In-Depth Look at Perpetual Energy Generators
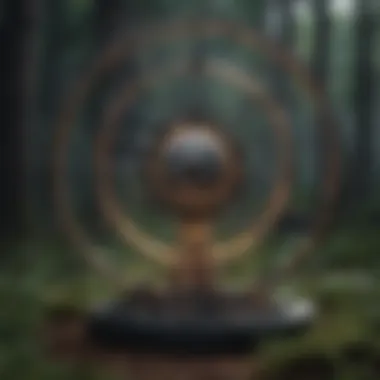
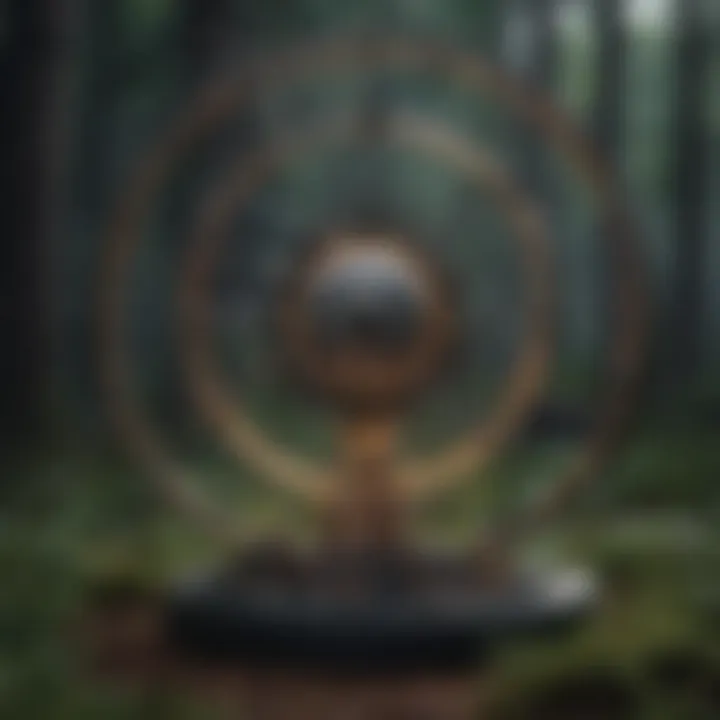
Intro
In the realm of energy generation, the concept of perpetual energy generators has sparked significant intrigue and debate. These systems, which aim to produce energy indefinitely without an external supply, challenge our current understanding of physics and energy conservation. Many have sought to explore these intriguing machines, pondering if they could hold the key to a sustainable energy future or if they reside solely in the realm of theoretical speculation.
This discussion hinges on understanding both the principles governing energy generation and the persistent pitfalls faced by innovators in the field. As researchers and scientists grapple with these questions, we delve into the foundational theories and expectations surrounding perpetual energy. Armed with insights from historical attempts and current breakthroughs, this exploration seeks to illuminate the path forward for renewable energy initiatives.
Preamble to Perpetual Energy Generators
Exploring the concept of perpetual energy generators offers a window into an area mingling audacity with the boundaries of current scientific understanding. This exploration is not merely for the curious mind, but a significant dive into insights that may reshape energy generation, sustainability, and our approach to innovation. Thus, it’s vital to grasp why this topic blooms with relevance.
One of the most notable advantages of investigating perpetual energy is the potential to revolutionize the energy landscape. With the continual rise of energy consumption and environmental degradation, a successful perpetual energy generator could mitigate these issues significantly.
Specific elements worth noting include:
- Sustainability: The allure of harnessing a consistent energy source that operates without depleting natural resources is compelling. It speaks directly to the global need for eco-friendliness.
- Technological Trends: Recent strides in related technologies spark debates and innovations in energy efficiency and clean energy solutions, setting the stage for further research.
- Challenging Conventional Wisdom: By breaking free from standard scientific constraints, the pursuit of perpetual energy systems pushes researchers to reconsider the very definitions of energy and motion, prompting fresh insights into classical physics.
However, it’s not all sunshine and rainbows; a myriad of considerations must be acknowledged. The major caveat lies in the longstanding laws of physics that seem to oppose the very concept of a perpetual motion machine. The complexities surrounding energy conservation call into question how these systems could theoretically operate without external energy loss.
Energy generation is at the heart of modern civilization, and reaching for an ever-elusive self-sustaining system nonetheless nurtures the innovation seed that humanity lives by. As we unravel the intricacies of these machines, we engage in a deeper conversation about our technological future and the ethos surrounding our stewardship of Earth.
"While the path is riddled with technical and philosophical hurdles, the pursuit of perpetual energy machines enlivens the conversation around what sustainable energy can mean for us in the coming years."
This introduction lays the groundwork for diving further into the essential definitions and historical backgrounds, turning the spotlight on the intricate dance between aspiration and practicality that defines our ongoing journey with perpetual energy generators.
Fundamental Principles of Energy Generation
Understanding the fundamental principles of energy generation is crucial to grasp the intricacies of perpetual energy generators. Energy generation isn’t just about generating electricity or power; it's deeply tied to the laws of nature that govern how energy can be produced, transformed, and utilized. The implications of these principles not only influence technological development but also touch upon environmental issues, sustainability, and the future of energy policies around the globe.
When discussing energy generation, one cannot ignore the role of efficiency, sustainability, and the conservation of energy. These concepts lay the groundwork for innovative designs, offering a lens to evaluate the feasibility of perpetual motion systems. Let's take a closer look at the foundational aspects that shape our understanding of energy generation.
Laws of Thermodynamics
The laws of thermodynamics form the backbone of all scientific inquiry into energy generation. These fundamental laws dictate not just how energy can be produced, but also how it behaves in various systems. The first law, often referred to as the law of energy conservation, states that energy cannot be created or destroyed, only transformed from one form to another. This principle is why perpetual motion machines, which claim to produce more energy than they consume, fundamentally contradict established scientific principles and become a source of fascination and debate.
The second law takes this further by introducing the concept of entropy. In any energy transfer or transformation, some energy will invariably be lost as waste heat, making it impossible to create a perfectly efficient machine. In simpler terms, even the most advanced technology will always experience energy loss. This is where the challenge lies—how can we develop systems that come as close to this ideal as possible, while recognizing that complete efficiency is unattainable?
"The laws of thermodynamics fundamentally shift our perspective on energy: rather than seeking to create energy from nothing, we must innovate ways to utilize existing resources more effectively."
Energy Conservation and Its Implications
Energy conservation is a principle that transcends mere scientific theory and underpins significant social and economic implications. In the context of perpetual energy generators, this principle challenges researchers to rethink the methodologies through which we generate and consume energy. The focus shouldn’t just be on producing more, but also on ensuring what we do create works efficiently and sustainably.
This conservation leads to several important considerations:
- Resource Management: Efficient use of available resources is paramount. Innovations in energy generation must consider not only their efficiency but also the environmental impact of sourcing materials needed for production.
- Policy Development: Policymakers must understand the dynamics of energy conservation. Regulations should encourage technologies that not only work towards energy generation but also aim for sustainability and minimal waste.
- Public Awareness: Educating the public about energy conservation empowers them to make informed choices. It fosters a culture of responsibility towards energy consumption, urging individuals and businesses alike to seek out and support sustainable practices.
In summary, the principles of energy generation offer a framework upon which perpetual energy generators must be built. By adhering to the laws of thermodynamics and respecting the concept of energy conservation, researchers and practitioners can open new avenues for exploration while working within the confines of scientific integrity. Understanding these aspects positions us better to envisage a future where energy generation does not just serve the immediate needs but aligns with the long-term goals of sustainability and resource management.
The Science Behind Perpetual Motion Machines
The concept of perpetual motion machines stirs curiosity within the realms of physics and engineering alike. These machines challenge the fundamental laws of nature, particularly the laws of thermodynamics. Understanding the science behind them is essential, as it helps illuminate why such inventions, while tantalizing, have remained largely theoretical. Comprehending these concepts not only furthers knowledge about energy conservation but also serves as a springboard for innovative thinking in energy generation technologies.
Types of Perpetual Motion Machines
First Kind
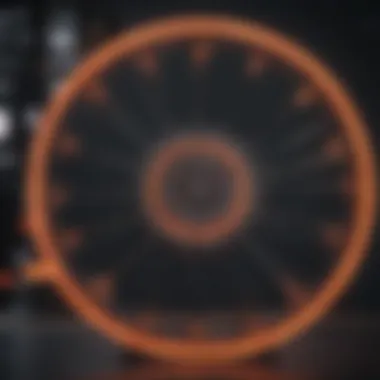
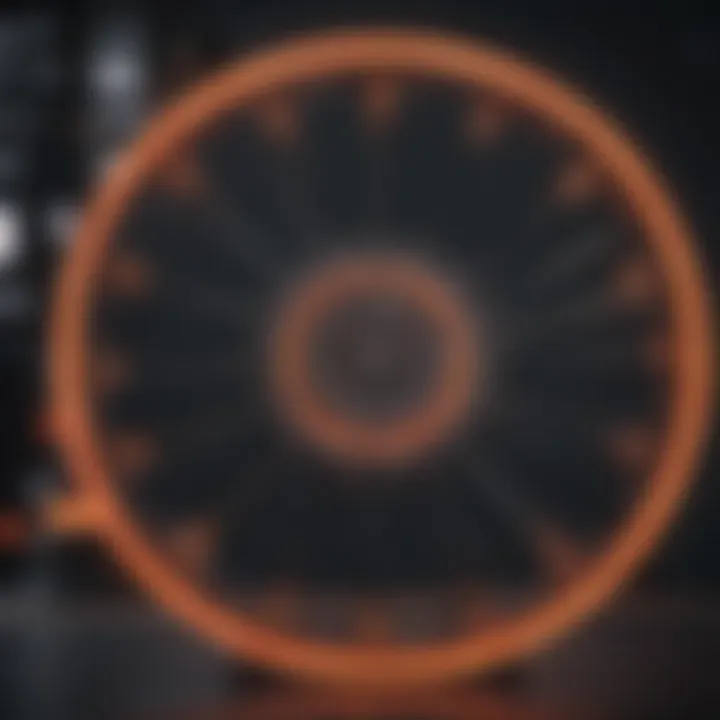
The First Kind of perpetual motion machines is characterized by their ability to produce work without an energy source. These machines are supposed to operate indefinitely without any energy input, violating the first law of thermodynamics, which states that energy cannot be created or destroyed. A classic example often discussed in this context is a wheel that spins forever once set in motion.
A noteworthy characteristic of First Kind machines is their theoretical simplicity. Many inventors have proposed designs using basic mechanical principles, thinking they could solve the energy crisis. However, despite their attractiveness, these machines inevitably succumb to friction and air resistance. The unique feature here lies in the allure of an effortless, free-flowing energy source that promises a utopian future.
Yet, the lack of feasibility brings several disadvantages. They have become somewhat of a scientific parlor trick, drawing attention more for their impossibility than practicality.
Second Kind
The Second Kind of perpetual motion machines aims to spontaneously convert thermal energy into mechanical work without any energy loss. These designs often involve heat sources and coolificators that try to maintain a temperature differential, supposedly getting work from this cycle indefinitely.
The key characteristic of Second Kind machines is their reliance on heat differentials. Theoretically, if one could create or maintain an environment with constant temperature differences, perpetual work could emerge. Such ideas have sparked interest in thermoelectric generators and their potential applications.
However, the major disadvantage lies in the second law of thermodynamics. Entropy increases in all natural processes means that no machine can operate in such a way without losing some energy overall. Thus, while tantalizing, the practical application of this kind remains elusive.
Third Kind
The Third Kind of perpetual motion machines refers to designs that can operate indefinitely without any work being done on them, essentially reaching a state of perpetual motion without energy loss. These machines push into the boundaries of quantum mechanics, where some theorists posit possibilities for behavior that seems to defy Newtonian physics. The most noteworthy example is often a magnetic perpetual motion machine, which theorizes magnetic forces that allow motion without energy expenditure.
An important characteristic of Third Kind machines is their outside-the-box thinking. Unlike the previous types, they encourage exploration into unique energy sources, often leading down the rabbit hole of advanced fields like quantum mechanics and field theory. However, a significant disadvantage is the lack of replicable experiments. Most findings are confined to theoretical discussions with no tangible applications in the foreseeable future.
Ideal Conditions for Operation
For any perpetual motion machine, the ideal conditions for operation are crucial yet virtually impossible to achieve. Essentially, these machines would require a perfect vacuum to eliminate air resistance, a frictionless environment to avoid loss of energy, and an endless energy supply not subject to degradation.
In practical terms, even if components are well-crafted and controlled, real-world applications are constantly besieged by entropy and energy mismanagement.
In sum, the science behind perpetual motion machines presents an exciting blend of creativity and scientific principles, but also serves as a reminder of the limitations dictated by the laws of thermodynamics. Studying these machines not only challenges conventional understanding but may, in turn, inspire new approaches to sustainable energy generation.
Modern Approaches to Energy Generation
The landscape of energy generation is undergoing a significant transformation, largely due to modern advancements and innovative technologies. This section dives into modern approaches that are changing the way we think about energy generation, emphasizing their importance for a sustainable future. By evaluating new technologies and research trends, we open the door to drastic improvements in efficiency and environmental sustainability within energy systems.
Innovative Technologies
Magnetic Levitation
Magnetic levitation utilizes magnetic forces to lift and propel objects without physical contact. Its contribution to energy generation lies primarily in reducing friction—an inherent problem in conventional systems. The key characteristic of magnetic levitation is its ability to create a stable, frictionless environment, enhancing the efficiency of machinery, as there is minimal energy loss due to friction. This positioning makes it a favorable choice for transport systems, such as Maglev trains, which can achieve remarkable speeds with reduced energy expenditure.
One unique feature of magnetic levitation is its application in generating kinetic energy from moving magnets that can potentially create a perpetual cycle. However, a significant drawback is the high initial cost of implementation and the need for precise engineering. Thus, while it offers great potential, extensive research and development are required to make it widely applicable.
Electromagnetic Systems
Electromagnetic systems encompass a vast range of devices that use electric and magnetic fields to generate energy. Their contribution is significant, particularly in renewable energy technologies, where they facilitate the efficient harvesting of energy from natural sources such as wind or solar. The standout characteristic of electromagnetic systems is their adaptability; they can be engineered for a variety of applications, from small-scale devices like generators to large-scale power plants.
What makes electromagnetic systems popular in energy research is their proficiency in converting mechanical energy to electrical energy. They possess a unique feature of scalability, allowing them to be used in diverse setups, from household appliances to industrial machinery. However, challenges include electromagnetic interference and energy losses due to heat, which can hinder efficiency if not managed appropriately.
Quantum Energy Extraction
Quantum energy extraction leverages principles from quantum mechanics to harvest energy in innovative ways, particularly from the fluctuations in quantum fields. Its contribution to the current energy landscape is intriguing, as it promises potential breakthroughs in efficiency that traditional systems cannot achieve. The key characteristic of quantum energy extraction is its ability to exploit energy at a microscopic scale, which could revolutionize our approach to energy generation.
A distinctive feature of this technology lies in its capability to tap into the energy of seemingly empty space. While this presents remarkable opportunities, the field is in its nascent stages, and many practical applications are still theoretical. Concerns exist regarding the stability and predictability of quantum systems, which can pose significant challenges for widespread adoption.
Research Trends and Developments
The field of energy generation never stops evolving. Research trends are shifting towards integration between traditional and innovative approaches, exploring sustainability and exploring the intersection of fields like material science and engineering. With an increasing focus on environmental policies and reducing carbon footprints, the future of energy generation is moving toward more collaborative efforts across industries.
As we progress, continuous advancements will shape next-generation technologies. The merging of ideas, supported by interdisciplinary collaboration, can potentially deliver solutions that address the growing demands of energy while maintaining a keen eye on efficiency and ecological impact. This collaborative spirit fosters innovation that stands to redefine how energy is generated and consumed in the coming years.
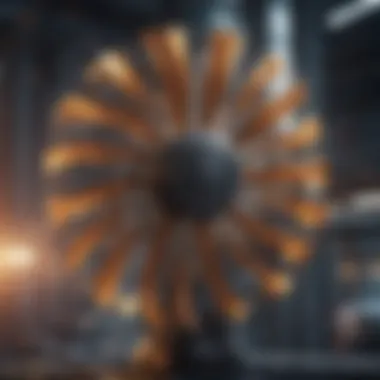
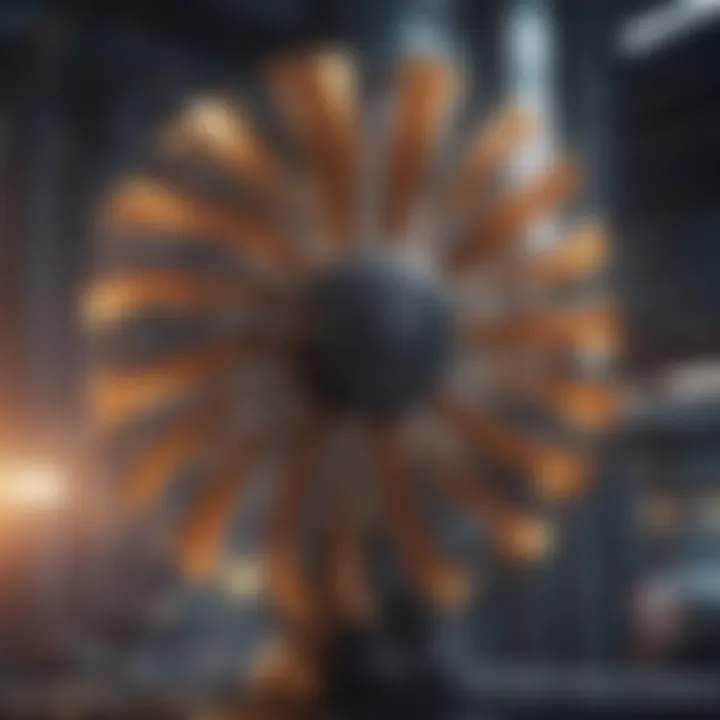
Challenges in Developing Perpetual Energy Generators
The quest for perpetual energy generators is not just a bold dream; it is a complex challenge that warrants a thorough exploration. As technology becomes more integral to our daily lives, understanding the obstacles in harnessing perpetual energy is vital. The significance of this topic resonates deeply within the realms of science and ethics. There are technical limitations, economic constraints, and societal implications at play, all contributing to the difficulty in realizing these systems.
Technical Limitations
When discussing perpetual energy, one must first grapple with the technical limitations that underpin any potential design. The laws of thermodynamics, which govern energy transfer and conservation, pose significant restrictions. Specifically, the first law states that energy cannot be created or destroyed, only transformed. This principle inherently contradicts the idea of a device that continuously outputs energy without an input—a cornerstone concept in perpetual motion machines.
Yet, it is not solely the foundational physics that dictates the feasibility of these generators. Engineering challenges also abound. Most designs require materials that can withstand immense strain over time without succumbing to degradation. For example, if we consider magnetic levitation systems, the technology must operate in environments free from air resistance and friction to maintain efficiency. Designing such a system as environmentally friendly and practical remains a tall order.
Some current approaches involve advanced materials and innovative designs, yet they often fall short under real-world conditions. It becomes a delicate balancing act, striving for efficiency while adhering to established physical laws.
Economic Viability
Beyond technical hurdles, economic viability is a looming concern for any budding perpetual energy generator project. Developing reliable technology often requires substantial investment in research and development, which can stretch budgets to their limits. The reality is, any investor or research body needs to see a clear path toward return on investment.
For instance, magnetic levitation systems may demonstrate potential, yet the initial costs for creating a prototype can be staggering. This factor raises the question: how can one manage costs while ensuring that the technology remains attractive in the market?
To shed light on this challenge, consider the contrast between renewable energy technologies—like solar and wind power—and the elusive perpetual energy generator. The latter often faces skepticism from traditional energy markets. Until perpetual generators can deliver tangible and scalable solutions, they might remain underfunded compared to more established renewable technologies.
Furthermore, policies and regulations surrounding energy generation impact the economic landscape. It would take a considerable shift in governmental support and public interest to drive funding towards these seemingly fanciful projects.
In summary, the development of perpetual energy generators is stymied by both technical limitations and economic realities. Breaking through these barriers requires innovative thinking and a commitment to redefining how we view energy generation.
Potential Applications and Impacts
The exploration of perpetual energy generators holds significance not just in theoretical discussions but also in real-world applications. This section aims to shed light on how these generators could reshape various industries and influence global energy policies, thereby impacting everyday life.
Industrial Use Cases
Perpetual energy generators can be a game-changer in numerous industries. In manufacturing, for instance, factories could harness these systems to power machinery without continual fuel input, reducing operational costs drastically. Here are a few potential applications:
- Renewable energy integration: Companies could integrate perpetual generators with solar and wind systems, enabling them to store energy for times when renewable sources are less available.
- Transportation: In the automotive sector, vehicles designed with these generators could operate efficiently without frequent refueling, thereby reducing dependency on fossil fuels and cutting costs.
- Telecommunication: Remote cell towers could utilize perpetual energy systems, allowing for consistent operation without the need to rely on local power grids or diesel generators in inaccessible areas.
These examples highlight how industries might leverage perpetual energy for increased efficiency and sustainability. However, while the prospect sounds appealing, there are also considerations, chiefly concerning the engineering and feasibility of such systems in diverse operational conditions.
Implications for Global Energy Policies
The emergence of perpetual energy generators could lead to critical shifts in global energy policies. Here are several facets worth considering:
- Energy independence: Nations may find themselves less reliant on imported fossil fuels, potentially democratizing access to energy and stabilizing national economies.
- Regulatory frameworks: Policymakers will need to navigate the challenges posed by new technologies, ensuring that regulations encourage innovation while safeguarding public interest.
"As we venture deeper into the realm of perpetual energy, the need for clear policies becomes paramount to harness its benefits effectively and ethically."
- Environmental considerations: With reduced emissions from traditional energy sources, countries could make strides toward their climate goals, fostering a more sustainable global environment.
- Social equity: Energy systems powered by perpetuity could level the playing field, making energy more accessible in developing regions, where traditional infrastructure may be lacking.
Culmination
Addressing the potential applications and impacts of perpetual energy generators isn't merely an academic exercise. It's a stepping stone toward envisioning a sustainable energy future. As industries adopt these technologies, the ripple effects on global energy policies could redefine our collective approach to energy consumption and dependency.
Ethical Considerations in Energy Research
The quest for innovations in energy generation, particularly within the realm of perpetual energy, is a nuanced journey that intertwines scientific ambition with profound ethical questions. As society strides towards solutions that promise sustainable energy, it is imperative to consider the ethical dimensions that underlie this pursuit. These considerations help shape a future where technological advancements don't compromise our moral compass or environmental integrity.
Environmental Impact
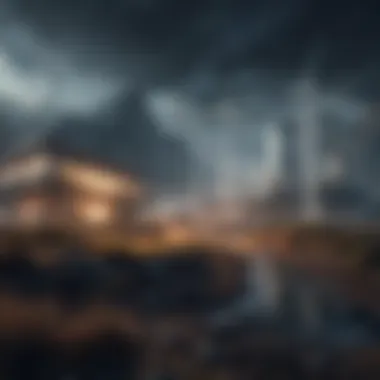
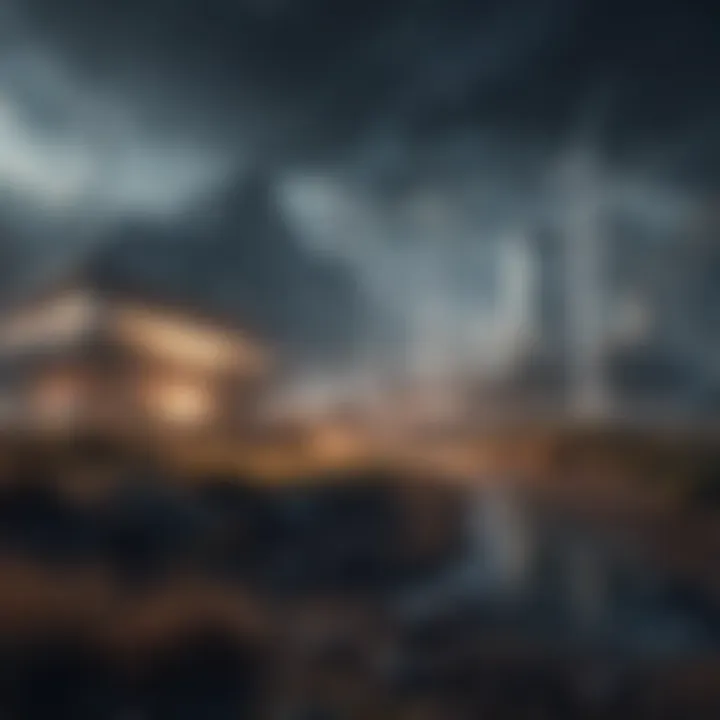
The notion of perpetual energy generation inherently raises the bar regarding environmental accountability. At the heart of this discussion is the fundamental responsibility to minimize any adverse effects on our planet. While these generators are often hailed as eco-friendly alternatives to traditional energy sources, one must probe deeper into their life-cycle assessments.
For instance, the materials used in constructing such generators can have varying ecological footprints. Extracting rare minerals for magnet technology or other components poses significant challenges. Destruction of ecosystems, along with the potential for pollution -- such as from the manufacturing processes -- necessitates a careful evaluation.
Additionally, the disposal of obsolete technology must be addressed. Waste management approaches for components that do not contribute to ecological harm should be a priority in every research agenda. To quote a prominent environmentalist, "We do not inherit the earth from our ancestors, we borrow it from our children." This perspective reinforces the weighty responsibility that falls on researchers and developers of energy technologies.
Sustainability and Resource Management
Sustainability is about more than just developing new technologies; it encompasses the holistic stewardship of resources throughout the entire lifespan of a product. In the context of perpetual energy generators, this means integrating sustainable practices from inception to end-of-life management.
To achieve this, interdisciplinary collaboration is crucial. Researchers in engineering, environmental science, and ethical studies must coalesce to devise best practices for material utilization, energy efficiency, and long-term impacts. Here are a few focal points to consider:
- Resource Optimization: Striving for maximum efficiency while using minimal resources is essential. This may involve recycling materials from outdated systems for use in newer technologies.
- Long-term Viability: Creating systems that are designed for longevity impacts not just resource consumption but also economic sustainability. Short-lived technologies that require frequent replacements can contribute to waste and resource depletion.
- Holistic Scale Evaluations: Assessing not only the direct ecological impact but also indirect consequences, such as societal impacts from the displacement of traditional energy industries, forms an irreplaceable part of responsible research.
Every energy research initiative must prioritize ethical considerations with diligence. While the allure of groundbreaking advancements may captivate, it is the integration of ethics that ensures progress remains aligned with our collective responsibility to both humanity and the environment.
Future Directions in Energy Generation Research
The search for innovative solutions in energy generation is ever-evolving, and the study of perpetual energy generators plays a pivotal role in this context. As global energy demands soar, it is essential to explore unconventional methods that might offer sustainable alternatives. This section investigates the promising future pathways in energy generation, highlighting emerging theories, new technologies, and the critical role of interdisciplinary collaboration.
Emerging Theories and Innovations
The field of energy generation is on the cusp of groundbreaking advancements that blend creativity with scientific inquiry. New theories are emerging that could reshape how we understand and harness energy. For example, concepts around zero-point energy, which suggests that energy exists in a vacuum at very small scales, are gaining traction. Researchers are exploring how this energy could be harnessed, potentially providing a near-limitless source for future generations.
Some innovations worth noting include:
- Quantum Energy Harvesting: Using principles of quantum mechanics to develop devices that can extract energy from the surrounding environment.
- Magnetic Flux Engines: This approach leverages magnetic fields to create motion, offering a feasible way to generate energy with minimal waste.
- Self-Powered Systems: Devices that can operate independently, utilizing ambient energy sources like temperature differentials or vibrations to sustain themselves.
These advances not only challenge traditional notions of energy generation but also promise improvements in efficiency and sustainability.
Interdisciplinary Collaboration
The integration of diverse fields of study is crucial for addressing the complexities of energy research. Collaboration among physicists, engineers, environmental scientists, and even behaviorists can yield richer insights and promote innovative solutions. This holistic approach encourages the blending of expertise, enhancing problem-solving capabilities.
Some notable benefits include:
- Cross-Pollination of Ideas: When experts from varying disciplines work together, they can challenge each other’s assumptions and inspire new lines of thought.
- Holistic Solutions: Combining knowledge from fields like ecology and sociology with engineering helps ensure that energy solutions are viable and socially accepted.
- Increased Funding Opportunities: Collaborative projects often attract more attention and resources from funding bodies, as they address multifaceted challenges in energy generation.
"The greater the variety of perspectives, the more comprehensive the potential solutions can become. The future of energy generation hinges on our ability to think outside the box."
The future of energy generation undoubtedly lies in embracing new theoretical approaches and fostering collaborative efforts across disciplines. This exploration is not just about technological advancement; it’s about reshaping our understanding of energy itself and paving the way for a sustainable future.
The End
In closing, the topic of perpetual energy generators holds profound significance in today's energy discourse. As we stand on the brink of various energy crises, exploring sustainable alternatives has never been more critical. These systems, which challenge our conventional understanding of energy generation, encourage us to rethink how we harness power, a necessity that grows with each passing day. The concept is steeped in both ambition and controversy, spurring debates in scientific, economic, and ethical arenas.
Recap of Key Points
Reflecting on the key ideas presented in this article:
- Definition and Historical Context: We clarified what perpetual energy means and traced its roots back through history, emphasizing the longstanding fascination and failures surrounding this pursuit.
- Scientific Principles: We examined the fundamental principles underpinning energy generation and delved into the laws of thermodynamics, highlighting their constraints on perpetual systems.
- Current Innovations: Our discussion on modern technologies showcased advancements such as magnetic levitation and quantum energy extraction, highlighting promising areas of research that may revolutionize energy generation.
- Challenges: Key obstacles like technical limitations and economic viability were outlined, underscoring why producing these systems in real-world scenarios remains elusive.
- Environmental Concerns: Ethical considerations were explored, focusing on the environmental implications and the necessity for sustainable practices.
- Future Directions: We looked forward to emerging theories and innovations that may pave the way for breakthroughs in energy solutions.
These elements weave together an intricate picture of where we stand and where we might go.
The Future of Energy Generation
Looking ahead, the future of energy generation is rife with possibilities yet unsettled by uncertainties. As technologies evolve, it becomes increasingly essential to blend scientific inquiry with pragmatism. The interdisciplinary collaboration of engineers, scientists, and policymakers will be crucial in addressing the multifaceted challenges that lie ahead.
Advancements in energy storage technologies could complement perpetual energy systems, providing stability and reliability to fluctuating renewable sources. Since the climate crisis looms ever larger, championing robust research into new avenues of energy generation can not only mitigate adverse environmental effects but also foster economic growth and job creation.
Moreover, the rise of global initiatives aimed at renewable energy adoption signifies a pathway toward practical implementations. Many countries are investing heavily in renewable technologies, demonstrating a recognition that sustainable energy isn't just an ideal—it's a necessity. As the global community shifts its focus to sustainable practices, the inquiry into perpetual energy systems may serve as a beacon of exploration.
In essence, while perpetual energy generators present enigmatic challenges, they also ignite the spirit of scientific advancement and innovation. Our collective future hinges on our ability to harness knowledge and creativity, pushing the envelope to develop systems that can truly change the energy landscape.