Quantum Computing and Cryptography: A New Frontier
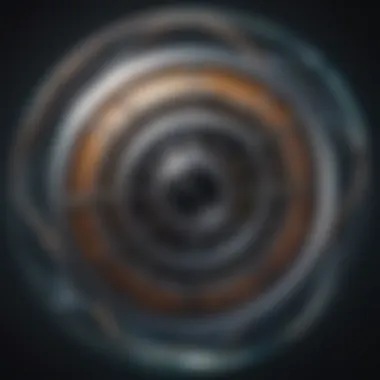
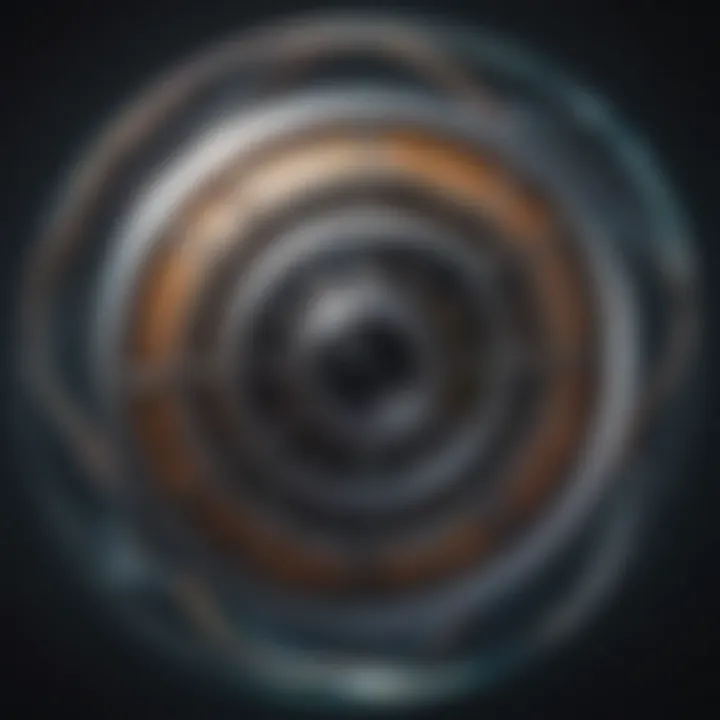
Intro
The rapid evolution of quantum computing signifies a paradigm shift in various fields, notably in cryptography. Quantum computing harnesses the principles of quantum mechanics to process information at an unprecedented scale and speed. As this technology advances, it poses unique threats to traditional cryptographic systems that underpin secure digital communication. This article examines the intricate relationship that exists between quantum computing and cryptography, illustrating how these two domains intersect and influence one another.
Key Research Findings
Overview of Recent Discoveries
Recent studies indicate that quantum computers have the potential to break widely used cryptographic algorithms, such as RSA and ECC, within feasible timeframes. Research shows that quantum algorithms like Shor's algorithm can factor large numbers efficiently, rendering many current encryption methods obsolete. These findings have intensified the ongoing discourse related to secure communications.
Furthermore, advancements in quantum key distribution (QKD) offer a novel approach to enhancing security. Through protocols established like BB84, parties can share secret keys with the assurance that any eavesdropping attempt would be detectable. This fluctuating landscape renders it essential for researchers to explore robust alternatives to conventional cryptographic practices.
Significance of Findings in the Field
The implications of these discoveries are far-reaching. For security professionals, the rise of quantum computing signifies a pressing need to reevaluate and reinvent security frameworks. Shifting to post-quantum cryptography is becoming critical to safeguard sensitive data. The urgency is underscored by governmental and academic initiatives aimed at developing quantum-resistant algorithms.
Breakdown of Complex Concepts
Simplification of Advanced Theories
To comprehend the impact of quantum computing on cryptography, it is beneficial to distill the complex theories underpinning both fields. At its core, quantum computing leverages qubits instead of traditional binary bits. This allows simultaneous processing of multiple possibilities, unlike classical computers that handle one task at a time.
Contrastingly, cryptography relies on mathematical problems that are easy to compute in one direction but significantly difficult to reverse. Quantum computing challenges this framework, as it can efficiently solve problems deemed hard under classical computation. Understanding these distinctions is necessary for grasping the overarching threats and opportunities at play.
Visual Aids and Infographics
In presenting the relationship betwen quantum computing and cryptography, visual aids can greatly enhance understanding. Infographics illustrating the mechanisms of quantum key distribution or the differences between classical and quantum algorithms can serve as valuable educational tools. Such resources can clarify the dynamism in this evolving field and help audiences visualize the tangible impacts of quantum advancements on cryptographic security.
"Quantum computing is no longer theoretical; it is a real and imminent threat to current encryption methodologies, necessitating immediate action in cryptography research."
This highlights the urgent need to understand both domains to ensure future security measures are robust enough to withstand quantum threats. As we continue to examine this intersection, it becomes evident that a proactive approach is essential for maintaining the integrity of digital communication.
Foreword to Quantum Computing
In the contemporary landscape of technology, the significance of quantum computing cannot be overstated. Its ability to process vast amounts of data through quantum bits, or qubits, represents a paradigm shift from classical computation. Understanding quantum computing is crucial as it lays the groundwork for exploring its implications on cryptography. The unfolding advancements in quantum technologies challenge existing security frameworks, demanding a profound reassessment of encryption methods designed to protect sensitive information.
Definition and Fundamentals
Quantum computing leverages the principles of quantum mechanics to perform calculations at exceptional speeds. Traditional computers process information in binary form, using bits that represent either a 0 or a 1. Conversely, a qubit can exist simultaneously in multiple states due to superposition. This characteristic enables quantum systems to explore numerous possibilities at once, leading to potentially exponential increases in computational power. Moreover, another fundamental aspect is entanglement, where the state of one qubit is directly related to the state of another, irrespective of the distance separating them. This unique trait is essential for the development of quantum algorithms that could outperform classical counterparts.
Historical Context
The initial concepts of quantum computing emerged in the early 1980s. Richard Feynman and David Deutsch were pivotal in proposing that quantum systems could simulate physical phenomena more effectively than classical computers. However, practical implementation was a distant challenge. The late 1990s marked the advent of significant algorithms that demonstrated quantum computing's capabilities. Shor's algorithm, developed by Peter Shor in 1994, illustrated the potential of quantum computers to factor large numbers efficiently, raising alarms about the future security of widely used cryptographic systems like RSA.
Since then, research and investment in quantum technologies have surged. Various institutions, including IBM and Google, are now actively developing quantum processors, pushing the boundaries of what is possible. This historical backdrop sets the stage for exploring how quantum computing interacts with cryptographic practices, highlighting the urgent need for reevaluating security measures in light of these advancements.
Principles of Quantum Mechanics
Understanding the principles of quantum mechanics is essential for comprehending the relationship between quantum computing and cryptography. Quantum mechanics introduces concepts that fundamentally change the approach to data, privacy, and security. These principles not only highlight the vulnerabilities in classical cryptographic systems but also pave the way for innovative solutions that can provide enhanced security. The interplay between quantum mechanics and cryptography is a significant area of research, as it directly impacts how information is secured in an increasingly digital world.
Qubits and Superposition
Qubits, or quantum bits, are the basic units of quantum information. Unlike classical bits that can exist only as 0 or 1, qubits can be in a state of superposition, meaning they can represent both 0 and 1 simultaneously. This property of qubits is crucial because it provides quantum computers the ability to perform multiple calculations at once.
The impact of superposition on computational power is profound. In cryptography, this allows quantum algorithms to tackle problems that would take classical algorithms an impractical amount of time. For example, with the ability to evaluate multiple possibilities at once, quantum computers can threaten established encryption methods that rely on the difficulty of certain mathematical problems. This duality of superposition means it can transform current cryptographic methods into increasingly insecure systems in the face of quantum advancements.
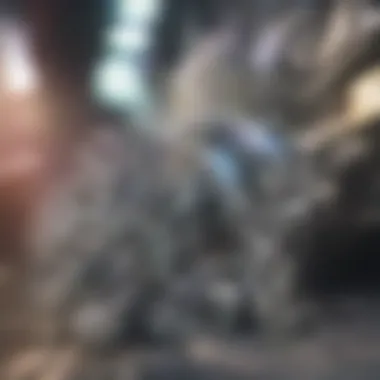
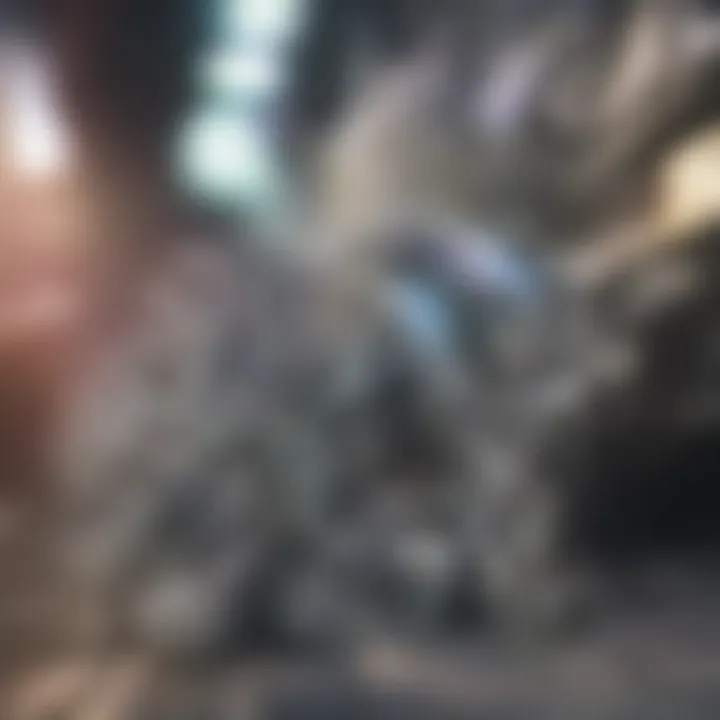
Entanglement Explained
Entanglement is another critical phenomenon in quantum mechanics, wherein pairs or groups of particles become interconnected in such a way that the state of one particle directly influences the state of another, irrespective of the distance separating them. This property is vital for many potential applications in quantum cryptography.
In terms of cryptographic security, entanglement can be utilized for secure key distribution. When entangled qubits are shared, any attempt to eavesdrop will disturb the state of these particles, thus alerting the communicating parties to the presence of an intruder. This not only enhances security but also addresses the fundamental issues present in classical cryptographic systems, where such disturbances often go undetected.
"Entanglement, as a foundational element of quantum mechanics, enables secure communication that the classical counterpart could never achieve."
The understanding of these principlesβsuperposition and entanglementβunderscores the potential and challenges faced in securing digital communications in a quantum age. As the landscape of both quantum computing and cryptography evolves, it is critical to stay informed and prepared for the implications that these principles may introduce.
Understanding Cryptography
Cryptography is a foundational element in securing information in todayβs digital landscape. As quantum computing emerges, understanding cryptography becomes increasingly important. It serves to protect data from unauthorized access and ensures the integrity and confidentiality of communications. By exploring cryptographic techniques, we can better appreciate how quantum phenomena may disrupt traditional methods and inspire new frameworks.
Historical Overview of Cryptographic Systems
Cryptographic methods have evolved significantly over centuries. From simple ciphers used in ancient times, like the Caesar cipher, to sophisticated algorithms found in modern systems, the history of cryptography highlights a constant quest for security. Notably, cryptographic practices were pivotal during wartime, where secure communication was vital. The growth of electronic communications in the 20th century led to the development of key management and complex encryption techniques, setting the stage for current practices.
Types of Cryptographic Techniques
Symmetric Encryption
Symmetric encryption is a form of cryptography where the same key is used for both encryption and decryption of data. This method is noteworthy for its speed and efficiency. It utilizes algorithms like AES (Advanced Encryption Standard), which offers strong security when properly managed. A key characteristic is that both sender and receiver must share the key in a secure manner. This requirement can be a challenge in large networks, but when implemented correctly, symmetric encryption can effectively safeguard data. Its main advantages include faster processing speed and lower resource consumption.
Asymmetric Encryption
Asymmetric encryption differs significantly as it uses a pair of keys: a public key for encryption and a private key for decryption. This technique is crucial for secure transactions, particularly on the internet. A highlighted feature is its ability to enable secure communications without the need to exchange secret keys beforehand. RSA (RivestβShamirβAdleman) is a popular algorithm in this category. Its benefits include improved security for online transactions, but it tends to be slower compared to symmetric methods, making it less suitable for large data volumes.
Hash Functions
Hash functions serve a unique purpose in cryptography. They take an input and produce a fixed-size string of characters, which is typically a digest that represents the input data. This feature makes them important for verifying integrity and authenticity. An essential characteristic of hash functions is their one-way nature; they cannot be reversed to reveal the original input. Common algorithms include SHA-256 (Secure Hash Algorithm). Their advantages lie in creating digital signatures and ensuring data integrity, but they are not used for encrypting data due to their irreversible properties.
Quantum Threats to Cryptography
In the evolving landscape of information security, quantum computing presents notable challenges to existing cryptographic methods. This section focuses on how quantum threats can undermine traditional security protocols, particularly those that rely on the mathematical complexity difficult for classical computers to solve. Understanding these threats is crucial for developing measures that can safeguard information in a quantum future.
Shor's Algorithm
Mechanics of Factorization
Shor's Algorithm significantly alters the approach to integer factorization. It efficiently factors large numbers, which is the cornerstone of many encryption techniques like RSA. The key characteristic of this algorithm is its polynomial time complexity. This means, unlike classical algorithms, which can take exponential time for large inputs, Shorβs Algorithm can compute factors in a manageable timeframe. For this article, this is a beneficial aspect because it highlights inherent vulnerabilities in widely used encryption systems. Its unique feature is the ability to utilize quantum superposition through quantum circuits, creating multiple calculation paths simultaneously. The main advantage is speed, allowing for quick solutions to what was previously considered hard problems.
Implications for RSA
The implications of Shor's Algorithm for RSA encryption are profound. RSA relies on the difficulty of factoring large prime numbers to ensure security. With Shor's Algorithm, RSA's foundational security principle is compromised. The essential characteristic of these implications is the feasibility of breaking RSA encryption, which could render countless secure transactions vulnerable to attack. This makes it a critical consideration in this article. Its unique feature is that it necessitates an urgent reevaluation of RSA's reliance on prime factorization for security. The disadvantage lies in the potential for widespread security failure unless faster adoption of quantum-resistant algorithms occurs.
Mitigation Strategies
Mitigation strategies become paramount as Shor's Algorithm poses significant threats. Several approaches can be employed to enhance security against these quantum threats. One major strategy is implementing post-quantum cryptography. This involves developing algorithms that are believed to be resistant to quantum attacks. The key characteristic of these strategies is their proactive approach to encryption, which is essential for maintaining trust in digital communications. Their unique aspect is the combination of mathematical techniques that do not rely on the same principles as current cryptographic systems. However, the challenge lies in the integration of these new methods into existing systems without compromising current security.
Grover's Algorithm
Speeding Up Searches
Grover's Algorithm introduces another layer of concern regarding symmetric encryption, especially in its capacity to speed up search operations. The ability to search an unsorted database in roughly the square root of the time is a hallmark of this algorithm. The key characteristic of this speed increase is that it effectively reduces the effort required to break symmetric key encryption. This is beneficial for this article as it illustrates another method by which quantum computing could potentially compromise traditional security measures. The unique feature of Grover's Algorithm is its structure, which allows it to influence the efficiency of brute-force attacks, thereby diminishing the security margin provided by current key lengths.
Effects on Symmetric Key Lengths
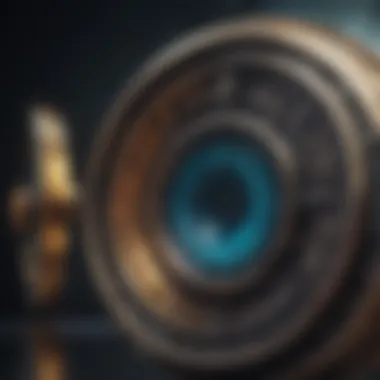
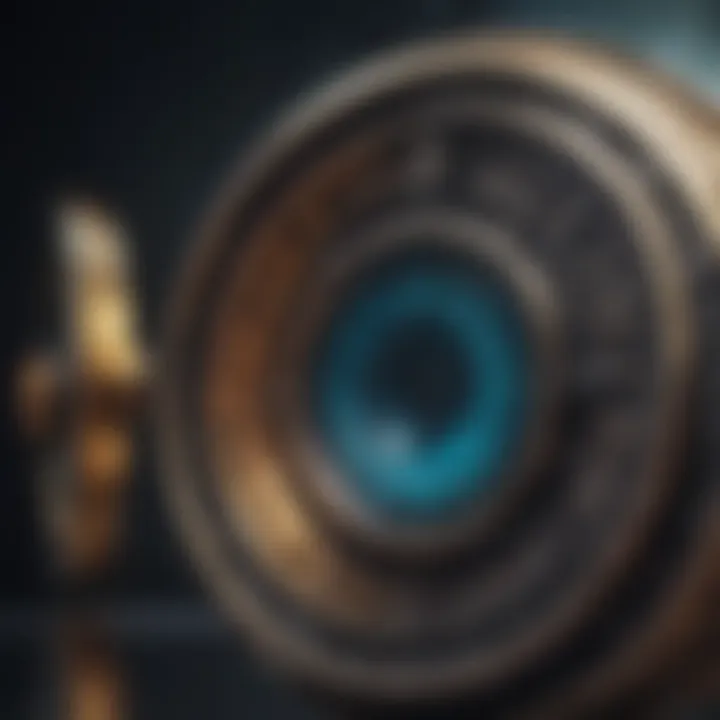
The effects of Grover's Algorithm on symmetric key lengths are critical to understanding the broader implications of quantum computing. Due to the enhanced search capabilities, the security afforded by symmetric keys is significantly weakened. The key characteristic is that a doubling of the required key length may be necessary to maintain security against quantum attacks. This is an important point for this article, as it indicates a direct response that the cryptographic community might have to undertake to preserve data integrity. A unique feature of this consideration is that while current standards may be viewed as adequate, the emergence of quantum capabilities may render them obsolete much faster than anticipated. The main disadvantage is the increased computational load and potential inefficiencies in updating existing systems.
The Role of Quantum Cryptography
Quantum cryptography represents a significant advancement in the field of information security. As we move further into an era defined by digital communication, leveraging the properties of quantum mechanics offers unique advantages that classical methods cannot provide. This section will delve into the mechanisms of quantum cryptography, particularly focusing on quantum key distribution which is foundational to secure transmission. The inherent characteristics of quantum systems not only enhance security but also present new challenges in implementation and infrastructure.
Prelude to Quantum Key Distribution
Quantum key distribution (QKD) forms the bedrock of quantum cryptography. Its primary goal is to enable two parties to generate and share a secret key securely, using the principles of quantum mechanics to ensure the key's integrity against eavesdropping. For instance, if a third party attempts to intercept the key exchange, their presence will be detectable through changes in the quantum states being transmitted.
BB84 Protocol
The BB84 protocol, developed by Charles Bennett and Gilles Brassard in 1984, is one of the first and most widely studied QKD protocols. The key characteristic of BB84 is its use of quantum bits, or qubits, which are transmitted in randomly chosen quantum states. This protocol enables a secure key exchange by utilizing properties of superposition and measurement in quantum mechanics.
A notable advantage of BB84 is its simplicity and robustness; the mechanism inherently detects eavesdropping attempts. However, it also has limitations, such as the necessity for a direct line-of-sight communication between participants, which can restrict its applications in certain environments. In practical implementations, factors like noise and distance can affect the efficient transmission of qubits, making the deployment of BB84 a topic of extensive research and development.
E91 Protocol
Developed by Artur Ekert in 1991, the E91 protocol introduces concepts of quantum entanglement into the framework of quantum key distribution. A key characteristic of the E91 protocol is that it allows two parties to share entangled qubits. The measurement results from these qubits exhibit correlations that are stronger than what classical physics would predict.
This unique feature not only enhances security but it also ensures any eavesdropping can be detected through altered correlations. One beneficial aspect of the E91 protocol is its adaptability to different communication environments since its reliance on entangled particles allows for sharing keys in a broader range of scenarios compared to BB84.
However, like BB84, the E91 protocol faces challenges. The generation and maintenance of entangled states are more complex and fragile than the transmission of single qubits, thus requiring sophisticated technological infrastructure. This often makes the practical deployment of E91 protocol less accessible compared to BB84, requiring further advancements in quantum technologies.
Benefits Over Classical Cryptography
The transition to quantum cryptography provides several notable benefits over classical cryptographic methods:
- Unconditional Security: The laws of quantum mechanics allow for a security model that is not based on computational assumptions, unlike classical cryptography.
- Eavesdropping Detection: Any attempt to intercept or measure qubits during the key exchange process can be detected, ensuring the integrity of the key.
- Secure Encryption and Decryption: The keys generated through quantum processes enhance the security of encrypted data, significantly mitigating risks associated with current threat models that challenge classical encryption systems.
In summary, quantum cryptography presents a transformative approach to securing digital communications, drawing from the principles and features unique to quantum mechanics. As research progresses and technologies evolve, the practical implementation of quantum cryptography may redefine how we approach data security.
Implementation Challenges
In the exploration of quantum computing and cryptography, understanding the challenges associated with implementation is crucial. These challenges influence not only the feasibility but also the effectiveness of deploying quantum technologies in existing systems. Various technical hurdles and infrastructure requirements must be addressed to successfully integrate quantum cryptography into real-world applications. This section will examine the specific elements that contribute to these challenges and their anticipated benefits.
Technical Limitations of Quantum Systems
Quantum systems, while promising vast potential, face several technical limitations that hinder their current usability. One significant issue is decoherence, which pertains to the loss of quantum states due to environmental interference. When qubits interact with their surroundings, they can lose their quantum properties, leading to errors in computation or communication. This makes maintaining coherence a primary concern.
Another challenge involves the error rates in quantum computation and communication. Until now, quantum devices have exhibited much higher error rates compared to classical systems. This affects the reliability of operations and necessitates the development of error correction techniques, which can increase the complexities of quantum systems.
Moreover, scalability presents a formidable challenge. Current quantum computers accommodate only a limited number of qubits. For practical implementation in cryptography, systems must scale to thousands or millions of qubits. Technologies like superconducting qubits or trapped ions require further innovations to achieve the needed scale, which is still in developmental stages.
These technical limitations not only impact the efficiency of quantum algorithms but also pose considerations in cost and accessibility. Manufacturers must invest heavily in research to find solutions, making widespread deployment a greater challenge.
Infrastructure Needs for Deployment
The deployment of quantum cryptography systems requires significant foundational infrastructure. One of the primary needs is the integration of quantum networks. Unlike classical networks, quantum networks rely on different principles, necessitating new types of communication protocols. This is essential for transmitting quantum keys securely.
Building quantum communication infrastructures, such as quantum repeaters, is another critical requirement. Quantum repeaters are necessary to extend the range of quantum key distribution beyond the limits of direct light transmission, which typically is about 100 kilometers. Without these repeaters, it becomes unfeasible to utilize quantum communication in many scenarios.
Additionally, physical security of quantum key distribution systems is paramount. Given that quantum keys can be intercepted, the environments in which quantum systems operate must be tightly controlled. This could involve advanced security measures in server farms, data centers, and communication points to protect quantum devices from tampering or theft.
It is also worth noting the training and education needs for professionals in the field. Expertise in quantum mechanics and cryptographic techniques is still developing. Therefore, establishing educational programs and training initiatives will be needed to cultivate a workforce capable of supporting quantum technologies.
"As quantum technologies evolve, proper infrastructure becomes as crucial as the quantum technologies themselves."
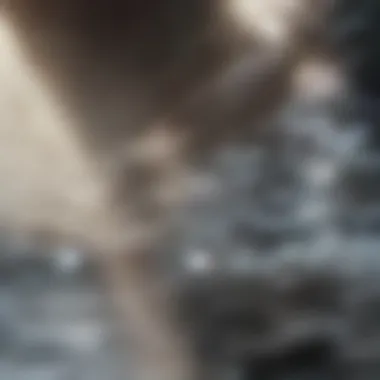
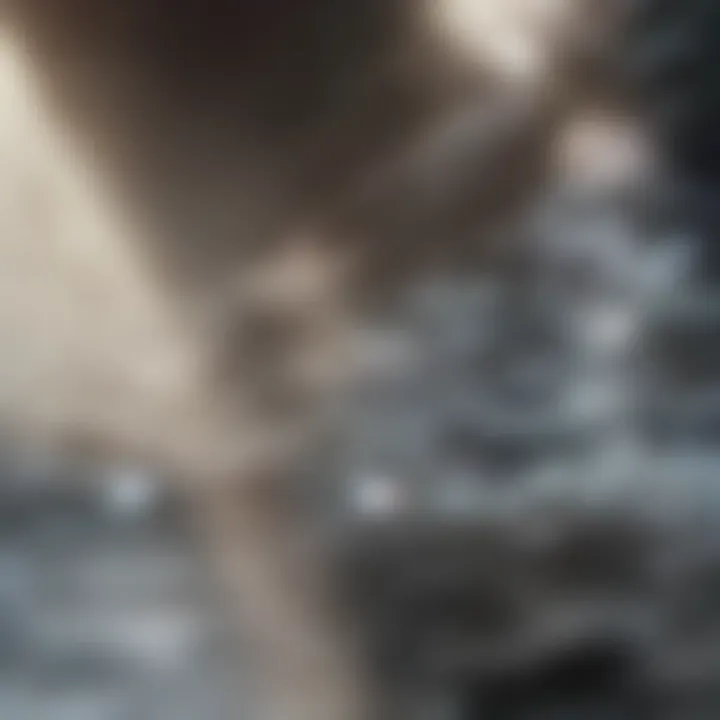
Future Prospects
The recognition of future prospects in the realm of quantum computing and cryptography is vital. The advancements in quantum technologies are not just theoretical; they promise to transform how we approach information security. This section assesses essential elements and benefits of these prospects, shedding light on the broader implications for security in a digital environment.
Predictions for Quantum Computing Advancements
As quantum computing technology matures, experts predict several significant developments. First, increased quantum processing power is anticipated. With this, more complex problems will be solved faster than classical computers can handle. For example, optimization problems that are currently time-consuming might see improvements in speed and efficiency.
Second, we expect to see developments in quantum error correction methods. Error rates in quantum computers pose a challenge. Solutions in this field will enable more reliable operations, thereby opening gateways for commercial applications.
Moreover, another prediction centers on the integration of quantum computing into current paradigms. Hybrid systemsβcombining classical and quantum computingβcould become common, enabling organizations to gradually adopt quantum technologies.
This blend will likely reduce risks associated with a complete transition to quantum systems. Furthermore, as more industries recognize the potential of quantum computing, advancements will enable tailored algorithms that address specific sector challenges, enhancing cybersecurity strategies.
Potential for New Cryptographic Algorithms
The development of new cryptographic algorithms tailored for quantum environments is essential. As quantum computers threaten existing systems, new algorithms must leverage quantum properties to ensure secure communications.
Various quantum-resistant algorithms are being researched. For instance, lattice-based cryptography shows promise as it is believed to be secure against quantum attacks. Other candidates include hash-based signatures and multivariate polynomial cryptography. These methods aim to provide the same level of security as classical systems while being resistant to quantum threats.
Additionally, as quantum cryptography becomes more mainstream, the industry will likely innovate more advanced algorithms utilizing quantum mechanics principles such as entanglement. This could lead to more secure key distribution methods that are inherently immune to interception.
The evolution of cryptographic algorithms will be crucial in establishing a secure future in quantum environments.
As quantum mechanics continues to influence our world, understanding these trends becomes essential for researchers and professionals alike.
Case Studies
Understanding real-world applications and implementations of quantum cryptography is crucial for grasping the implications of this emerging technology. Case studies provide tangible examples of how quantum cryptography is applied in practice, showcasing both its potential benefits and the challenges encountered in real-world settings. This section aims to elucidate specific scenarios where quantum cryptography has been employed, highlighting its impact on security and communication protocols.
Real-World Applications of Quantum Cryptography
Quantum cryptography has begun to carve out its niche in various sectors, demonstrating its effectiveness in enhancing security protocols. One of the most notable applications is in the realm of secure communications between financial institutions. For instance, the use of quantum key distribution (QKD) has been implemented in certain banks to protect sensitive data transfers. With QKD, banks can generate and exchange cryptographic keys in a manner that detects any potential eavesdropping attempts.
Another compelling application can be found in government and military communications. For instance, countries like China have started deploying quantum networks to secure communication channels likely targeted by hackers. By utilizing quantum teleportation, these networks ensure that any interception attempt results in an immediate alert, thus maintaining the integrity and confidentiality of sensitive information.
Some tech companies are also conducting experiments with practical implementations of quantum cryptography. For example, researchers at IBM have demonstrated prototypes in controlled environments. Their projects test the effectiveness of quantum moments in securing cloud-based services. The findings from these experiments could influence future commercial products, promoting the viability of quantum cryptography in mainstream usage.
Analysis of Quantum Security Implementations
Examining the implementation of quantum cryptography reveals both its robust strengths and inherent weaknesses. The importance of testing and refining these systems cannot be overstated. Not all quantum networking technologies are created equal. For instance, some implementations focus on urban applications, while others aim for long-distance communications. Each of these scenarios presents distinct challenges in terms of infrastructure, reliability, and scalability.
In assessing quantum security implementations, it is crucial to consider the infrastructure requirements. Quantum networks necessitate specialized equipment, such as photon detectors and quantum repeaters, which can be complex and costly. Moreover, environmental factors can impact the transmission quality of quantum states. Maintaining the fidelity of qubits in less-than-ideal conditions poses a significant technical challenge.
Ultimately, the lessons learned from these case studies contribute significantly to refining quantum cryptography techniques. They help identify practical barriers and potential solutions, guiding future research and development. Furthermore, as awareness grows surrounding the potential of quantum cryptography, it will push more industries to invest in secure quantum communications, blending theoretical advancements into actual practice.
Culmination
In the context of this article, the conclusion serves as a crucial element to emphasize the intricate interplay between quantum computing and cryptography. As technology evolves, understanding these complexities is vital for maintaining robust security measures. The profound implications of quantum computing on cryptographic practices raise both opportunities and hurdles that must be navigated with care.
Summarizing Key Insights
The exploration of quantum computing's impact on cryptography reveals several key insights:
- Quantum Threats: The emergence of algorithms such as Shor's and Grover's pose significant risks to traditional cryptographic systems, necessitating urgent reconsideration of current security protocols.
- Quantum Cryptography: Innovative solutions like Quantum Key Distribution (QKD) offer a promising avenue for secure communication in a quantum future, mitigating vulnerabilities found in classical systems.
- Future Directions: As quantum technologies progress, new cryptographic methods will likely need to be developed to safeguard data integrity and confidentiality.
These insights underscore the importance of continuously evolving security measures to counter the risks introduced by quantum advancement.
The Future of Encryption in a Quantum World
Looking ahead, the future of encryption is bound to change dramatically due to quantum computing. As quantum machines become more capable, it will be necessary to rethink and redesign encryption methods:
- Post-Quantum Cryptography: Research into cryptographic algorithms that can withstand quantum attacks will become paramount. These new algorithms must prioritize security against potential threats posed by quantum computers.
- Integration of Quantum Technologies: Future systems will likely integrate quantum principles directly into encryption processes, enhancing security while fostering new forms of secure communication.
- Educational Initiatives: As understanding of quantum technologies grows, educational and research institutions will need to prioritize training and resources to prepare the next generation of professionals in the field.