Exploring Quantum Loop Theory: Insights and Implications
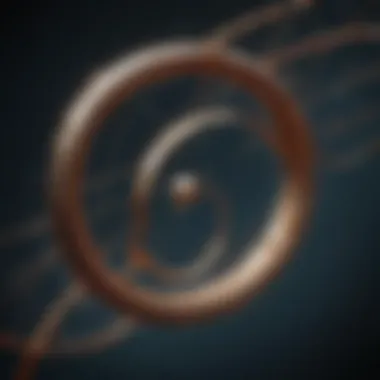
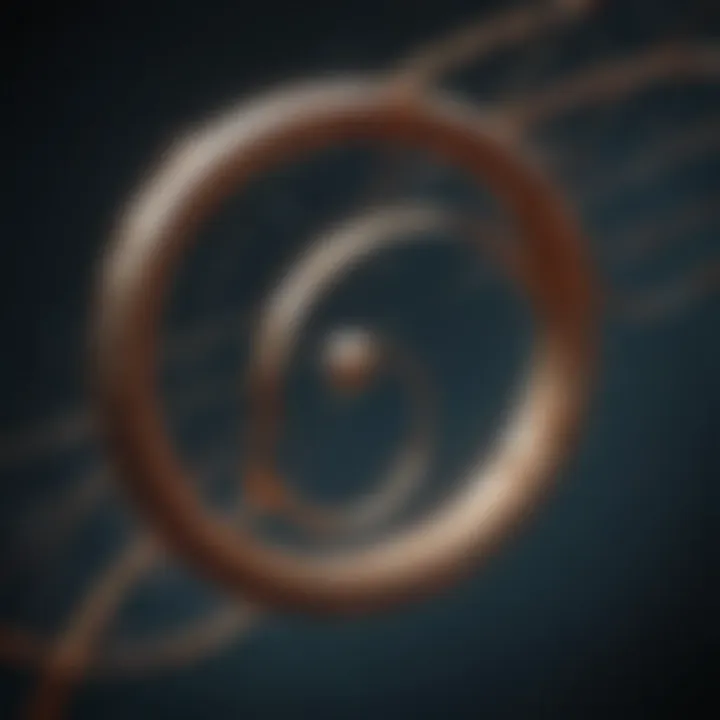
Intro
Quantum loop theory represents a pivotal approach to the intersection of general relativity and quantum mechanics. It aims not just to unify these foundational theories of physics but also to offer insights into the very fabric of spacetime. This article intends to dissect the core principles behind quantum loop theory, scrutinize its mathematical foundations, and delve into the profound implications it holds for our comprehension of the universe.
In the following sections, we will explore the key research findings in this field, identify recent discoveries, and analyze their significance. We will also simplify complex theories to enhance understanding, making the details accessible to both students and seasoned researchers. Furthermore, we will address ongoing challenges in experimental research and propose future directions for exploration, aiming for a broad yet detailed overview.
By examining the depth of quantum loop theory, readers will gain a comprehensive grasp of its contributions to modern physics, shaping the way we perceive space and time.
Prelude to Quantum Loop Theory
The significance of Quantum Loop Theory within theoretical physics cannot be overstated. This framework attempts to reconcile two seemingly incompatible realms of physics: quantum mechanics and general relativity. By exploring Quantum Loop Theory, researchers and enthusiasts alike gain insights into the elusive nature of gravity at the quantum level. This unification holds promises of deepening our understanding of the universe and encouraging new avenues of thought in fundamental physics.
In this article, we will delve into the frameworks that support Quantum Loop Theory while addressing its strengths and weaknesses. The major elements of this study include an examination of its foundational principles and an exploration of its implications for our broader comprehension of space and time. Like any scientific endeavor, critical examination enhances its relevance; hence, we will also discuss the benefits and considerations associated with this theory, highlighting its impact on modern physics.
Understanding Quantum Gravity
Quantum gravity remains a vital topic for scientists as it aims to explain how gravity behaves at the quantum level. This understanding contributes to answering fundamental questions related to black holes and cosmic phenomena. The challenge lies in integrating general relativityβour best explanation for the force of gravity on larger scalesβwith quantum mechanics, which governs subatomic particles. Quantum Loop Theory offers a possible pathway.
At its core, Quantum Loop Theory proposes that space-time is not a continuous fabric but rather constructed from discrete loops. This perspective reshapes our understanding of the fundamental structure of the universe, suggesting that at the smallest scales, space-time has a grainy quality akin to how matter exhibits quantization. This notion is compelling because it can potentially end the long-standing divide between the continuous view of spacetime of Einstein and the discrete nature prevalent in quantum mechanics.
Historical Context and Development
The journey towards understanding Quantum Loop Theory begins with the historical developments in physics. Since Einstein proposed his theory of general relativity, the challenge in physics has been to formulate a quantum version of gravity. Notable contributions came from physicists like Carlo Rovelli and Lee Smolin, who laid the groundwork for this theory in the 1990s.
Initial discussions focused on trying to combine existing theories rather than creating entirely new models. The development of quantum loop theory shifted in focus toward a more radical perspective. Early research laid the foundation for a new approach that would reveal new insights into the universe's structure.
One critical milestone in this journey was the recognition of spin networks, which is a central concept in Quantum Loop Theory. These structures depict how different quantum states relate to one another, leading to a better understanding of how gravitational interactions work at the quantum level.
The transition from traditional views of gravity to a quantum perspective has profound implications. As knowledge expands, so do the theories that explain cosmic phenomena, shaping future research direction.
In summary, the historical context surrounding Quantum Loop Theory provides essential insights into its evolution, unveiling a complex but rich narrative within the broader scope of physics. Understanding this history helps appreciate the nuances and challenges that have shaped contemporary theories.
Fundamental Concepts
Understanding the fundamental concepts of quantum loop theory is crucial for grasping its significance in theoretical physics. These principles serve as the building blocks that integrate the disparate realms of quantum mechanics and general relativity. They represent a framework through which scientists can explore the nature of the universe at both the cosmic and subatomic levels. The clearer our understanding of these concepts, the better we can navigate the complexities of unifying two of physics' foundational theories.
Basic Principles of Quantum Mechanics
Quantum mechanics rests on a set of distinct principles that govern the behavior of matter and energy on very small scales. Central to quantum theory is the idea of quantization, where physical properties like energy, momentum, and angular momentum take on discrete values. Other important principles include:
- Wave-Particle Duality: Particles, such as electrons and photons, exhibit both wave-like and particle-like properties. This duality challenges classical notions of particle behavior.
- Uncertainty Principle: Formulated by Werner Heisenberg, this principle states there are limits to how precisely we can know certain pairs of properties, like position and momentum, simultaneously.
- Superposition: This principle posits that quantum systems can exist in multiple states at once until observed. Measurement causes the system to collapse into one of the possible states.
These foundational aspects are essential for understanding how quantum loop theory seeks to describe gravitational interactions at quantum scales and the potential of developing a more unified framework of physics.
General Relativity and Its Limitations
General relativity, introduced by Albert Einstein, revolutionized our understanding of gravity as the curvature of spacetime caused by mass and energy. While it successfully describes large-scale phenomena, its limitations become noticeable when delving into extremes, such as at the centers of black holes or during the Big Bang. Key limitations include:
- Singularities: These points in spacetime, where densities become infinite, defy traditional laws of physics. General relativity does not provide a way to explain what happens at singularities.
- Quantum Scale Effects: General relativity fails to incorporate the principles of quantum mechanics, hence struggles to explain gravitational interactions at tiny scales effectively.
- Lack of a Quantum Framework: The inability to describe gravity using quantum principles highlights a significant gap in theoretical physics, making the case for a need for a more unified approach.
Recognizing these limitations is fundamental for exploring new theories like quantum loop theory, which aim to bridge the gap between the quantum world and gravitational phenomena.
The Need for Unification
The quest for unification in physics has long been a goal for scientists. The contradictions between general relativity and quantum mechanics present profound challenges. To develop a comprehensive understanding of the universe, unifying these theories is critical. The need for unification is driven by several factors:
- Comprehensive Nature of Reality: A single theory could seamlessly describe all physical phenomena across all scales, from cosmic structures to subatomic particles.
- Advancements in Technology: As experimental methods develop, the ability to observe and test the foundations of both theories simultaneously has become more feasible, urging the scientific community to explore unified models.
- Implications for Future Research: A successful unification could lead to new areas of research, offering insights into dark matter, dark energy, and the fundamental fabric of spacetime itself.
The exploration of quantum loop theory represents one of the most promising avenues towards addressing these crucial gaps in our understanding of gravity and quantum mechanics.
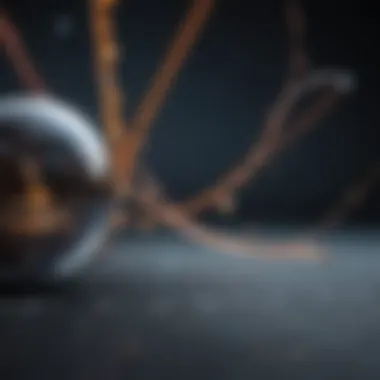
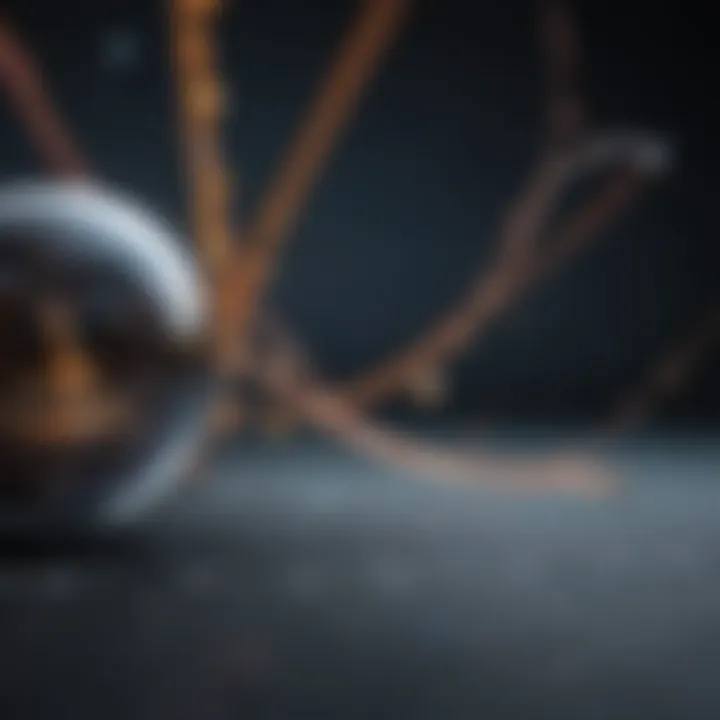
Unifying theories in physics hold the key to transforming our grasp of the universe and advancing the breadth of scientific inquiry.
By dissecting these fundamental concepts, we gain a clearer perspective on the necessity of developing a cohesive theoretical framework that encompasses both quantum mechanics and general relativity.
Mathematical Foundations
Mathematical foundations are essential to understanding the complex structure of quantum loop theory. This framework seeks to merge the principles of quantum mechanics with general relativity. A rigorous mathematical underpinning is necessary to formulate the theories accurately and to derive testable predictions. Without a solid mathematical basis, the ideas presented in quantum loop theory could remain hypothetical and lack empirical relevance. Thus, understanding its mathematical elements becomes crucial.
Tensor Calculus in Gravity
Tensor calculus serves as a vital tool in the study of gravitational fields within the realm of general relativity. In quantum loop theory, it plays a significant role in expressing the Einstein field equations, which describe how masses influence the curvature of space-time.
- General Concepts: Tensors allow us to work with multi-dimensional spaces, which is essential in considering the fabric of space-time itself. They provide a streamlined method for dealing with various physical quantities like tensors of stress-energy and the metric tensor.
- Without It: Not using tensor calculus would make it extraordinarily difficult to formulate the interactions between gravity and quantum fields. Therefore, grasping tensor calculus is not only beneficial but fundamentally necessary.
Lattice Quantum Field Theory
Lattice quantum field theory introduces a framework in which space-time is discretized. It allows physicists to model scenarios that would be computationally intensive if approached differently. The importance of this aspect within quantum loop theory cannot be overstated.
- Discrete Models: By placing particles on a lattice, researchers can simulate quantum fields more easily. This approach helps overcome infinities and divergences found in traditional quantum field theories.
- Computational Efficiency: Lattice structures enhance the ability to perform calculations numerically. They open the door to exploring physical phenomena that would otherwise be out of reach.
Overall, lattice quantum field theory is a crucial mathematical pillar that supports various predictions of quantum loop theory.
Spin Networks and their Significance
Spin networks form a core concept in quantum loop theory, representing quantum states of the gravitational field. They are a type of graph that encodes information about quantum geometry. Understanding spin networks helps link the abstract mathematical formalism of quantum loop theory to physical phenomena in the universe.
- Graphical Representation: Each node and edge in a spin network represents quantum states and relational interactions between them. This permits the analysis of complex interactions in a more manageable visual form.
- Quantum Geometry: Spin networks suggest that the geometry of space itself has a discrete nature at the quantum level. This concept has profound implications in how we understand gravity and the structure of the universe.
Spin networks not only enhance the foundational aspects of quantum loop theory but also invite new discussions regarding the nature of reality, geometrical structures, and quantum phenomena.
"The mathematical foundations of quantum loop theory are not just tools; they enable us to probe the very nature of reality at its deepest levels."
In summary, the mathematical underpinnings of quantum loop theory are critical. They provide the frameworks necessary to merge disparate physical theories into a coherent whole while offering insights into the nature of gravity, space, and time.
Implications of Quantum Loop Theory
The implications of quantum loop theory stand as significant in the ongoing discourse of physics. This theory proposes a way to reconcile quantum mechanics with general relativity, aiming to form a cohesive understanding of the universe. By investigating this area, researchers can uncover new insights into fundamental physical concepts, which may lead to transformative developments in various fields, including cosmology and theoretical physics.
Impact on Cosmology
Quantum loop theory offers a fresh perspective on cosmology, particularly in its approach to understanding the origins and evolution of the universe. One of the key implications is how this theory addresses the concept of the Big Bang. Traditional models suggest a singular start point; however, quantum loop theorists argue for a more nuanced viewpoint. They propose concepts such as a pre-Big Bang scenario or a cyclical universe, potentially eliminating singularities and offering explanations for cosmic inflation.
This reimagining of cosmological models introduces complexities like how space and time behave at small scales. The theory also enhances the understanding of cosmic structures, shedding light on dark matter and dark energy. These elements have puzzled scientists for decades, and insights derived from quantum loop theory could pave the way for a more accurate depiction of cosmic phenomena.
Black Holes and Singularities
The study of black holes is profoundly affected by quantum loop theory. Traditional understandings lead to paradoxes, primarily due to singularitiesβregions where space and time lose their meaning. However, quantum loop theory posits that these singularities may not exist as initially conceived. Instead, matter may undergo a phase transition that prevents infinite density.
This perspective opens possibilities for a more coherent understanding of phenomena like Hawking radiation, which challenges classical interpretations. Moreover, quantum loop theorists explore the implications regarding the information paradoxβwhether information that falls into a black hole is lost forever or if it can be recovered. Understanding this could revolutionize the fundamental nature of reality as well as our grasp on information theory in physics.
Revising the Concept of Space-Time
At the heart of quantum loop theory is the reconfiguration of how space-time is understood. Traditional physics views space-time as a smooth fabric, yet quantum loop theory suggests a more granular structure. This shift proposes that space is composed of discrete units or 'atoms of space'. This idea fundamentally alters interpretations of gravitational interactions and may lead to a new physics framework that merges quantum mechanics and relativity.
Such revisions have profound implications for our understanding of the universe. They challenge long-held notions in physics and compel researchers to rethink how we perceive fundamental forces. The insights gained from these considerations also resonate with philosophical questions concerning the nature of reality and our place within it. As this field progresses, ongoing research may provide clearer answers regarding the linkage between quantum mechanics and the universe's architecture.
"Quantum loop theory invites us to re-evaluate our understanding of the cosmos by proposing frameworks that challenge classical notions of space and time."
Current Research and Developments
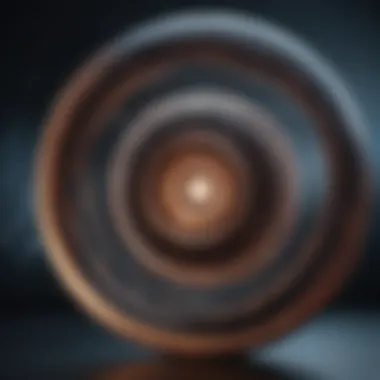
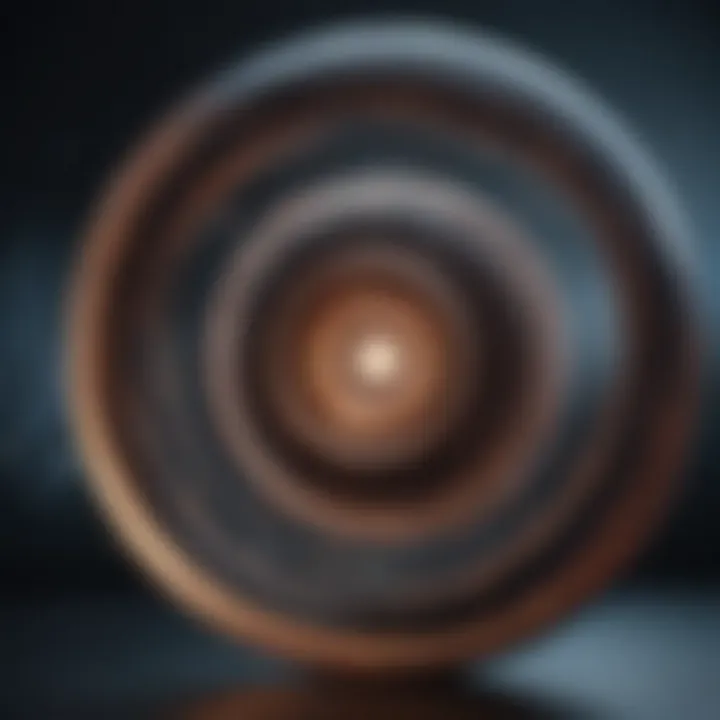
The study of quantum loop theory is continually evolving, reflecting the dynamic nature of theoretical physics. Current research plays a crucial role in expanding our understanding of this framework. By examining modern advancements, we gain insight into the ongoing efforts to consolidate quantum mechanics with general relativity. These developments not only deepen scientific knowledge but also pave the way for tangible applications in various fields.
Leading Theoretical Advances
Recent theoretical advancements have brought new perspectives to quantum loop theory. One significant trend is the exploration of non-perturbative approaches to quantum gravity. Researchers are investigating the role of loop quantization, which highlights the discrete nature of space-time at the Planck scale. This approach suggests that space-time could be fundamentally made up of loops rather than being continuous.
Another key advancement involves the refinement of spin foam models. These models attempt to encapsulate the dynamic evolution of quantum geometries. Understanding the subtleties of these models can lead to greater insights on how the universe behaves on both cosmic and subatomic scales.
Moreover, traditional methods such as the application of the path integral formulation to loop quantum gravity have garnered renewed interest. This method allows researchers to visualize gravity in the context of quantum field theories, bridging gaps previously thought insurmountable.
Experimental Validation Efforts
The experimental validation of quantum loop theory remains a significant challenge yet is critical for the theory's acceptance within the scientific community. Several groups have begun to focus on the identification of phenomena that could provide empirical support for loop quantum ideas. One promising direction is the investigation of black hole thermodynamics and the information paradox. Researchers are aiming to uncover whether loop quantum gravity can deliver predictions that differ from those of classical theories.
Additionally, the observation of potential signatures in cosmic microwave background radiation is being pursued. Such signatures might reveal non-trivial structures that arise from the quantization of space-time.
In laboratory contexts, scientists are also exploring highly sensitive measurement techniques. The goal is to test predictions regarding the quantum characteristics of space-time at measurable scales.
"Validation of quantum loop theory through experimental means is essential for establishing its credibility within the broader scientific community."
The multifaceted nature of research in quantum loop theory encapsulates a spectrum from theoretical exploration to experimental validation. These efforts not only strive to affirm the principles outlined by the theory, but also aim to inspire a more profound understanding of the universe.
Challenges and Criticisms
In the realm of theoretical physics, no concept emerges unscathed from scrutiny. Quantum loop theory, despite its elegance and power, faces numerous challenges and criticisms. Addressing these points is essential to understanding its relevance in the ongoing quest for a unified theory of physics. This section will delve into the mathematical challenges that arise when formulating the theory and explore the philosophical debates that accompany it.
Mathematical Challenges
One of the foremost hurdles for quantum loop theory lies in its mathematical structure. The theory utilizes concepts from both quantum mechanics and general relativity, which may seem incongruous at first. This integration leads to complex equations and formulations that do not easily lend themselves to solutions.
For instance, one notable challenge involves the renormalization process. In quantum field theories, infinities can appear in calculations, demanding a method to make sense of them. Quantum loop theory struggles with this, complicating efforts to produce experimentally verifiable predictions. Mathematicians and physicists are still working towards a comprehensive framework that resolves these issues.
Another aspect is the reliance on non-perturbative methods, which diverge from standard approaches in quantum field theory. While non-perturbative techniques have potential, they often lead to tedious and intricate computations. The vast number of degrees of freedom in the network states increases the complexity even further.
Lastly, one must consider the compatibility of quantum loop theory with existing mathematical frameworks. Concerns arise about whether the current mathematical tools are sufficient to fully capture its dynamics. Many researchers fear that without significant advancements in mathematical theory, fundamental aspects of the model may remain unexamined.
Philosophical Debates
Beyond mathematics, the implications of quantum loop theory invite philosophical scrutiny. One central issue is the nature of reality itself. How does quantum loop theory reconcile the discrete nature of space-time at the quantum level with the continuous fabric of general relativity? Questions arise about the ontological status of space and time. Are they fundamental entities or merely emergent properties of a deeper reality?
The concept of background independence deserves attention as well. Quantum loop theory posits that the geometry of space-time is dynamic rather than fixed. This raises questions about the traditional role of space-time in physical theories. Some argue that such radical rethinking complicates our understanding of physical laws. Others see it as a promising avenue for deeper insights into the universe.
Moreover, the interpretation of quantum mechanics itself becomes pertinent. Different interpretations may either support or contradict the principles behind quantum loop theory. The implications of observer effects, entanglement, and non-locality spark lively discussion among philosophers and physicists alike.
In essence, these debates do not merely reflect theoretical uncertainties. They highlight the importance of interdisciplinary dialogue between physics and philosophy. This collaboration may pave the way for substantial advances in our understanding of fundamental physics.
"The intersection of physics and philosophy is where the most fascinating questions about the nature of reality reside."
Comparative Analysis
In the realm of theoretical physics, the process of comparative analysis is essential for fostering comprehensive understanding. It enables researchers to evaluate the merits and limitations of various theories, helping to illuminate the strengths of specific models while revealing gaps or inconsistencies. This article delves into two prominent theories: Quantum Loop Theory and String Theory. Such an approach not only facilitates deeper insights into each theory but also enhances the discourse on unification of general relativity and quantum mechanics, a long-standing quest in physics.
Quantum Loop Theory vs. String Theory
Both Quantum Loop Theory and String Theory aim to reconcile the principles of quantum mechanics with general relativity. However, they approach this task from fundamentally different perspectives. Quantum Loop Theory focuses on quantizing spacetime itself, proposing that space is composed of finite loops, or "quantum loops". This model suggests that at the most fundamental level, space is a network of interconnected loops, eliminating the notion of smooth, continuous spacetime.
In contrast, String Theory suggests that the fundamental building blocks of the universe are not point particles but rather one-dimensional strings that vibrate at different frequencies. This theory posits that different vibrational modes of strings correspond to different particles. Thus, the forces of nature emerge from the interactions of these strings.
The implications of these differing approaches are profound. Some researchers argue that Quantum Loop Theory is more aligned with the principles of general relativity because it preserves the geometrical nature of spacetime, while others contend that String Theory provides a more elegant framework that incorporates additional dimensions and unifies all fundamental forces.
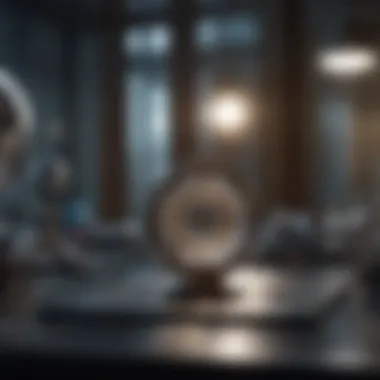
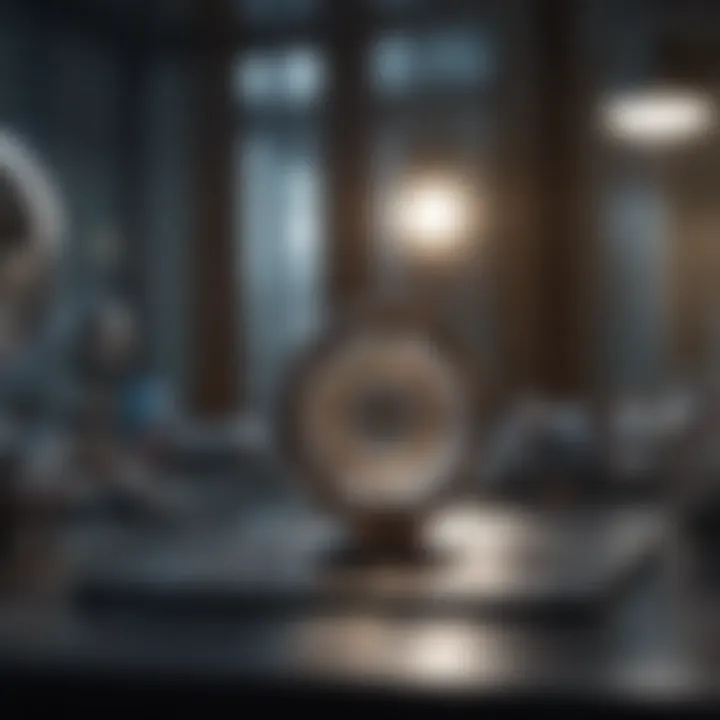
"While both theories aim for a grand unification of physics, their methodologies and implications vary significantly."
Assessment of Different Approaches
Assessing the diverse approaches within Quantum Loop Theory and String Theory reveals crucial insights into the current state of theoretical physics. Each theory presents unique advantages and challenges, shaping the landscape of research and experimentation.
- Quantum Loop Theory
- String Theory
- Advantages:
- Challenges:
- It provides a compelling framework for understanding black hole thermodynamics and singularities.
- It aligns closely with the classical description of spacetime in general relativity, maintaining a foundation that many physicists find appealing.
- The mathematical formulation of Quantum Loop Theory can be complex, often requiring advanced tools such as tensor calculus.
- There is ongoing debate concerning its compatibility with quantum field theory.
- Advantages:
- Challenges:
- It offers potential mechanisms for unifying all fundamental forces, including gravity, making it an attractive option for a Theory of Everything.
- The theory incorporates various dimensions, which could explain certain observed phenomena in particle physics.
- Experimental validation remains elusive due to the high energy scales involved and the need for extra dimensions that are not easily observable.
- The vast number of possible string theory vacua complicates the task of making definitive predictions.
By critically examining these two approaches, researchers can derive insights that help refine their theories, making comparative analysis a vital tool in advancing our understanding of the universe.
Future Directions
The future directions of quantum loop theory offer potent opportunities for advancing our understanding of fundamental physics. As researchers continue to explore the implications of this theory, there are several important aspects to consider. The integration of new experimental data with theoretical advances can lead to significant breakthroughs. Furthermore, these directions impact not only academic physics but also practical technological applications.
Potential Developments in Research
Research into quantum loop theory is at a pivotal moment. Current investigations focus on several potential developments:
- Improved Mathematical Models: Researchers strive to refine the mathematical frameworks underpinning quantum loop theory. This includes exploring new formulations that can address existing limitations and enhance predictive power.
- Experimental Validation: Efforts are ongoing to validate quantum loop theory through experiments. Major projects include observations related to gravitational waves and their implications on quantum gravity. Understanding how these phenomena align with theoretical predictions is vital.
- Interdisciplinary Collaboration: Combining insights from fields such as condensed matter physics and quantum information science enriches the study of quantum loop theory. Collaborations can bring about innovative methodologies and fresh perspectives, driving progress.
"The interplay of different scientific disciplines can reveal hidden connections that promote a deeper understanding of the universe."
These developments hold promise for not only theoretical advancement but also practical applications in technology and computations.
Long-Term Implications for Physics
The long-term implications of advancements in quantum loop theory could reshape our understanding of modern physics. Some notable areas include:
- Fundamental Physics Unification: Achieving a successful unification of quantum mechanics and general relativity could revolutionize our theoretical frameworks. It could lead to a more cohesive understanding of fundamental forces.
- New Insights into Cosmology: A well-established quantum loop theory may provide new insights into the early universe and cosmic inflation. Understanding these concepts can enhance our grasp of the universe's evolution.
- Technological Innovations: The principles arising from quantum loop theory might inspire new technologies, such as in quantum computing or quantum communications. Innovations derived from advanced theories can drive the development of new materials and methods.
Finale
The conclusion draws together the crucial insights garnered from the analysis of Quantum Loop Theory throughout this article. This framework stands at the intersection of quantum mechanics and general relativity, shedding light on profound questions about the nature of the universe. Understanding these insights is vital for appreciating how scientists are attempting to unify competing theories that have traditionally defined modern physics.
The first fundamental insight is the role of quantum loop theory in addressing the shortcomings of both quantum mechanics and general relativity. By exploring the concept of discrete space-time rather than a smooth continuum, this theory opens doors to potential new understandings of gravity. In doing so, it invites a reconsideration of basic assumptions about the fabric of the universe.
Second, quantum loop theory provides a unique perspective on phenomena such as black holes and the big bang. It challenges our existing theories and suggests alternative models that could better align with both observational data and theoretical requirements. Moreover, it impacts cosmological research by offering fresh approaches to puzzling questions such as the ultimate fate of the universe.
Finally, the implications extend beyond physics into various fields such as philosophy and technology. The exploration of Quantum Loop Theory raises questions about the nature of reality itself, pushing the boundaries of human understanding. Its ongoing development promises to explore new experimental techniques, potentially revolutionizing how we observe the universe.
The complexities within this theoretical framework embody the pursuit of knowledge in its purest form, marked by endless curiosity and diligence. As we surmise the journey laid out in this article, one must recognize the ongoing effort and intellectual rigor behind the exploration of such deep-seated topics.
Summary of Key Insights
- Unification of Theories: Quantum loop theory aims to bridge quantum mechanics and general relativity, offering a consistent narrative for gravitational phenomena.
- New Perspectives on Gravity: By examining space-time at a microscopic level, it introduces the concept of quantized space, which has potential implications for how we view cosmological structures.
- Impact on Black Holes: Insights into black holes challenge classical ideas of singularities, making space for more sophisticated frameworks that could redefine our understanding of these cosmic entities.
- Technological Relevance: The principles derived from quantum loop theory may lead to novel technologies in various fields, particularly in quantum computing and secure communication systems.
The Broader Impact on Science
The repercussions of quantum loop theory extend far beyond theoretical physics into broader scientific realms. The integration of insights from this framework can stimulate interdisciplinary collaboration across various scientific domains. As theoretical concepts gain experimental verification, they can refine existing technologies or even spawn entirely new fields of study.
- Philosophical Considerations: The paradigm shifts prompted by this theory force us to reconsider metaphysical ideas about reality, existence, and the interconnectivity of the universe.
- Interdisciplinary Influence: Its implications reach into areas such as mathematics, computer science, and even economics, where models of complex systems often borrow principles akin to those in quantum theories.
- Educational Impact: The exploration of these concepts fosters a new generation of thinkers, enticing students and early-career researchers to delve into complex problems with fresh perspectives.
In summary, the ongoing inquiry in quantum loop theory not only endeavors to consolidate physics but also elevates the broader quest for knowledge, intertwining various scientific disciplines and philosophies.