Essential Guide to Quantum Physics for Beginners
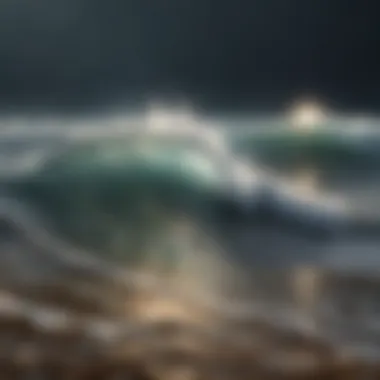
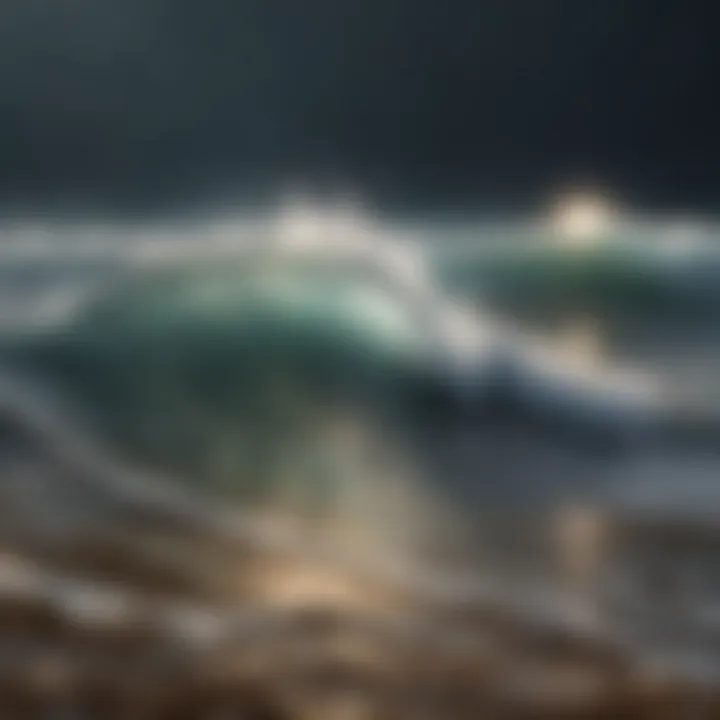
Intro
Quantum physics represents a fundamental shift in the way we understand the universe. At its core, this branch of physics studies the behavior of matter and energy at atomic and subatomic levels. It departs from classical mechanics, which adequately describes larger scales, in favor of principles that can seem surreal or counterintuitive. This article serves as a primer for those looking to grasp the basic concepts of quantum physics, exploring its key elements such as wave-particle duality, superposition, and entanglement.
Being increasingly relevant in various fields, from computing to cryptography, a foundational understanding of quantum mechanics can enhance one's comprehension of modern technology and ongoing scientific advancements. Whether you are a student, educator, or science enthusiast, this guide aims to present complex topics in an approachable format, highlighting both historical context and contemporary relevance.
Key Research Findings
Overview of Recent Discoveries
In recent years, quantum physics has witnessed remarkable breakthroughs. Research into quantum computing, for instance, has led to the development of more efficient algorithms and practical applications that could revolutionize data processing. Notable advances include Google's 2019 demonstration of quantum supremacy, where a quantum computer performed a calculation faster than any classical supercomputer could.
Significance of Findings in the Field
These discoveries have profound implications. Quantum mechanics now plays a crucial role in various scientific and technological domains. The development of quantum encryption methods promises unparalleled security for data transmission. Moreover, understanding phenomena like entanglement opens up new pathways for developing communication systems and networks that leverage quantum states.
"Quantum physics is not only about particles but also about how these particles interact and influence the greater universe."
Breakdown of Complex Concepts
Simplification of Advanced Theories
Critical concepts in quantum physics often pose challenges for newcomers. Here is a simplified overview of essential theories:
- Wave-Particle Duality: Particles such as electrons exhibit properties of both particles and waves, leading to complexities in observation and measurement.
- Superposition: A quantum system can exist in multiple states simultaneously until measured, highlighting the probabilistic nature of quantum mechanics rather than deterministic predictions.
- Entanglement: Particles can become interconnected, such that the state of one instantaneously influences another, regardless of distance. This phenomenon challenges our classical understanding of information transmission.
Visual Aids and Infographics
Incorporating visual aids can foster a better understanding of these concepts. Diagrams of wave functions or infographics depicting experiments, like the double-slit experiment, serve as valuable tools for visualization. Such representations can distill intricate details into graspable forms, aiding those who are new to the subject.
As we progress in this exploration of quantum physics, we aim to deepen your understanding and appreciation of this fascinating field that challenges the very nature of reality. It is not just an intellectual pursuit; it powers the technologies shaping our future.
Preface to Quantum Physics
Quantum physics marks a significant departure from classical physics, reshaping our understanding of the physical world. It is essential to study this field due to its foundational principles that govern the behavior of matter and energy at the smallest scales. These principles are not just theoretical; they have real-world impacts in technology, medicine, and even philosophy. Understanding quantum physics allows individuals to grasp fundamental concepts about the universe and enhances critical thinking.
Understanding Its Importance
The importance of quantum physics extends beyond academic interest. It plays a crucial role in many modern technologies. Devices such as lasers, semiconductors, and magnetic resonance imaging rely heavily on quantum phenomena. Understanding quantum physics can lead to innovative applications that might solve current technological challenges. Moreover, it lays the groundwork for fields such as quantum computing and quantum cryptography, which promise to revolutionize data processing and security. For learners and educators alike, exploring this discipline can spark curiosity and a deeper appreciation for science and its applications in everyday life.
Historical Background
Classical Physics vs. Quantum Physics
Classical physics, built on Newtonian mechanics, describes the motion of macroscopic objects. It is intuitive and can predict a wide range of phenomena with great accuracy. However, it fails to explain certain behaviors at the atomic and subatomic levels, especially when light and matter interact. That is where quantum physics emerges, providing explanations that classical physics cannot. For instance, classical models cannot account for the photoelectric effect, which revealed the particle-like behavior of light. The benefit of comparing these two frameworks lies in understanding the limitations of classical physics and the necessity of quantum models in describing reality more fully.
Key Figures in Quantum Theory
Many prominent figures have shaped quantum theory, bringing essential concepts to light. Albert Einstein, despite his classical roots, contributed significantly through his work on the photoelectric effect and the nature of light. Niels Bohr introduced the Bohr model of the atom, explaining electron orbits around a nucleus in quantized energy states. Additionally, figures like Werner Heisenberg and Erwin SchrΓΆdinger provided vital advancements in quantum mechanics, including the Uncertainty Principle and wave equations, respectively. Their contributions underscore the collaborative nature of scientific discovery and emphasize that no single individual could fully define the field. This section highlights the unique features of their theories and how each addresses gaps left by classical physics, advancing our overall understanding of quantum mechanics.
Fundamental Concepts of Quantum Physics
Understanding the fundamental concepts of quantum physics is essential for grasping how the universe operates at a subatomic level. These concepts reveal the limitations of classical physics, introducing ideas that seem counterintuitive, yet are supported by experimental data. By exploring these principles, one can appreciate the nuances of quantum mechanics and its profound implications across various scientific disciplines and technologies.
Wave-Particle Duality
Photons and Electrons
Photons and electrons serve as foundational elements in the study of quantum mechanics. Both exhibit properties of waves and particles, impacting theories in physics and providing insight into the behavior of matter and light. The key characteristic of photons is their role as carriers of electromagnetic radiation, while electrons are fundamental constituents of atoms. This duality allows for a better understanding of light and matter interaction. The adoption of these particles in this article is beneficial as they simplify complex phenomena through relatable characteristics.
One unique feature of photons is their speed, travelling at approximately 299,792 kilometers per second in a vacuum. Electrons, on the other hand, have mass and can exhibit a range of behaviors based on their energy states. Understanding their behavior leads to advances in fields like optics and materials science. Their dual nature highlights how classical perceptions are insufficient for explaining quantum realities.
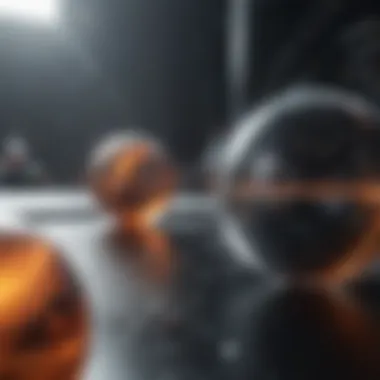
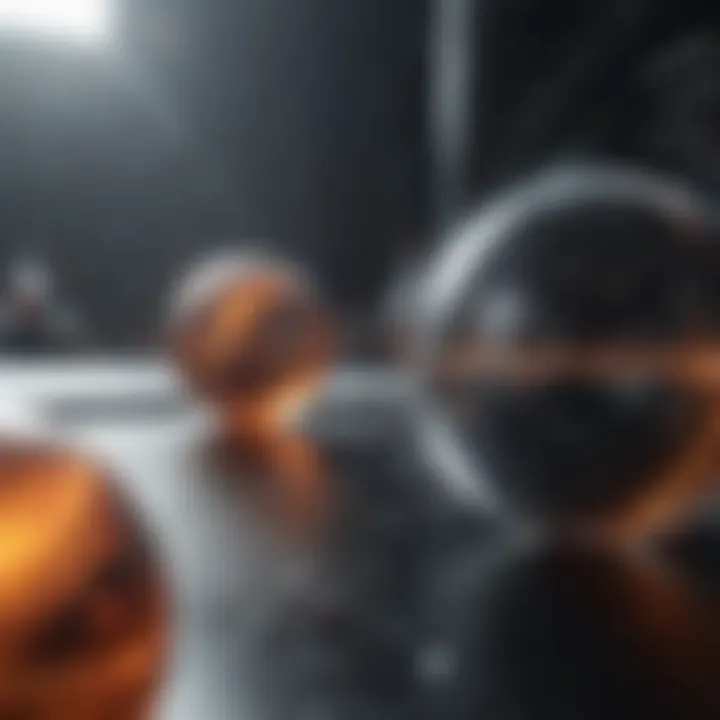
Experiments Demonstrating Duality
Experiments demonstrating wave-particle duality, such as the double-slit experiment, illustrate essential principles of quantum mechanics. This experiment reveals how particles can show interference patterns, suggesting they behave as waves under certain conditions. The key characteristic of such experiments is their ability to challenge and redefine our understanding of particle behavior. They are a crucial choice for this article as their findings are powerfully illustrative of quantum principles.
The unique feature of these experiments is their ability to showcase the probabilistic nature of quantum mechanics, offering insights into concepts like observation and measurement. Their advantage lies in their use as teaching tools for both students and educators, while the drawback remains the inherent complexity in interpretation, which can lead to misconceptions.
Quantum Superposition
Defining Superposition
Superposition is a fundamental aspect of quantum theory, representing the idea that a quantum system can exist in multiple states simultaneously until measured. This concept is crucial as it highlights the departure from classical physics, where objects exist in a single state. The key characteristic of superposition is its role in explaining phenomena such as quantum computing and quantum states.
Its inclusion in this article is beneficial due to its relevance to many modern applications and its ability to engage readers with its complexity. The unique feature here is the mathematical framework that supports superposition, allowing for predictions about the behavior of particles. However, this can also present disadvantages, as the abstract nature can be difficult for beginners to fully grasp without a solid mathematical background.
Schrodingerβs Cat Thought Experiment
Schrodingerβs Cat is a thought experiment designed to illustrate the peculiar implications of quantum superposition. In this scenario, a cat in a sealed box can be described as simultaneously alive and dead until observed. This thought experiment encourages deep contemplation about reality and observation in quantum mechanics. The key characteristic of Schrodingerβs Cat is its symbolic representation of quantum indeterminacy.
Incorporating this thought experiment into the article is beneficial because it evokes curiosity and helps clarify the principles of superposition. The unique feature of this scenario lies in its ability to provoke philosophical discussions about measurement and awareness. However, it can also confuse readers who may misinterpret it as a literal scenario rather than a conceptual tool.
Quantum Entanglement
Einsteinβs Critique
Einsteinβs critique of quantum entanglement is a notable highlight in the dialogue surrounding quantum mechanics. He famously referred to entanglement as βspooky action at a distance,β questioning the instantaneous nature of interactions between entangled particles. The key characteristic of Einstein's critique lies in its tension with the principles of locality and causality.
This critical perspective is essential to include in this article as it highlights the ongoing debates in the scientific community about the interpretation of quantum mechanics. The unique feature of Einstein's critique is its ability to spark discussions about the limitations of classical explanations and the nature of reality itself. However, this skepticism can also create confusion about the validity of quantum entanglement among beginners.
Applications of Entanglement
Applications of quantum entanglement are rapidly growing in various fields, such as quantum cryptography and quantum computing. The aspect of entanglement allows particles to be connected in such a way that the state of one instantly affects the state of another, regardless of the distance between them. The key characteristic of these applications is their potential to revolutionize technology and communication.
Including this topic in the article serves to emphasize the practical significance of quantum concepts. The unique feature of entanglement lies in its promise for secure communication and advanced computational capabilities. The advantages are numerous, but they come with the challenge of developing practical systems that can harness such quantum phenomena effectively.
Key Principles of Quantum Physics
The principles of quantum physics form the core of our understanding of the subatomic realm. This section highlights essential concepts that govern quantum mechanics and why they are indispensable in exploring the universe at the smallest scales. By grasping these key principles, one can begin to appreciate the implications that quantum physics has on both science and technology, as well as its philosophical underpinnings.
Uncertainty Principle
The Uncertainty Principle, formulated by Werner Heisenberg, is one of the most significant fundamentals of quantum mechanics. It states that certain pairs of physical properties, such as position and momentum, cannot be simultaneously measured with arbitrary precision. This means that the more accurately you measure one quantity, the less accurately you can measure the other.
Importance of the Uncertainty Principle:
- It challenges classical notions of determinism. In traditional physics, if you know a system's initial conditions, you can predict its future states. The Uncertainty Principle disrupts this straightforward view.
- It introduces the idea that at a quantum level, reality is inherently probabilistic. For example, particles do not have definite positions or velocities until they are observed.
Understanding this principle is crucial for comprehending the behavior of particles at atomic and subatomic levels, influencing fields from quantum computing to materials science.
Quantum Tunneling
Quantum Tunneling is a phenomenon that allows particles to pass through potential barriers that they would ordinarily not be able to cross. This occurs because particles exhibit wave-like properties, and their wave functions can extend beyond barriers, allowing a probability of the particle being found on the other side.
Significance of Quantum Tunneling:
- It plays a key role in nuclear fusion, where protons can tunnel through the electrostatic barrier created by their mutual repulsion.
- It is also essential in technologies such as scanning tunneling microscopes and certain types of semiconductor devices.
Understanding tunneling helps demystify processes that, while seemingly impossible classically, are integral to modern physics and technology.
Quantum States and Measurement
Quantum states are mathematical objects used to describe the condition or state of a quantum system. Each possible state corresponds to a specific set of probabilities for various measurable quantities. However, measuring a quantum state collapses it into one of the possible states, a process known as wave function collapse.
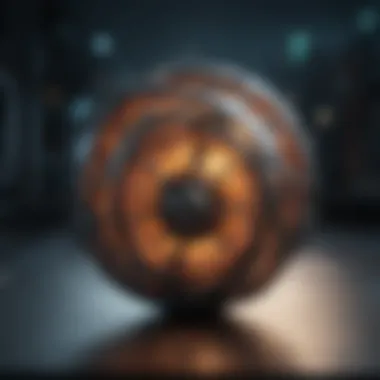
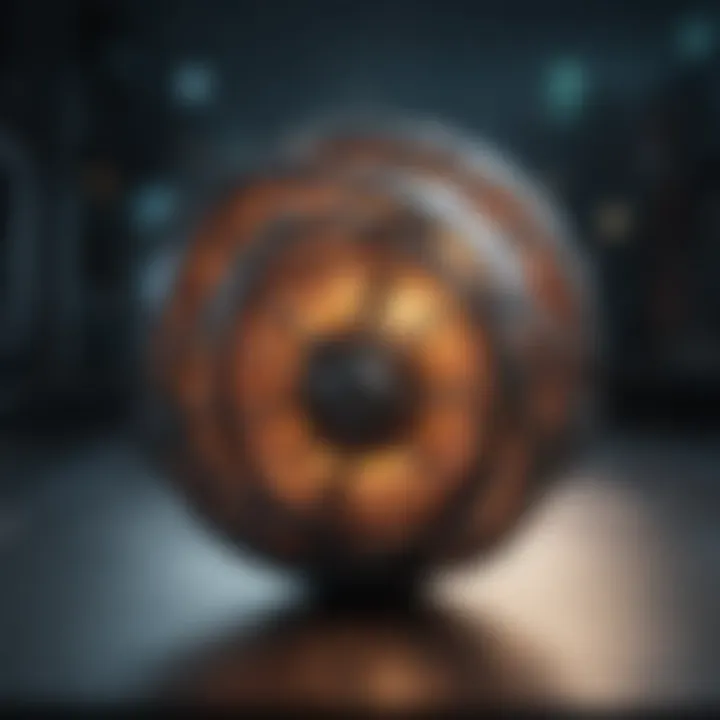
Key Considerations regarding Quantum States and Measurement:
- The concept of superposition implies that a system can exist in multiple states simultaneously until it is measured.
- The measurement problem poses philosophical questions regarding the role of the observer and the nature of reality in quantum mechanics.
- This plays a pivotal role in fields such as quantum computing, where qubits utilize superposition for processing information.
The way we interpret quantum states and their measurement shapes our understanding of reality and opens avenues for groundbreaking innovations in technology.
Understanding the key principles of quantum physics allows us to navigate through complex phenomena that govern the universe at a foundational level, paving the way for advancements in technology and scientific inquiry.
Interpreting Quantum Physics
Understanding quantum physics goes beyond grasping its fundamental concepts and principles. It involves unraveling the different ways physicists have sought to explain the unique and often paradoxical behavior of quantum systems. Interpretation offers insights into how we perceive these phenomena and shapes our understanding of reality. This section examines various interpretations that have emerged to make sense of quantum mechanics and highlights their significance.
Different Interpretations
Copenhagen Interpretation
The Copenhagen Interpretation is arguably the most influential interpretation of quantum physics. It emphasizes the role of measurement in determining the state of a quantum system. According to this view, until a measurement occurs, particles exist in a state of superposition, containing all possible outcomes. The act of measurement collapses this superposition into a single outcome, leading to definite results. This characteristic makes it a widely accepted option in the quantum community.
A key feature of the Copenhagen Interpretation is its acknowledgment of the inherent randomness in quantum systems. Unlike classical physics, where outcomes are deterministic, the Copenhagen approach accepts that the behavior of particles can only be described probabilistically. This influential idea shaped many experimental methodologies and increased understanding of phenomena such as quantum tunneling.
However, this interpretation also faces criticisms. Some argue that it leaves unanswered questions about the nature of reality and the observer's role. The subjective nature of measurement raises philosophical queries. Nonetheless, its practical utility in predicting experimental outcomes makes it a beneficial choice in many applications within this article.
Many-Worlds Interpretation
Another prominent interpretation is the Many-Worlds Interpretation. This framework suggests that every quantum event results in the branching of the universe into multiple, co-existing realities. Instead of collapsing into a single outcome during measurement, all possible outcomes occur, each in a separate, parallel universe. A key characteristic of this model is that it eliminates randomness and the concept of wave function collapse, promoting a deterministic view of quantum evolution.
Many-Worlds is a popular choice for those seeking a comprehensive understanding of quantum mechanics without invoking observer-induced changes. Its unique feature lies in its ability to explain quantum phenomena coherently without the convolutions necessary in other interpretations. However, challenges arise in conveying which world we experience and what implications this has for our perception of reality.
Philosophical Implications
The interpretations of quantum physics carry significant philosophical implications. They provoke thought on the nature of reality, the role of the observer, and the limits of human understanding. Questions arise about the existence of multiple realities and how measurements affect our perceptions of the universe. These discussions are crucial for students, educators, and researchers alike as they navigate through complex quantum theories.
Technological Applications of Quantum Physics
Technological applications of quantum physics play a significant role in modern science and engineering. They bridge theoretical concepts with practical innovations. This section delves into essential applications like quantum computing, quantum cryptography, and quantum sensors. These topics illustrate how quantum mechanics spurs advancements that can reshape technology. Understanding these applications helps grasp quantum physics' relevance to our daily lives and future developments.
Quantum Computing
Basic Principles
Quantum computing relies on qubits, which are the fundamental units of information in quantum systems. Unlike classical bits, which represent either zero or one, qubits can exist in multiple states simultaneously due to superposition. This property enables quantum computers to process complex problems much faster than traditional computers.
A key characteristic of quantum computing is its potential to solve problems considered infeasible for classical computing. For example, tasks such as factoring large numbers or simulating molecular structures would take impractically long times on standard machines. However, these can be managed efficiently with quantum algorithms like Shor's algorithm.
The unique feature of quantum computing is its ability to leverage entanglement, where qubits become interconnected. This interconnection leads to increased computational power and speed. Yet, building stable quantum computers remains a significant challenge due to decoherence, which disrupts the fragile state of qubits, limiting their practical use.
Current Developments
The field of quantum computing is rapidly evolving. Companies like IBM and Google are making strides in increasing qubit counts and developing error-correcting algorithms. These advancements aim to enhance the performance and reliability of quantum systems.
One notable aspect of current developments is the exploration of quantum supremacy. This concept suggests that quantum computers can outperform classical ones in specific tasks. Google's 2019 experiment achieved this milestone, generating interest and investment in quantum technologies. However, it's crucial to be cautious about the interpretations of these results as practical applications still require significant refinement.
The unique potential of quantum computing opens doors to diverse applications, like drug discovery and optimizing complex systems, though substantial technical hurdles remain before widespread adoption.
Quantum Cryptography
How It Works
Quantum cryptography leverages the principles of quantum mechanics to enhance security in data transmission. The primary method used is quantum key distribution (QKD). QKD enables two parties to generate a shared private key securely, allowing for encrypted communication.
A standout characteristic of quantum cryptography is its reliance on the uncertainty principle, which states that measuring a quantum state disturbs it. Therefore, any attempt to intercept or observe the transmitted key will alter its state, alerting the parties involved. This property offers a significant improvement over classical cryptography methods, which often become vulnerable to advanced computational attacks.
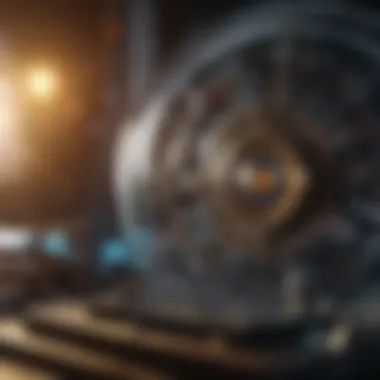
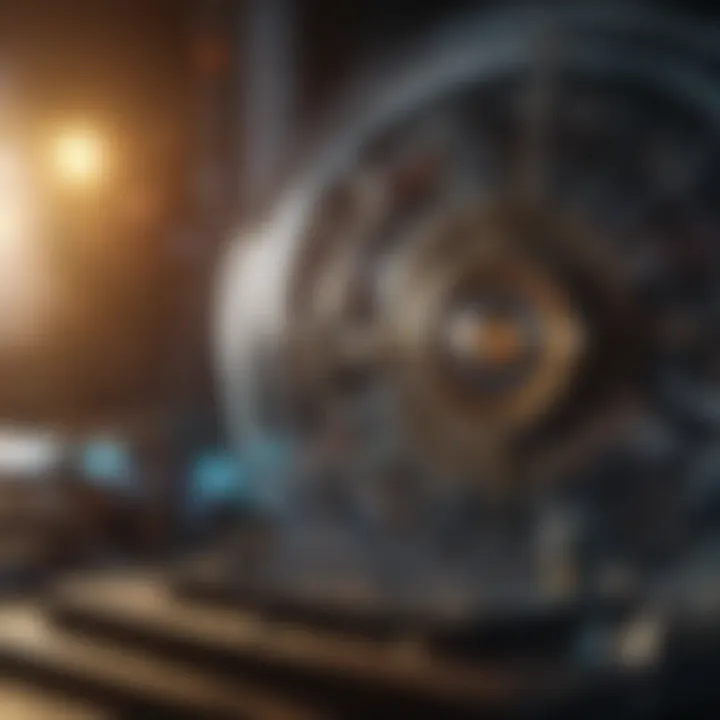
The unique feature of QKD is that it can mathematically guarantee the security of key exchange, which is crucial for sensitive information. Current implementations, however, face challenges related to distance limitations and technical complexities, which can hinder comprehension and application of this technology in real-world scenarios.
Real-World Applications
The real-world applications of quantum cryptography are continually expanding. Organizations dealing with highly sensitive information, such as financial institutions, defense systems, and healthcare databases, can benefit from quantum-secure communication. Several pilot projects have already proven the viability of quantum networks over limited distances.
A key characteristic of these applications is their ability to provide enhanced security against cyber threats. As global cyberattacks grow in sophistication, adopting quantum cryptography can significantly lower the risk of data breaches. However, the technology's reliance on specific conditions and infrastructure may limit its scalability and implementation in broader contexts.
The unique aspect of quantum cryptography lies in its forward-looking capability to secure future communications against potential quantum hacking threats.
Quantum Sensors
Applications in Measurement
Quantum sensors exploit quantum phenomena to enhance measurement precision, often surpassing classical sensor capabilities. They can measure various parameters, including time, magnetic fields, and gravitational forces, with unprecedented accuracy. The principle of superposition allows quantum sensors to obtain more precise measurements since they can capture information from multiple sources concurrently.
One key characteristic of quantum sensors is their ability to operate at the quantum noise limit. This helps in applications like navigation, geology, and even in medical imaging techniques. For instance, atomic clocks, which are reliant on quantum mechanics, are essential for global positioning systems (GPS).
The unique feature of quantum sensors is their potential to change the landscape of various scientific disciplines by allowing for more sensitive and accurate readings compared to traditional methods. However, they still face issues related to environmental sensitivity and cost, which can hinder wide-scale adoption.
Future Prospects
The future prospects of quantum sensors are promising. As research progresses, scientists are exploring methods to mitigate existing limitations and improve sensor performance. The development of portable quantum sensors could revolutionize fields like meteorology, where accurately predicting weather patterns is crucial.
One of the main characteristics of future developments in this area is miniaturization. Creating smaller and more efficient quantum sensors might lead to their integration into everyday technologies, such as smartphones or wearables.
Overall, while the innovations in quantum sensing hold immense potential, practical application still necessitates thorough understanding and significant investment to fully realize their capabilities in various sectors.
Quantum physics reshapes technology, bringing innovative solutions to contemporary challenges, from computing to secure communication.
Current Research and Future Directions
The field of quantum physics is not static; it is continuously evolving. Current research offers insights into phenomena that challenge our perception of reality, while future directions promise revolutionary advancements. Understanding ongoing experiments and upcoming challenges is crucial for anyone interested in the practical implementation of quantum principles.
Ongoing Experiments
Significant research projects are ongoing globally, aimed at uncovering the mysteries of quantum mechanics. These experiments often leverage cutting-edge technology and innovative methodologies. Some critical areas include:
- Quantum Computing: Researchers are testing various algorithms to harness the power of qubits for computation. Prominent projects, like those at Google and IBM, focus on creating scalable quantum systems that could outpace classical computers.
- Quantum Teleportation: Teams are exploring ways to transfer quantum information between particles over significant distances. Studies have noted successes in teleporting qubits using entangled particles, allowing for potential advancements in quantum communications.
- Testing the Foundations of Quantum Mechanics: Experiments related to Bell's theorem address the nature of quantum entanglement. Results support the violation of classical predictions, reinforcing the inherent non-locality of quantum systems.
These explorations not only reaffirm existing theories but also challenge the boundaries of our understanding of quantum physics. They showcase a dynamic interplay between theory and experimental verification.
Future Challenges
Despite the exciting prospects, several challenges confront researchers in quantum physics. These challenges could hinder progress or refine the field's trajectory. Key issues include:
- Decoherence: Maintaining quantum states is difficult, as environmental interactions threaten the stability of qubits. Developing error-correcting methods or finding materials that resist decoherence will be vital for practical quantum systems.
- Scalability: Building large-scale quantum computers involves intricate engineering challenges. Researchers must find ways to increase qubit numbers while controlling interactions accurately to avoid errors.
- Interdisciplinary Collaboration: The complexity of quantum physics often necessitates collaboration across diverse fields. Bridging gaps between physicists, computer scientists, and engineers is essential to create a robust framework for advancing quantum technologies.
In addressing these challenges, researchers can pave the way for functional applications in various sectors including computing, cryptography, and sensing technologies. The quest to conquer these obstacles will determine the speed and scope of quantum physics advancements in the coming years.
"The future of quantum physics is full of potential, but it necessitates rigorous inquiry and cooperation across disciplines to solve its many challenges."
As our understanding deepens and technological capabilities expand, the balance between experimentation and theoretical modeling will shape the future of quantum physics.
The End
In any discussion of profound scientific fields, the importance of understanding quantum physics can hardly be overstated. This article has provided a thorough introduction to basic concepts and principles that define quantum physics today. By examining topics from wave-particle duality to quantum entanglement, a clearer picture emerges of how these elements contribute to the wider landscape of science and technology.
The Impact of Quantum Physics on Science and Society
Quantum physics does not just occupy a niche in the world of theoretical science; it has pervasive effects on practical technology and societal perspectives. Its principles lay the groundwork for advanced applications in various fields. For instance:
- Quantum Computing: It promises to revolutionize computational tasks, offering capabilities unattainable with classical computers.
- Quantum Cryptography: It provides unparalleled security in communications, safeguarding sensitive information against potential threats.
- Quantum Sensors: These instruments achieve unprecedented accuracy in measurements, improving various scientific and industrial applications.
The philosophical implications of quantum theory force us to reevaluate our understanding of reality itself. Concepts such as superposition and uncertainty challenge traditional notions of measurement, causality, and even determinism. As we continue to delve deeper into ongoing research within this domain, a multitude of questions arise:
"How do we reconcile the probabilistic nature of quantum events with our everyday experience of a deterministic world?"
The impact of quantum physics, therefore, extends far beyond mere equations on a blackboard. It shapes technology, alters societal structures, and transforms our philosophical paradigms. As we ponder its future applications, it is crucial to consider not only the benefits it brings but also the ethical and societal considerations imperative for its advancement.