In-Depth Analysis of Quantum Physics and Its Impacts
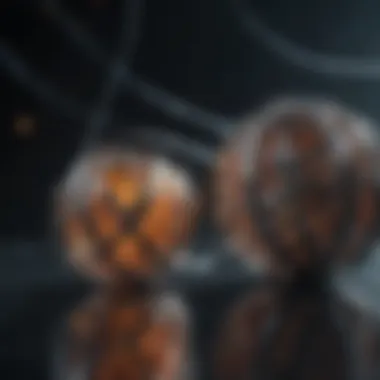
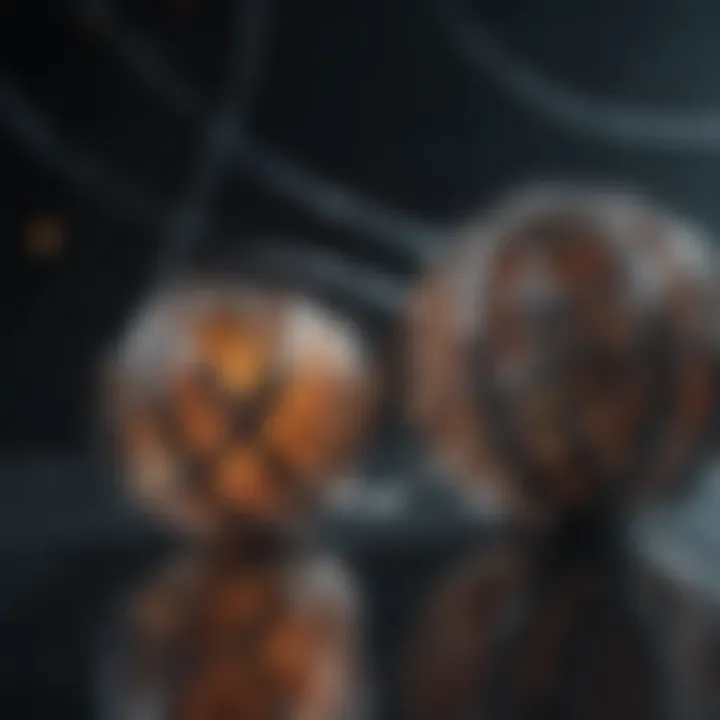
Intro
As we dive into the heart of quantum physics, we find ourselves standing on the shoulders of giants in the realm of science. This field, rich in intrigue, is not just about the small things but reshapes our understanding of the universe itself. Itโs a blend of the mind-boggling and the fascinating, exploring how matter and energy behave when examined closely. This examination spans from the grand cosmic structures down to the tiniest particles that make up everything around us.
Quantum physics sits at the crossroads of classical physics and the unknown, challenging our long-held beliefs about reality. The principles and phenomena that arise in quantum mechanics lead to profound implications far beyond the laboratory, influencing technology, philosophy, and our very perception of existence. Concepts like superposition and entanglement illustrate the complexity of this universe.
In this article, we will break down the significant discoveries made in quantum physics and articulate their impact on both science and real-world applications. Itโs not merely an academic pursuit; it holds the key to future technological advancements as diverse as quantum computing and secure communications.
As we embark on this journey through the peculiarities and revelations found within quantum physics, we'll aim to bridge the gap between complex scientific theories and their implications. Let's unravel this intricate tapestry together, make sense of the puzzling aspects, and appreciate their relevance in our ever-evolving world.
Prelims to Quantum Physics
Quantum physics is like peering through a keyhole into the intricate workings of the universe. The foundation of our understanding of the microscopic realm, it informs not only how subatomic particles behave, but also how these behaviors govern the broader world of technology and philosophy. With the likes of quantum computing and quantum cryptography at the forefront, itโs imperative to grasp the significance of this field, which often seems more like something out of science fiction than hard science.
In this context, it is crucial to consider the implications of concepts such as wave-particle duality and quantum entanglement. These principles challenge our perception of reality, nudging us to reconsider what we once thought was a complete understanding of physical laws. As we delve into the principles of quantum physics, we will unearth not only the underlying mechanics but also their consequences for technological advancements in our daily lives.
Defining Quantum Physics
Quantum physics can be defined as the branch of physics dealing with phenomena at atomic and subatomic levels. Unlike classical physics, which adheres to predictable laws, quantum physics introduces probabilities and uncertainties. Instead of just measuring where a particle is, quantum physics asks where it could be.
At its core, quantum physics breaks down into fundamental principles that govern the behavior of particles. For instance, when viewing light, it acts either as a wave or as a particle depending on how itโs observed. This duality poses questions that reach beyond mere physics, touching aspects of philosophy and even our understanding of reality itself.
Quantum theories stand apart through their reliance on complex mathematical formulations and experimental validations. Each element, from the behavior of an electron to the propagation of a photon, encapsulates the very essence of uncertainty and probability. Understanding this framework opens the door to technologies that may just redefine our world.
Historical Context
The journey of quantum physics begins in the early 20th century, at a time when classical physics seemed to explain almost everything around us. However, as scientists probed deeper into atomic structures, anomalies began to surface. Max Planck's introduction of quantized energy levels in 1900 marked the first crack in the classical facade, suggesting that energy, rather than flowing smoothly, occurs in discrete packetsโor quanta.
In 1905, Albert Einstein took a giant leap by extending Planckโs ideas to explain the photoelectric effect, showcasing how light itself consists of these discrete packets. This insight shifted the scientific paradigm, paving the way for further breakthroughs.
The development of quantum mechanics percolated through the scientific community in the following decades with contributions from Niels Bohr, Werner Heisenberg, and Erwin Schrรถdinger, among others. Notably, Bohrโs model of the atom brought clarity to atomic structure, while Schrรถdingerโs wave equation helped unify wave and particle concepts using a mathematical framework.
Each step in this historical progression laid the groundwork for todayโs quantum applications, establishing a rich tapestry of scientific inquiry that continues to evolve. As we journey through the following sections, itโs essential to appreciate how these historical milestones shaped our modern understanding of reality and paved the way for groundbreaking advancements in technology.
Core Principles of Quantum Physics
The core principles of quantum physics serve as the backbone of this fascinating field. Distinct from classical physics, these principles help explain the peculiar behavior of matter and energy at the microscopic level. Understanding these fundamental concepts is not merely for academic curiosity; they lay the groundwork for advancements in technology, from quantum computers to secure communication systems. Moreover, delving into these principles facilitates a deeper comprehension of reality itself, leading to new philosophical considerations about the nature of existence.
Wave-Particle Duality
Wave-particle duality is a central tenet of quantum physics, illustrating that particles such as electrons exhibit both wave-like and particle-like properties. Take, for instance, the famous double-slit experiment, where light behaves as a wave when unobserved, creating interference patterns. But when measured, light behaves like a particle, hitting the detection screen in discrete packets called photons. This dual identity challenges the classical idea of particles existing independently and presents the notion that the observer's influence plays a role in determining outcomes.
"In the world of quantum physics, reality is often a matter of perspective."
This principle not only captures the imagination but also has practical implications in fields like optics and quantum computing. The ability to switch between these states could lead to revolutionary technological advancements.
Quantum Superposition
Superposition refers to the ability of quantum systems to exist in multiple states simultaneously until observed. Think about Schrรถdinger's cat, a thought experiment where the cat is both alive and dead until someone opens the box to check. This peculiar phenomenon is not merely a theoretical concept; it has real-world applications, notably in quantum computing, where qubits can represent 1, 0, or both at the same time. This feature enhances computational capabilities significantly, allowing for processing complex problems much faster than traditional computers.
Quantum Entanglement
Quantum entanglement is another critical principle, where particles become intertwined in such a way that the state of one instantly informs the state of another, no matter the distance between them. Imagine two distant friends who always finish each otherโs sentences, regardless of the miles apart; this peculiar connection defies classical logic. Experiments confirm that entangled particles can communicate instantly, prompting discussions on the implications for information transfer and even theories of faster-than-light communication. The applications of entanglement go beyond theory and may lead to advancements in secure communication and quantum networks.
Heisenberg's Uncertainty Principle
Heisenberg's Uncertainty Principle asserts that there is a fundamental limit to how precisely we can measure certain pairs of properties of a particle, such as position and momentum. The principle aptly signifies the limitations of classical measurements, providing a sense that at a quantum level, nature is inherently uncertain. This realization reshapes our understanding of determinism in physics. In practical terms, it poses a challenge, especially in fields requiring precise measurements, including quantum computing and particle physics, where scientists must navigate this uncertainty to derive meaningful conclusions.
These core principles are not mere academic abstractions; they are the very fabric of how we understand the universe's most intricate mechanics. For students, researchers, and professionals, grappling with these concepts can open up new fronts in exploration and innovation.
Mathematical Foundations of Quantum Physics
In order to grasp the intricate concepts of quantum physics, it's essential to understand its mathematical underpinnings. Mathematics serves as the backbone of quantum theory, providing the tools necessary to describe phenomena that defy classical intuition. Rather than merely representing physical systems, mathematical frameworks allow us to predict outcomes, analyze behaviors, and comprehend the complex interactions inherent in microscopic realms. These foundations help bridge the gap between abstract ideas and tangible experiments, allowing researchers to explore phenomena that would otherwise remain elusive.
Quantum State Representation
The representation of quantum states is crucial in the study of quantum mechanics. A quantum state encapsulates all the information regarding a system's properties and behaviors. Quantum state can be described mathematically using vectors in a complex Hilbert space. For example, consider a single electron. One can represent its state as ( |\psi\rangle ), which might include parameters like its position, momentum, and spin.
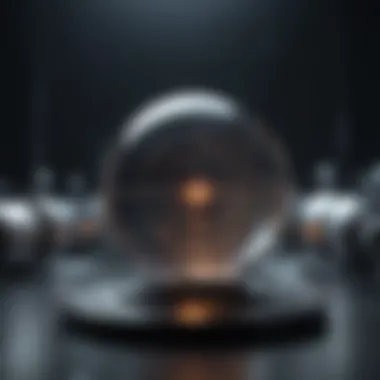
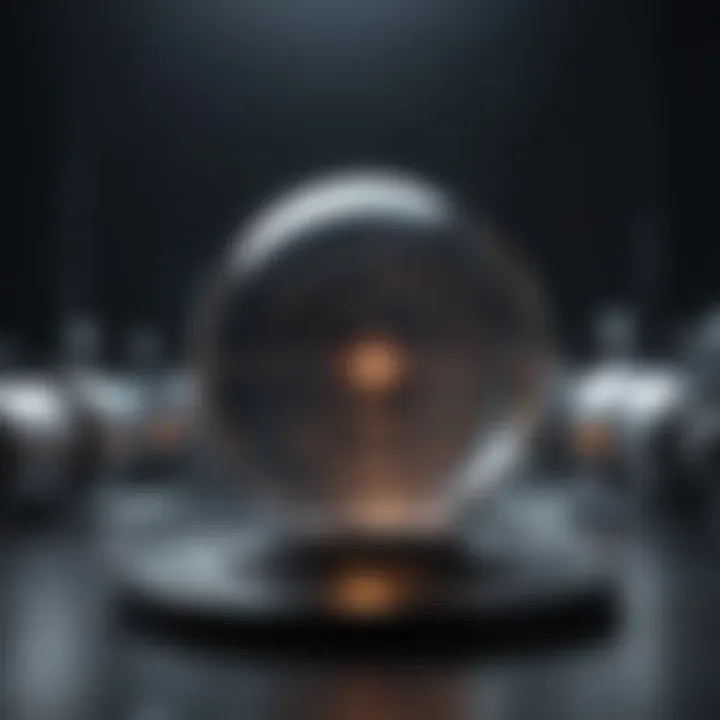
An important aspect of these representations is that each quantum state can exhibit multiple possible outcomes, which directly relates to the concept of quantum superposition. For instance, the electron's spin can be simultaneously in a 'up' state and a 'down' state until measured. This duality is mathematically illustrated using linear combinations of basis states, presenting a nuanced understanding of how outcomes probabilistically unfold when observation occurs.
Operators and Observables
Diving deeper into quantum physics, we encounter operators, which play a pivotal role in quantum mechanics. An operator is a mathematical entity that acts on the quantum state vector. For instance, the position operator can determine where a quantum particle is likely to be found. Mathematically, applying an operator to a state produces another state, known as an observable. The expectation values derived from these operations help in predicting outcomes during measurement.
Common operators include:
- Position Operator (( \hatx \))
- Momentum Operator (( \hatp = -i\hbar \fracddx \))
- Angular Momentum Operator (which represents rotational motion)
A quintessential feature of observables is the non-commutative nature of operators. This often leads to uncertainty relationships reflected in Heisenberg's principle, where knowing one observable precisely limits knowledge of another. In this light, the interplay between operators and observables becomes crucial, encapsulating the essence of quantum behavior and measurement perspectives.
The Schrรถdinger Equation
At the heart of quantum mechanics lies the Schrรถdinger equation, a fundamental equation that describes how quantum states evolve over time. This equation establishes the relationship between the quantum state of a system and its energy, essentially governing the dynamics of the system.
In its time-dependent form, the equation can be expressed as: [ i\hbar \frac\partial\partial t |\psi(t)\rangle = \hatH|\psi(t)\rangle ] where (\hatH) is the Hamiltonian operator which represents the total energy of the system, incorporating both kinetic and potential energies.
The significance of the Schrรถdinger equation cannot be overstated. It allows us to predict the behavior of quantum systems, revealing how particles like electrons move through potential fields. Solutions to this equation yield wave functions, which serve as the basis for determining probabilities and expectations related to measurements. The myriad solutions often illustrate phenomena like tunneling, where particles traverse barriers they classically shouldn't be able to.
Key Insight: The Schrรถdinger equation does not merely describe quantum systems; it fundamentally reshapes our understanding of reality, blurring the lines between determinism and probability.
The power of these mathematical foundations lies in their ability to articulate the behaviors and characteristics of particles within the quantum realm. By delving into representation, operators, and core equations, one unveils a comprehensive roadmap that guides exploration in the intriguingly complex landscape of quantum physics.
Quantum Mechanics versus Classical Mechanics
In the grand scheme of physics, the schism between quantum mechanics and classical mechanics stands out as one of the most crucial distinctions. Each theory encapsulates a unique perspective on how the universe operates, yet they approach the realm of reality from entirely different angles.
Fundamental Differences
The heart of the contrast lies in how each framework explains the behavior of matter and energy. Classical mechanics, as fundamentally described by Isaac Newton, operates on principles that always assume predictability. It offers a deterministic outlook on the universe, where future states of a system can be precisely calculated based on its current state. For example, the motion of a baseball thrown through the air can be accurately predicted using classic equations of motion.
In stark contrast, quantum mechanics introduces a reality laden with probabilities. Here, particles like electrons exist in a blurry state where their positions and velocities cannot be determined simultaneously with precision (Heisenberg's Uncertainty Principle). Instead of definite paths or trajectories, quantum entities are described by wave functions, which illuminate the probabilities of finding a particle in a particular state at any given time.
This fundamental difference in approach signals significant implications:
- Predictability vs. Probability: Classical mechanics assumes that given the same initial conditions, the outcome will always be the same. Quantum mechanics, however, acknowledges that outcomes can vary widely even with identical conditions, incorporating a spectrum of possibilities.
- Scale of Application: Classical mechanics is effective for everyday observablesโthe size of a soccer ball, for instanceโbut fails to give accurate predictions at atomic and subatomic levels, where quantum mechanics prevails.
- Determinism vs. Randomness: In classical physics, cause and effect reign supreme. Quantum physics, on the other hand, delves into realms where randomness and fluctuations permeate the very fabric of reality.
Implications for Understanding Reality
The divergence between quantum and classical mechanics compels us to reconsider reality itself. While classical physics satisfactorily explains most macroscopic phenomena, the microscopic world presents a more intricate and chaotic dynamic. The implications of these differences stretch far beyond theoretical considerations: they inform how we view consciousness, perception, and even the framework of reality itself.
The world of quantum mechanics is not just a sample of curiosity; it presents a rich tapestry of implications that challenge traditional notions of reality.
- Nature of Reality: Quantum phenomena raise questions about the essence of existence. For instance, the idea of observer effectโthe principle that the act of measuring can alter a quantum systemโsuggests a reality that isn't merely passive but instead is deeply intertwined with consciousness.
- Philosophical Enquiries: This body of science leads to profound philosophical debates and inquiries. For example, concepts such as locality and nonlocality arise, challenging established ideas about how objects interact at a distance.
- Technological Transformation: Beyond philosophical musings, these quantum principles are propelling technological advancements. Applications in computing, cryptography, and telecommunications emerge directly from the understanding of quantum behaviors, heralding a new era of innovation.
Applications of Quantum Physics
Understanding the applications of quantum physics is essential, as this field of study is not just an abstract theoretical pursuit, but it has tangible implications that influence contemporary technology and day-to-day life. From computing to security, and even the realm of teleportation, quantum principles bridge the gap between our microscopic understanding of the universe and practical breakthroughs.
Quantum Computing
Quantum computing stands as a beacon of innovation, harnessing the principles of quantum physics to revolutionize processing power. Unlike classical computers that use bits as the smallest unit of data, quantum computers utilize qubits. This shift enables them to perform complex calculations at astounding speeds.
For instance, consider a classical computer tasked with factoring large numbers to decrypt data; it might take years to crack an encryption based on the smarts of traditional bit processing. In stark contrast, a quantum computer can potentially complete this in a matter of hours or even minutes, due to its ability to exist in multiple states simultaneously through superposition.
- Advantages of Quantum Computing:
- Increased computational speed for complex algorithms.
- Better optimization solutions for diverse industries, including logistics and finance.
- Revolutionizes fields such as drug discovery, optimizing molecular configurations at a rapid pace.
Yet, challenges loom large in this frontier. Noise and error rates in quantum circuits remain significant roadblocks. Thus, while the promise is substantial, the technology is still maturing.
Quantum Cryptography
Moving onto security, quantum cryptography takes advantage of quantum mechanics to create communication systems that are theoretically secure against eavesdropping. The pivotal concept here is quantum key distribution (QKD), which uses the principles of quantum entanglement to securely share encryption keys.
In practical terms, if someone attempts to intercept the transmission of a quantum key, the very act of observation alters the key's state, alerting both parties that the communication has been compromised. This characteristic builds a resilient barrier against potential cyber intruders.
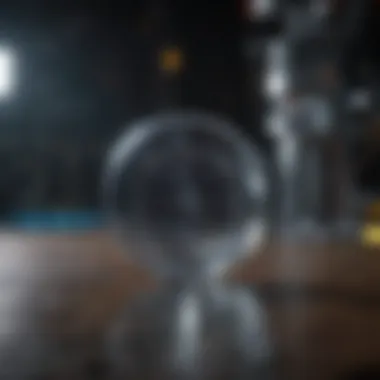
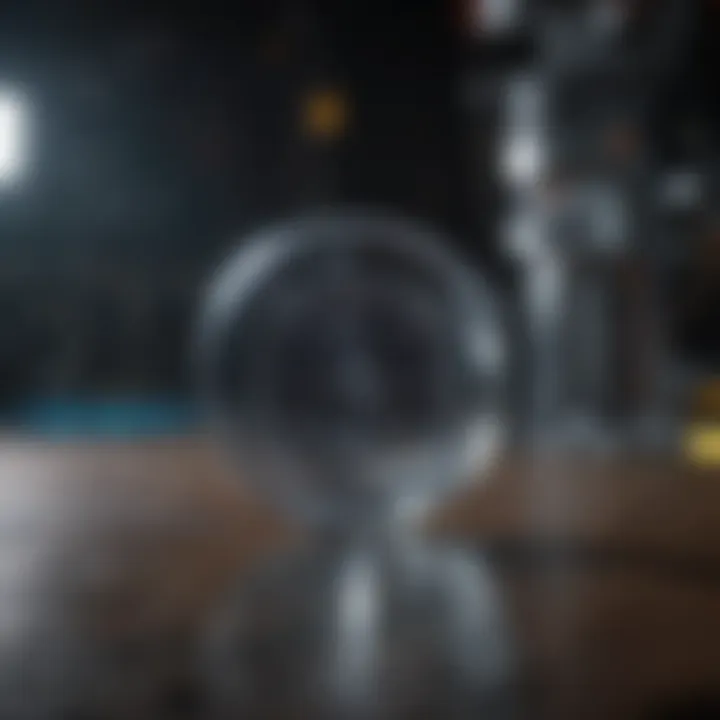
- Reasons to Consider Quantum Cryptography:
- Heightened security for sensitive data transmissions.
- Future-proofing communications against advancing hacking technologies.
- Protects privacy, making it attractive for military and governmental communications.
While the promise of unbreakable encryption is alluring, the technology's high costs and complex infrastructure need further research and development.
Quantum Teleportation
Quantum teleportation often scoots into science fiction discussions; however, it does have a firm grounding in quantum physics. This process involves transmitting quantum information from one place to another via entangled particles without transferring the physical particle itself. This isnโt about beams and sci-fi teleportation but showcases an intriguing capability.
What does this mean for practical applications? If we can successfully teleport quantum states, we can achieve faster-than-light communication and create non-local communication frameworks that challenge our traditional understanding of distance and information transfer.
- Implications of Quantum Teleportation:
- Potentially expands communication methods in a secure fashion.
- Opens avenues for novel data storage and computational architectures.
- Could lead to revolutionary changes in networking technologies.
Although still in its early stages of research, the theoretical foundations of quantum teleportation prompt scientists to reevaluate the architecture of future technologies, positing that classical boundaries are just warm-ups to the calisthenics of quantum understanding.
The major takeaway from quantum physics applications is that they bring theoretical ideas into the real world, showing their vast potential in shaping future technologies and methodologies.
As we delve deeper into understanding these applications, we'll see how they continue evolving, potentially changing our engagement with both technology and the very fabric of reality itself.
Philosophical Implications of Quantum Theory
The realm of quantum physics doesnโt merely touch upon the physical world; it digs into profound considerations about reality, perception, and existence itself. Within this framework, the philosophical implications of quantum theory take center stage, inviting scrutiny and contemplation. Itโs pivotal because it reframes our understanding not just of particles and waves, but of how we approach the fundamental nature of reality and the relationship between observers and observed phenomena. In an age where technology interlinks seamlessly with science, grasping these implications is essential for students, researchers, and professionals alike.
Reality and Observation
At the heart of quantum theory lies an unsettling question: what is reality? In classical terms, reality is often seen as an objective construct that exists independently of our observations. However, when we step into the quantum arena, this perspective shifts dramatically. Quantum phenomena suggest that the act of observation plays a crucial role in shaping reality itself.
Consider the famous double-slit experiment. When light or particles are directed through two slits, they create an interference pattern, suggesting wave-like behavior. Yet, if one attempts to measure which slit a particle passes through, this pattern collapses, and particles behave like solid balls. This raises a dilemma: does the act of observation somehow influence the outcome? Are the properties of particlesโlike position and momentumโmerely potential until they are observed?
This leads us to ponder:
- Is reality contingent upon observation?
- Do we create the universe by merely looking at it?
- What does it mean to know something, if knowing alters its state?
Such questions not only challenge scientific norms but also probe philosophical musings about free will, consciousness, and existence. They remind us that our understanding of reality may not be as straightforward as we once thought.
The Measurement Problem
The measurement problem in quantum mechanics adds yet another layer to these philosophical considerations. It deals with the perplexing behavior of quantum systems upon measurement, which complicates our intuitive grasp of physical reality. Essentially, the problem highlights the distinction between the quantum worldโincluding superpositions and probabilitiesโand the classical world, which is deterministic and stable.
When a quantum system exists in superposition โ where it can be in multiple states at once โ it defies our classical laws of physics. Only when a measurement is made does this superposition collapse into one definite state. But this collapse raises a question: how does the measurement process decide the outcome? Is an observer's consciousness integral to this process?
To dig deeper:
- What constitutes a measurement?
- Are consciousness and observer effect significant in determining a quantum state?
- Can non-conscious systems lead to the outcome of a measurement, or is there an inherent necessity for observation by conscious beings?
This philosophical puzzle has sparked extensive debates in the scientific community and beyond. Some suggest it's not just about quantum mechanics but about addressing the very fabric of existence.
The philosophical implications of quantum theory stretch beyond mere scientific debatesโthey demand a rigorous examination of our beliefs about reality, observation, and existence itself. As our understanding of quantum physics deepens, so must our philosophical inquiry into what it means to observe, measure, and ultimately, to exist in a universe dictated by the rules of quantum mechanics.
Recent Discoveries in Quantum Physics
Recent advancements in quantum physics herald a significant shift in our comprehension of the micro world. These discoveries serve as a reminder that despite having a solid foundation of theories, the quantum realm remains robustly unpredictable and fraught with mysteries waiting to be unraveled. By examining the latest research and collaborations in the quantum arena, one can appreciate the boundaries pushed by modern science.
Cutting-Edge Research Developments
The landscape of quantum physics is undergoing rapid evolution, with groundbreaking research altering our understanding at its core. For example, scientists have recently made strides in utilizing quantum bits, or qubits, that can exist in multiple states simultaneously, which is fundamental to creating effective quantum computers. Researchers at Google and IBM, in their pursuit of quantum supremacy, are unveiling new algorithms within these quantum systems that may solve problems impervious to classical computing.
Moreover, experiments have been conducted to understand quantum teleportation more comprehensively. This is the transfer of quantum states over distances without physical movement of particles. In essence, a quantum state can be teleported from one point to another, maintaining its properties. Experiments reaffirm that this phenomenon isn't just some far-flung theory but a tangible aspect of the quantum world.
Yet, the path forward is not devoid of complications. The inherent fragility of qubits means that quantum systems can easily become entangled with their environments, causing decoherence. This poses hurdles for creating stable quantum computers. Addressing these challenges is crucial for harnessing the incredible power of quantum mechanics.
Collaborations in Quantum Research
What is remarkable is the collaborative effort observed among researchers globally, which has led to a rich tapestry of findings. Institutions such as the Massachusetts Institute of Technology (MIT), Stanford University, and various international universities are pooling their resources and expertise together. The advent of quantum information science has not merely focused on individual breakthroughs but has also paved a path for cross-disciplinary dialogues.
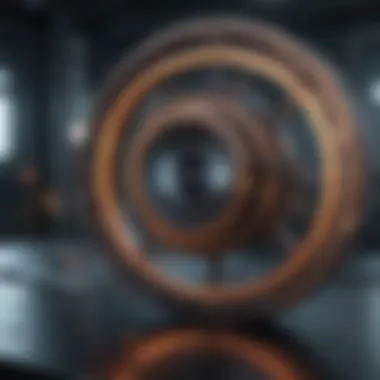
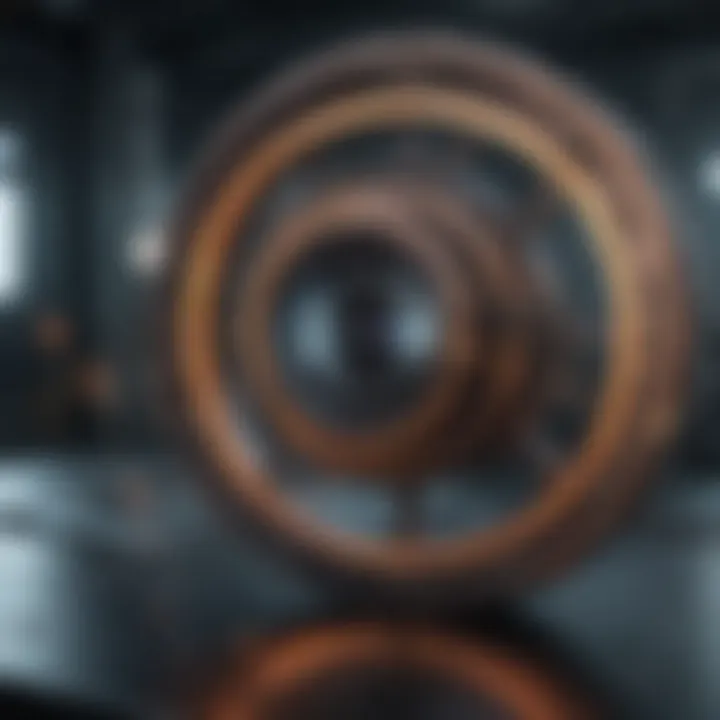
In particular, partnerships between industries and academic institutions have birthed initiatives aimed at addressing relevant real-world problems through quantum technology. For instance, collaborations between entities like the European Organization for Nuclear Research (CERN) and various tech firms exemplify how public-private partnerships can catalyze advancements in both fundamental research and practical applications. This unity fosters an environment where ideas can flourish unimpeded.
"The collective effort in quantum research allows for an exchange of knowledge, pushing the boundaries of what is possible in this fascinating field."
As various organizations align their goals around quantum technology, investigating quantum networking and enhanced security protocols becomes increasingly feasible. The essence of these collaborative endeavors lies not solely in accelerating the pace of research but also in augmenting the depth of understanding.
In summary, the recent developments and collaborative efforts in quantum physics are redefining the limits of what is achievable. The integration of various disciplines and the strategies employed demonstrate a unified approach in tackling the enigma of the quantum world.
Challenges and Limitations of Quantum Physics
In the realm of quantum physics, understanding the myriad of challenges and limitations is pivotal. While this branch of science opens doors to remarkable innovations and advancements, it also presents substantial hurdles that researchers must navigate. The complexity of quantum systems, the nuances of experimental setups, and the constraints inherent in theoretical frameworks all contribute to a landscape that can be daunting. Here, we will explore the specific elements of these challenges, their implications, and the considerations they raise for scientists and enthusiasts alike.
Experimental Challenges
When it comes to experimental science, quantum physics is no walk in the park. One of the primary considerations is the need for extreme precision in measurements and controls. Quantum systems are highly sensitive to environmental changes. Even the slightest disturbance can lead to decoherence, making it crucial for researchers to maintain stringent conditions. This often means working in ultra-high vacuum chambers or employing advanced cooling techniques to minimize noise and fluctuations.
Moreover, constructing experiments that effectively isolate and manipulate quantum states is a task akin to threading a needle in a haystack. The necessity of using specialized equipment, such as lasers and superconducting circuits, adds layers of complexity and cost. Importantly, these factors can limit the repeatability of experiments, which is a cornerstone of scientific validation. It's not uncommon for a well-planned experiment to yield results that differ from theoretical predictions due to these practical issues, creating a discrepancy that needs to be addressed.
Additionally, the scale at which quantum phenomena manifest poses its own set of challenges. For instance, observing quantum entanglement requires working with systems that are often on a minuscule scale, which can make practical experimentation quite cumbersome compared to the macroscopic systems we're used to in classical physics.
โMany of the problems faced in quantum experimentations stem from the delicate nature of quantum states themselvesโmanipulating these states to observe phenomena is inherently challenging.โ
Theoretical Limitations
While experiments breathe life into theories, the theoretical frameworks of quantum physics are also not without limitations. One major hurdle lies in the interpretation of quantum mechanics. Multiple interpretations exist, from the Copenhagen interpretation to many-worlds, each posing different implications about reality and observation. This plethora of interpretations can lead to confusion among students and researchers trying to grasp foundational concepts.
Another theoretical limitation is the challenge of unifying quantum mechanics with general relativity. While both theories hold up in their respective realms, merging their principles into a coherent framework remains tantalizingly elusive. This lack of unification can lead to gaps in our understanding, particularly in extreme conditions such as those found in black holes or during the Big Bang.
In addition, computations involving quantum systems often rely on approximations as pure analytical solutions become unwieldy. This adds uncertainty to predictions made on the behavior of complex quantum systems. The challenge of calculating quantum states and interactions accurately necessitates the development of sophisticated mathematical tools and computer algorithms, which can sometimes be limited by current technology.
Understanding these challenges and limitations does not diminish the grandeur of quantum physics; rather, it underscores the immense intricacies that research in this field entails. As scientists work toward overcoming these hurdles, the potential for breakthroughs and deeper insights into the nature of reality remains vast.
Future Prospects of Quantum Physics
The future of quantum physics is a realm bubbling with potential, imagination, and challenges that are yet to be addressed. In discussing this topic, we shine a light on how the foundations laid today could lead to seismic shifts in technology, our understanding of the universe, and even in day-to-day living. This examination is not just an academic exercise; it crosses into practical territories that could reshape industries and daily life alike.
Potential Breakthroughs
Quantum physics stands at the crossroads of breakthrough discoveries that could revolutionize multiple fields. Some potential breakthroughs include:
- Quantum Computing: Traditional computers, while powerful, have limitations bound by classical bits. Quantum computers, leveraging qubits, promise exponential increases in processing power. This could lead to unimaginable speeds in data processing and problem solving. From full-scale simulations of complex systems to advancements in AI, quantum computing holds immense promise.
- Quantum Communication: Envision a world where communication is utterly secure. Quantum key distribution allows for unhackable transmission of information, an essential development in an era where data breaches have become commonplace. This could protect sensitive information ranging from financial transactions to personal health records.
- Quantum Sensing: By utilizing quantum states to measure physical quantities with unprecedented precision, quantum sensors may provide insights into gravitational fields, time, and temperature like never before. Imagine being able to measure subtle variations in the Earth's gravitational field, potentially unlocking secrets to deeper scientific inquiries.
"Assembling these quantum technologies isnโt merely about advancements in machinery but the very way we interact with reality itself."
These breakthroughs, woven into the fabric of ongoing research and collaboration efforts, point to a monumental shift not just in technology but in how we perceive and engage with the world.
Quantum Integration into Everyday Life
When we consider the integration of quantum principles into our daily lives, it may come as a surprise that the start of this incorporation may already be upon us. Even in its infancy, this integration already hints at profound implications for society.
- Healthcare Innovations: The use of quantum technology in medicine could include better imaging techniques that allow for earlier and more accurate diagnosis of diseases. Moreover, quantum-enhanced drug discovery can drastically reduce the time and cost associated with bringing new medications to market.
- Smart Cities: Quantum sensors can contribute to smarter urban environments. For example, these sensors could optimize energy use, improve traffic management, and enhance public safetyโall crucial elements in creating sustainable cities.
- Consumer Electronics: As quantum technology matures, we may find quantum devices breaking into the consumer market. The possibility of quantum-enabled gadgets could change how we interact with technology daily, from personal devices to household appliances.
- Environmental Monitoring: Quantum tools could provide more accurate data about climate change, helping in the fight against environmental crises. Monitoring pollutants at an unprecedented precision might allow for timely responses to ecological threats.
As we navigate through these potential advancements, it's essential to ponder ethical questions, standards, and regulations that should accompany these technologies. Ensuring that the benefits of quantum advancements are accessible and equitable across society will be part of the ongoing discourse.
In summary, the future prospects of quantum physics illustrate a dynamic and evolving landscape where theoretical pursuits could lead to practical applications. As the boundaries between quantum science and everyday life blur, it's evident that the implications, both immediate and long-term, warrant our attention for years to come.
Ending and Reflections
In wrapping up our exploration of quantum physics, it's pivotal to highlight not just what we've discussed, but truly grasp the importance of these complex ideas in modern scientific discourse. The overarching principle of quantum mechanics is that reality, at a foundational level, is much stranger than our everyday experiences would suggest. This conclusion opens doors to not only scientific but also philosophical dialogues, raising questions about observation, reality, and the nature of knowledge itself.
The journey through wave-particle duality, superposition, and entanglement isnโt merely academic; it paints a picture of the intricate dance of particles that rules the universe at its core. Understanding these concepts can fundamentally shift how researchers approach problems across various fields, from computing to cryptography.
Summarizing Key Takeaways
To distill the essence of our discussion, several key takeaways emerge:
- Quantum Mechanics Trumps Classical Logic: The non-intuitive features such as entanglement and superposition show that particles can exist in multiple states simultaneously, inherently challenging classical understandings of physics.
- Measurement Matters: The act of observation isnโt passive; it actively shapes the state of quantum systems. Heisenberg's Uncertainty Principle underscores this by indicating limits on our ability to measure certain pairs of properties.
- Applications Galore: The practical implications of quantum physics extend far beyond mere theory; they drive advancements in technology, including quantum computing and secure communication channels.
- Philosophical Repercussions: The implications raise ontological discussions about the nature of reality, pushing us to re-evaluate our definitions of determinism and causation.
Thereโs much more beneath the surface of quantum physics than what first meets the eye. As we exit the subject, itโs essential to remember that understanding these principles can significantly impact our view of the universe and our place in it.
Encouraging Further Exploration
As with any complex discipline, the journey doesn't end here. To continue unraveling the mysteries of quantum physics, consider the following paths:
- Join Online Communities: Platforms like reddit.com offer vibrant discussions where enthusiasts and experts alike share insights and queries about the latest discoveries and theories in the field.
- Engage with Literature: Books like Quantum Physics for Dummies and The Quantum World can provide foundational knowledge while diving deeper into the nuances of the subject.
- Attend Workshops and Seminars: Local universities often host public lectures on advances in quantum research. Participating in these can foster connections with professionals in the field.
- Experiment with Quantum Software: Familiarize yourself with platforms that enable coding quantum algorithms, like IBMโs Qiskit or Microsoftโs Quantum Development Kit.