenges in this domain.
Diving into this field often means navigating a labyrinth of academic programs and research opportunities. Each pathway offers unique benefits and considerations that cater to varying interests and career aspirations, ensuring a well-rounded approach to quantum education. Certainly, the journey isn’t always straightforward, but with the right guidance, anyone can find their niche.
Academic Programs
The academic programs available in quantum physics and mechanics are as diverse as the field itself. Most universities and institutions offer specialized degrees—bachelor's, master's, and Ph.D. programs—that focus on quantum theory, experimental physics, and engineering applications. Here are a few noteworthy aspects:
- Core Curriculum: Students typically engage with a core curriculum encompassing fundamental concepts of quantum mechanics, thermodynamics, and statistical mechanics. Some programs may also delve into advanced topics like quantum computing and cryptography.
- Hands-on Learning: Many programs emphasize lab work, allowing students to gain practical experience with real-world experiments. This practical exposure is essential, as it bridges the gap between theoretical knowledge and practical application.
- Interdisciplinary Focus: Quantum science overlaps with various fields such as computer science, materials science, and information theory. Educational pathways often promote interdisciplinary collaboration, which prepares students to think beyond their immediate discipline.
- Innovative Research Groups: Notably, many institutions boast research groups actively engaged in groundbreaking projects. This offers students a chance to participate in pioneering research, further enhancing their academic experience.
Choosing the right program can feel like picking a needle from a haystack. Prospective students should consider their career goals, available resources, and faculty specialties when making decisions. Seeking advice from mentors or current students can also provide clarity and direction.
Research Opportunities
In addition to academic programs, research opportunities significantly enrich the educational landscape in quantum science. Numerous avenues exist for students and professionals to engage in research that contributes to the advancements in this thrilling field:
- University Laboratories: Many universities operate specialized quantum laboratories where cutting-edge research is conducted. Engaging in these labs allows students to work alongside seasoned researchers, thereby enhancing their learning experience.
- Internships and Fellowships: Various organizations and research institutes offer internships and fellowships focused on quantum science, providing hands-on experience in real-world settings. These positions can lead to valuable connections and insights into the practical applications of theoretical knowledge.
- Collaborative Research Networks: Initiatives like Quantum Information Science and Technology (QIST) promote collaboration between universities and industry partners. Such collaborations foster an environment of shared expertise, allowing researchers to tackle complex problems more effectively.
- Graduate Research Projects: For those pursuing advanced degrees, involvement in significant graduate research projects can be pivotal. Many programs encourage students to have their research published, which can significantly enhance their academic and professional standing.
As opportunities for research continue to bloom within quantum science, they stand as a gateway to not just knowledge, but also an entry point into the exciting world of quantum careers. Engaging in research can pave the way for innovative ideas and solutions, essential for future breakthroughs in this field.
"The future of quantum science lies not only in understanding the theories but in the vibrant community of learners and researchers who exist within it."
The End: Synthesizing Quantum Discourses
In the grand scheme of scientific exploration, the distinction between quantum physics and quantum mechanics isn't just a matter of semantics. It echoes through classrooms, research labs, and philosophical discussions alike. Each term serves a purpose, collectively enriching our understanding of the universe at its most fundamental level. Within this article, we underscored the relationship and divergences of these two domains, aiming to provide clarity where confusions often arise.
The importance of this conclusion lies in its capacity to encapsulate the key findings of our exploration. It serves as a bridge between two seemingly disparate ideas, highlighting not only how they complement each other but also how they reflect the diverse layers of quantum theory. By clarifying these distinctions, we empower readers, including students, educators, and seasoned researchers, to navigate the intricacies of quantum science with a well-informed perspective.
This synthesizing effort sheds light on several core aspects:
- Clarity in Understanding: Distinguishing between quantum physics and quantum mechanics allows for a richer grasp of scientific consequences. It disentangles the complexities and helps in applying concepts correctly across various contexts.
- Practical Applications: The insights gained from understanding these fields can directly translate into advancements in technology including quantum computing and cryptography. It’s one thing to know the theory; it’s another to apply it wisely.
- Philosophical Reevaluation: The discussion prompts a deeper look at the philosophical implications that arise when one considers reality through the lens of quantum phenomena. Ideas about determinism, observation, and the nature of existence itself come into play.
Thus, synthesizing these discourses provides not just conclusions but a rich ground for further exploration and application in the evolving landscape of quantum science.