Mastering Quantum Physics: An In-Depth Exploration
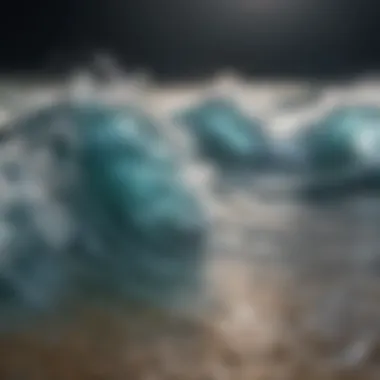
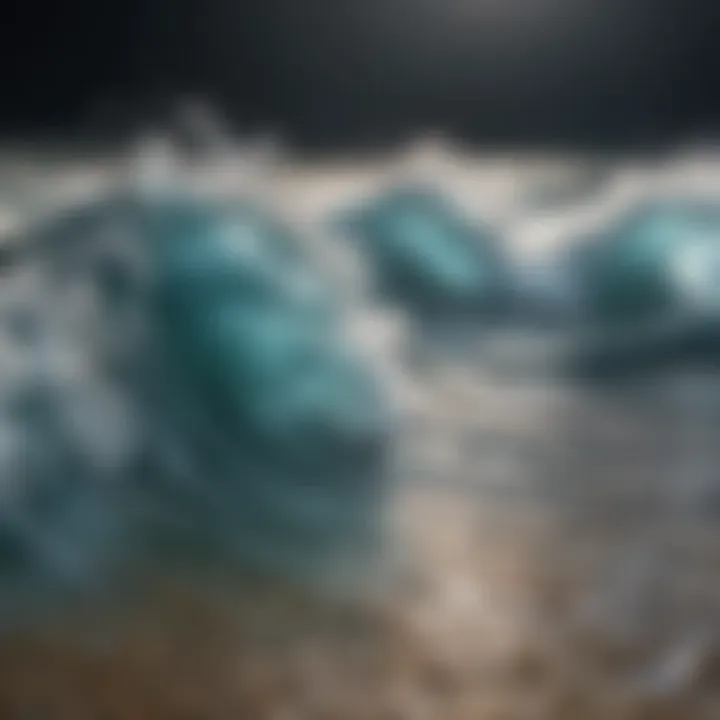
Intro
Quantum physics, a field often shrouded in mystery, provides the foundation for understanding the very fabric of our universe. It takes us through twisting paths of particles and waves, where the rules of classical physics seem to break down and new phenomena emerge. This guide is crafted as a stepping stone for those keen on unraveling the complexities of this fascinating discipline. Whether you're a student just dipping your toes into the subject or a seasoned educator seeking deeper insights, there’s plenty to uncover here.
You'll find discussions about pivotal concepts like superposition and entanglement, alongside a historical walk-through that traces the evolution of quantum theories. By delving into key experiments, we hope to illuminate the principles at play and showcase how these ideas are not just theoretical musings but have real-world applications reshaping technology and industry.
As we navigate through the intricate terrain of quantum physics, we'll also touch on emerging trends that point toward the future of research in this domain. Expect to come across graphs, charts, and other aids that will help break down some of those tough concepts into more digestible bites. So, buckle up for a journey that promises to be engaging, enlightening, and rich with insights.
"Quantum physics isn't just a branch of physics; it's a doorway into understanding our universe on a much deeper level."
Stay tuned for a closer look at the Key Research Findings, where we’ll dive into recent discoveries that are reshaping our comprehension of this remarkable field.
Preamble to Quantum Physics
Understanding quantum physics is like opening a treasure chest filled with mysteries that define the very foundation of our universe. This field of study transcends mere scientific interest; it forms the backbone of modern technology and influences various aspects of our daily lives. To appreciate the marvels of quantum mechanics, it's essential to grasp its significance, historical development, and applications.
The Importance of Quantum Mechanics
Quantum mechanics is more than just a collection of theories; it's a fascinating glimpse into the workings of the universe at its most fundamental level. Here’s why it matters:
- Foundation of Modern Physics: Quantum mechanics underpins most of today's physics. The principles established here have paved the way for notable theories such as quantum field theory and the standard model of particle physics.
- Technological Advancements: We wouldn't have the likes of lasers, semiconductors, and MRI machines without quantum mechanics. Its applications revolutionize industries like computing and medicine, showcasing how theoretical ideas can manifest in concrete technologies.
- Philosophical Questions: Quantum mechanics invites us to ponder profound philosophical questions regarding the nature of reality, observation, and existence itself. It challenges our classical notions and compels us to rethink what we know.
These aspects become increasingly relevant as our world becomes more interconnected with quantum technologies.
Historical Context
The journey of quantum physics is historic, marked by crucial milestones that reflect society's evolving understanding of nature. It is essential to have a backdrop of these events:
- Late 19th Century: Scientists like Max Planck laid the groundwork, proposing that energy is quantized and introducing the concept of quanta.
- Early 20th Century: Albert Einstein's explanation of the photoelectric effect in 1905 metaphorically slapped classical physics in the face, suggesting that light has both wave and particle properties.
- 1920s and 1930s: The works of Niels Bohr, Werner Heisenberg, and Erwin Schrödinger led to developments in theories like the wave equation and uncertainty principle. These breakthroughs enriched our understanding and laid the foundations for quantum theory as we know it today.
Studying this historical progression not only contextualizes quantum physics but also reveals the scientific community’s journey from uncertainty to clarity. It’s intriguing to see how many brilliant minds contributed to building this complex yet fascinating tapestry of knowledge.
In grasping these elements, learners can appreciate quantum physics as not just a subject brimming with equations but as a crucial story of our quest for understanding the cosmos.
Fundamental Concepts
Understanding fundamental concepts in quantum physics forms the groundwork for further exploration into this fascinating discipline. It encompasses the basic principles that describe how microscopic particles behave differently from our everyday experiences. This foundation not only helps in grasping complex theories but also sheds light on the real-world applications that stem from quantum mechanics. The significance of these concepts lies in their ability to fundamentally alter perspectives on reality itself, making the seemingly impossible a tangible field of study.
Wave-Particle Duality
Wave-particle duality is a cornerstone of quantum physics, indicating that particles such as electrons and photons exhibit both wave-like and particle-like properties. This means, for instance, that light can behave like a wave, spreading out and creating interference patterns, or like a particle, being counted in discrete packets called photons. This duality was notably showcased in the double-slit experiment, where light passing through two slits created an interference pattern when not observed, yet appeared to act as particles when measured.
Understanding this dual nature is crucial, as it challenges classical physics notions. No longer can we simply categorize matter as either a particle or a wave; they can be both at the same time. This opens up a new realm of possibilities in physics and technology, including modern advancements in photonics and telecommunications.
Quantum Superposition
Quantum superposition expands upon the idea of wave-particle duality, asserting that quantum systems can exist in multiple states at once until they are observed or measured. Imagine flipping a coin—prior to looking at it, it can be thought of as both heads and tails at the same time. However, once you check it, it collapses into one of the two outcomes. In quantum literature, famous thought experiments like Schrödinger's cat illustrate this phenomenon, positing that a cat inside a box can be simultaneously alive and dead until the box is opened.
This principle plays a role in the development of quantum technologies, such as quantum computing. Here, qubits allow for much greater processing capabilities than classical bits due to their ability to exist in multiple states of 0 and 1. Superposition leads to parallelism in computations, enabling vastly more powerful performance in certain tasks.
Quantum Entanglement
Quantum entanglement is perhaps the most intriguing aspect of quantum mechanics. Here, pairs or groups of particles become linked in such a way that the state of one particle instantaneously affects the state of the other, regardless of the distance separating them. This seemingly defies logic and the conventional understanding of information transfer and locality. Einstein famously referred to this phenomenon as "spooky action at a distance," illustrating the discomfort even some foundational physicists felt about the implications of entanglement.
Entangled particles remain correlated even when separated light years apart, which raises profound questions about the nature of reality and our understanding of the universe. Moreover, entanglement is harnessed in fields like quantum cryptography, where it ensures the security of information transmission, and quantum teleportation, paving the way for advancements that could revolutionize communications.
"Entanglement demonstrates that quantum particles can be intricately linked, with their states interdependent, no matter the distance."
By understanding these fundamental concepts—wave-particle duality, quantum superposition, and quantum entanglement—we arm ourselves with the essential building blocks to explore more advanced topics in quantum physics. As you delve deeper into this compelling field, these concepts will continue to resonate, revealing the intricate nature of the world at its most fundamental level.
Mathematics of Quantum Mechanics
Mathematics serves as the backbone of quantum physics, a tool that bridges the gap between abstract concepts and tangible observations. Unlike classical physics, where equations can sometimes be intuitive, quantum mechanics demands a higher level of mathematical sophistication. This complexity stems mainly from dealing with probabilities and uncertainties at the atomic scale. Understanding these mathematical underpinnings is crucial for students, researchers, and anyone eager to grasp the field of quantum physics.
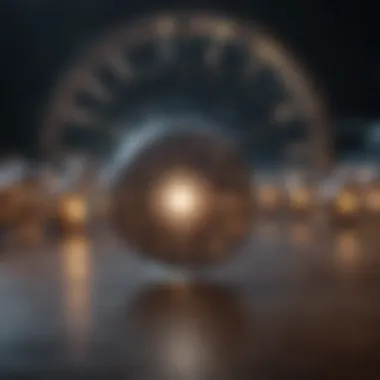
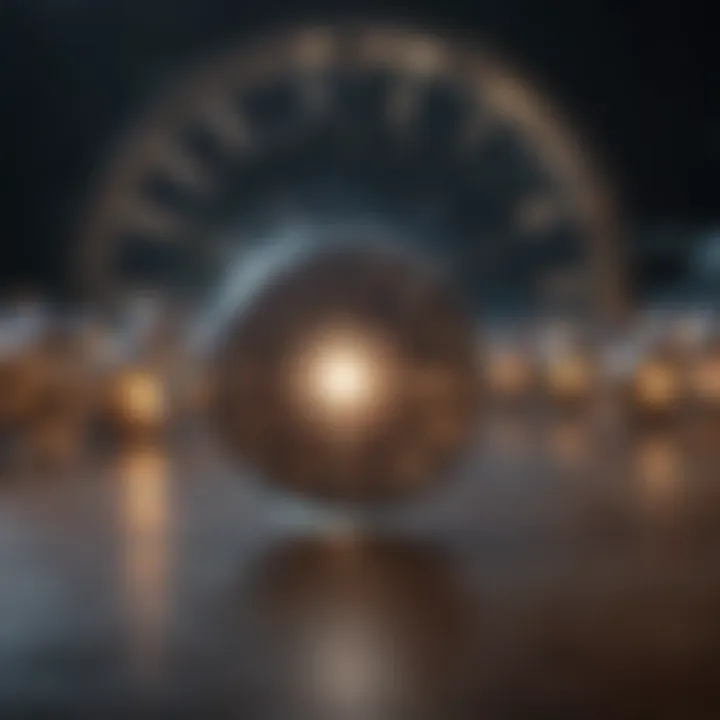
One of the primary benefits of understanding this mathematics is an enhanced capability to conceptualize phenomena that defy everyday experience. Mathematics not only articulates the rules of quantum theory but also offers frameworks for predicting outcomes in experiments — a key aspect that keeps this field at the forefront of modern research.
The inclusion of advanced topics such as linear algebra, probability theory, and operator calculus ensures a thorough engagement with quantum mechanics. By diving into these areas, one acquires tools essential for modeling quantum systems effectively and predicting their behaviors. Calculating probabilities, for instance, becomes less an exercise in guesswork and more a disciplined application of numerical methods.
Linear Algebra in Quantum Physics
Linear algebra is, without a doubt, the cornerstone of quantum mechanics. Every quantum state can be represented as a vector in a complex vector space, known as Hilbert space. Thus, concepts from linear algebra, such as vectors, matrices, and linear transformations, facilitate an understanding of state transformations and measurements. When considering quantum states, the linearity of quantum mechanics allows for the superposition of states, leading to phenomena that are impossible within classical frameworks.
Key Points of Linear Algebra in Quantum Physics:
- Vectors and States: Quantum states are often represented by vectors, allowing for the manipulation and transformation within abstract spaces.
- Matrices and Operators: Observables, such as position and momentum, are represented by operators, which correspond to matrices acting on quantum state vectors.
- Eigenvalues and Measurements: Measuring a quantum system’s observable relates to finding the eigenvalues of the corresponding operator, encapsulating the possible outcomes of an experiment.
Probability and Quantum States
Probability plays an indispensable role in quantum mechanics, primarily due to the inherent uncertainties associated with measuring quantum systems. Unlike classical mechanics, where predictability is paramount, quantum mechanics is inherently probabilistic. The probabilities of different outcomes can be mathematically described using the squared magnitudes of the wave function's components, resulting in the Born rule, a fundamental concept in quantum physics.
This probabilistic nature introduces unique challenges and considerations. For example, when analyzing a system, one doesn't just calculate a single path or outcome; instead, one must consider a whole range of possibilities. This necessity leads to Quantum state vectors and their associated probabilities defining a richer, more complex view of reality, where particles can exist in multiple states simultaneously until an observation is made.
Operators and Observables
In quantum mechanics, operators are essential constructs that help describe physical observables. These include various measurable quantities, such as energy, momentum, and position. Using mathematical operators, physicists can compute expected values and uncertainties of these observables, facilitating a deeper understanding of quantum behavior.
Every operator corresponds to a specific observable. For instance, the momentum operator acts on a wave function to yield momentum values. Furthermore, the behavior of operators under various conditions leads to crucial insights, as the commutation relations can help elucidate fundamental features of quantum systems.
To sum it up, the study of mathematics within quantum mechanics is not merely academic. It is a critical component that makes predictions, advancements, and overall understanding possible in a field that challenges our most basic intuitions about the universe. As we forge ahead into complex realms like quantum gravity or quantum biology, these mathematical foundations will be essential in guiding research and discovery.
Key Experiments in Quantum Physics
Understanding quantum physics isn't just about diving into theoretical frameworks or abstract mathematics. It's about grasping how these principles unveil the intricacies of our universe through experiments. Key experiments have played a monumental role in both illuminating the core concepts of quantum mechanics and debunking long-standing assumptions about the nature of reality. Whether you are a student, educator, or researcher, being familiar with these experiments provides a substantial foundation for appreciating quantum phenomena. This section will dissect three pivotal experiments: the Double-Slit Experiment, Quantum Teleportation, and Bell's Theorem Tests. Each showcases a different facet of quantum mechanics, allowing us to see both its beauty and its perplexities.
Double-Slit Experiment
The Double-Slit Experiment is often hailed as the crown jewel of quantum mechanics. Conducted first by Thomas Young in 1801, it set the stage for a seismic shift in our understanding of light and matter. At its core, the experiment illustrates two fundamental principles: wave-particle duality and the role of observation in determining physical reality.
When particles like photons or electrons are directed towards a barrier with two closely spaced slits, one might expect them to behave like traditional projectiles, producing two distinct patterns corresponding to the slits. However, when unobserved, these particles display an interference pattern typical of waves. This phenomenon indicates that particles possess dual characteristics, functioning as both particles and waves.
When an observer attempts to measure which slit a particle goes through, the interference pattern vanishes. This raises pressing questions about the impact of observation in quantum systems and throws a wrench in our understanding of causality and reality.
"In the double-slit experiment, the very act of measuring affects the outcome, confounding classical intuition."
Thus, this experiment not only demonstrated the peculiar behaviors of quantum entities but also ignited debates about the foundational philosophy of science.
Quantum Teleportation
Quantum Teleportation might sound like a page ripped straight from science fiction; however, it is very much grounded in reality and shows a potential pathway for advancing quantum communication. First achieved in 1998, this experiment goes beyond merely transmitting information. Essentially, quantum teleportation involves the transfer of quantum states from one particle to another at a distance, without physically moving the particle itself.
The principle hinges on entanglement, a fundamental concept of quantum mechanics where particles become interlinked, such that the state of one particle is dependent on the state of another, regardless of the distance between them. During quantum teleportation, the sender destroys the original particle's state, while a newly prepared particle takes on that state instantaneously.
This phenomenon has promising implications for future technologies, particularly in the realms of secure communication and computing.
Bell's Theorem Tests
Bell's Theorem, articulated by physicist John Bell in 1964, fundamentally altered our understanding of quantum theory and the classical worldview. The theorem's experiments test the validity of local realism, which posits that particles are distinct entities with predetermined properties. Bell's experiments, often involving entangled particles, demonstrate that no local hidden variable theory could adequately describe the correlations observed in quantum entanglement.
Key experiments designed to test Bell’s inequalities have consistently validated quantum mechanics, showing that entangled particles can exhibit correlations stronger than classical physics would allow, defying any local causal explanation. These findings have not only confirmed the bizarre nature of quantum mechanics but also prompted philosophical questions about reality, determinism, and the interconnectedness of the universe.
End
Each experiment discussed here reveals layers of complexity that beckon both fascination and contemplation. The Double-Slit Experiment compels us to rethink observation's role in shaping reality, Quantum Teleportation pushes the boundaries of how we understand space and time, and Bell's Theorem Tests challenge the very fabric of deterministic views of nature. Together, they form a narrative that is both perplexing and enlightening, inviting us to delve deeper into the mystique of quantum mechanics.
Interpretations of Quantum Mechanics
Understanding the interpretations of quantum mechanics provides a deeper comprehension of the underlying principles guiding the behavior of particles at the quantum level. Each interpretation offers a lens through which to view phenomena that challenge classical intuitions about the nature of reality. These interpretations do not just exist in isolation; they help shape discussions on fundamental questions regarding the universe's existence, human perception, and the very lines of science and philosophy.
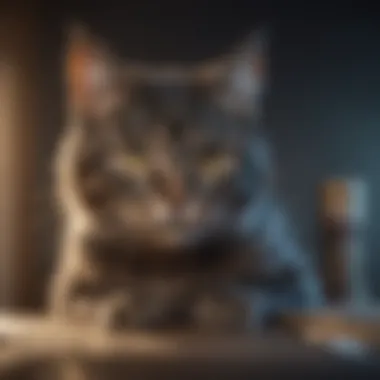
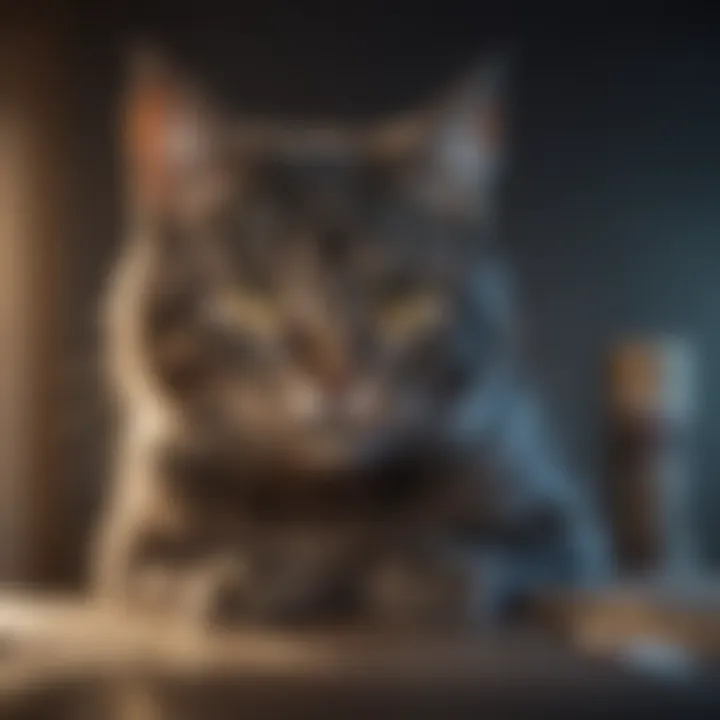
The nuanced perspectives of these interpretations can sway how researchers formulate their theories, ultimately leading to advancements in technology and a richer understanding of existence itself.
Copenhagen Interpretation
The Copenhagen interpretation stands as one of the most widely accepted interpretations of quantum mechanics, chiefly attributed to figures like Niels Bohr and Werner Heisenberg. This framework posits that quantum particles do not have definite states until they are measured. In simpler terms, it puts forward the idea that until we observe a quantum system, it exists in a superposition of all possible states.
One crucial aspect of the Copenhagen interpretation is the principle of complementarity. This states that different experiments can yield different results depending on how they are set up—much like how a rotating cube might look different when viewed from various angles. Thus, it emphasizes an important belief: the act of observation influences the system being observed. This intersection of measurement and reality raises philosophical questions about the observer's role in determining physical phenomena.
Benefits of the Copenhagen Interpretation:
- Provides a grounded approach to quantum mechanics that has practically guided physicists for decades.
- Bridges physics with philosophical inquiries about reality and knowledge.
Many-Worlds Interpretation
Contrasting sharply with the Copenhagen view, the Many-Worlds interpretation, proposed by Hugh Everett III, suggests that every quantum event spawns a multitude of parallel universes. Instead of collapsing to a single outcome upon measurement, all possibilities coexist in branching realities. Imagine flipping a coin; rather than simply landing heads or tails, the universe expands into two branches—one where the coin is heads and another where it is tails.
This interpretation opens fascinating discussions surrounding free will and determinism. The existence of multiple, simultaneous outcomes leads one to ponder: does every decision create a new branch of reality? While the Many-Worlds interpretation may seem like science fiction, it provides a robust framework to deal with quantum anomalies like entanglement without necessitating instantaneous communication across vast distances.
Considerations with Many-Worlds Interpretation:
- Challenges the classical notions of causality and singular outcomes.
- Raises questions about the nature of consciousness and whether beings in different universes perceive these divergent realities.
Pilot-Wave Theory
The Pilot-Wave theory, alternatively known as de Broglie-Bohm theory, offers another perspective. Unlike the Copenhagen interpretation, which wrestles with uncertainty and probability, Pilot-Wave theory asserts that particles possess definite trajectories guided by a wave function. The wave ostensibly serves as a pilot that governs the movement of particles, providing a deterministic framework despite the probabilistic nature of measurement in quantum mechanics.
A significant advantage of the Pilot-Wave theory is that it reinstates a classical sense of locality and determinism while accommodating quantum phenomena. For example, particles move under specific laws influenced by this guiding wave, allowing physicists to calculate precise outcomes without invoking randomness.
Key Points to Remember:
- The Pilot-Wave theory emphasizes a deterministic view, often appealing to those who prefer a more classical approach to understanding reality.
- This interpretation showcases quantum mechanics as compatible with a causally structured world, aligning with our everyday experiences.
"The interpretations of quantum mechanics don't merely explain the phenomena; they unveil the philosophical intricacies woven into the very fabric of existence."
Exploring these interpretations enlightens the discussions on the foundational aspects of quantum theory, and through this lens, we glimpse the philosophical implications they carry into broader scientific narratives.
Applications of Quantum Physics
The realm of quantum physics isn't just a theoretical playground; its applications are reshaping technology, security, and even healthcare. Understanding these applications provides a window into the profound impact quantum mechanics has on our daily lives and industries. Each advancement highlights the synergy between theory and practical uses, bridging the gap between abstract concepts and tangible solutions. The following sections delve into three significant applications: Quantum Computing, Quantum Cryptography, and Medical Imaging.
Quantum Computing
At the forefront of quantum physics applications is quantum computing. Unlike traditional computers, which use bits as the smallest unit of data, quantum computers employ qubits. These qubits can exist in multiple states simultaneously, thanks to principles like superposition. This characteristic allows quantum computers to process a vast amount of possibilities at once.
Imagine solving complex problems in seconds that would take classical computers millennia. From optimizing logistics to cracking cryptographic codes, the potential of quantum computing is extraordinary. Companies like IBM and Google are investing heavily in this technology. Their recent breakthroughs are not just theoretical, but they are getting closer to creating practical quantum computers suited for real-world problems.
"Quantum computing is set to revolutionize industries by solving problems that are currently impossible for classical computers."
Quantum Cryptography
Security in the digital age is crucial, and quantum cryptography emerges as a promising frontier. Utilizing principles of quantum mechanics, it offers unprecedented levels of security for information exchange. The most notable technique is Quantum Key Distribution (QKD), which enables two parties to create a shared, secret key. Any attempt to intercept this key alters its state, alerting users to potential breaches.
Consider the implications for banking and secure communications—where even the slightest breach can lead to catastrophic consequences. Companies specializing in cybersecurity are investigating quantum cryptography to safeguard transactions and maintain privacy in an increasingly digital world.
Medical Imaging and QI
In the healthcare domain, quantum principles are making waves, particularly in medical imaging techniques. Quantum Imaging (QI) uses quantum states of light to significantly enhance the resolution and sensitivity of imaging tools. This approach enables better detection of subtle changes in tissue and organs, providing clearer images than classical imaging methods.
For example, in oncology, improved imaging can lead to earlier detection of tumors, drastically affecting treatment outcomes. Additionally, research into Quantum-enhanced MRI technology is underway, promising to revolutionize diagnostics. By integrating quantum physics with medical imaging, healthcare providers stand to improve patient care and achieve unprecedented accuracy in diagnostics.
In summary, the applications of quantum physics are not merely theoretical musings; they are concrete innovations that promise to enhance our world in various fields. From computing to security and healthcare, the influence of quantum mechanics is increasingly evident, making it an essential area of study and exploration.
Philosophical Implications
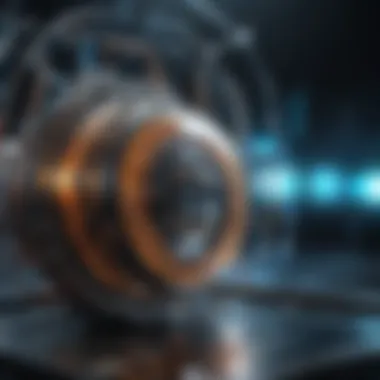
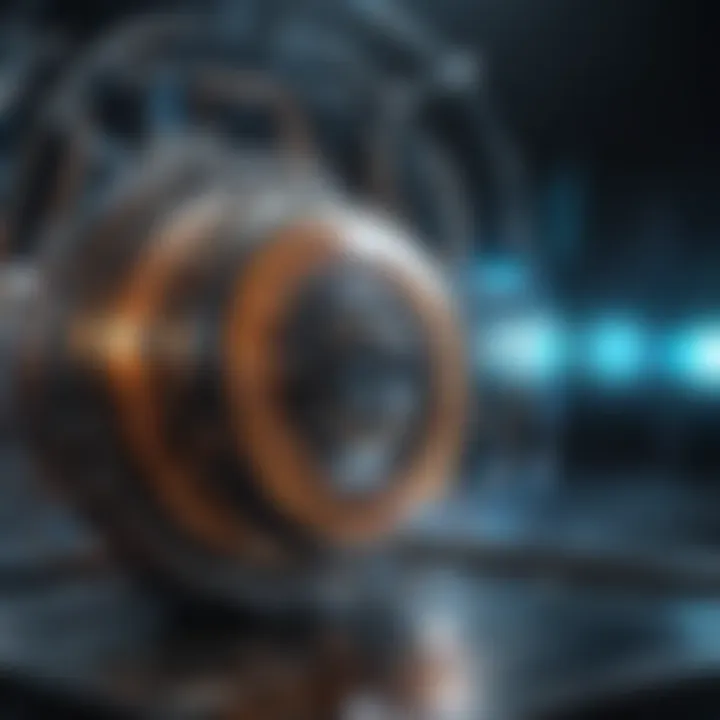
The realm of quantum physics does not just engage with the tangible; it reaches into the abstract. The philosophical implications of quantum mechanics are vast, influencing how we perceive reality, causation, and even our own existence. Understanding these implications can enrich our comprehension of the universe and ourselves, providing a deeper context for the scientific principles discussed throughout this article.
One of the key elements in this philosophical discourse is how quantum mechanics challenges classical notions of reality. Unlike everyday experiences where objects have well-defined states, quantum phenomena introduce uncertainty at a fundamental level. For instance, the behavior of particles at quantum scales cannot be precisely predicted, but rather described by probabilities. This shift raises questions about the deterministic universe that classical physics proposed. It encourages metaphysical discussions about what it means for something to exist if its state is not definitively known until it is observed.
Moreover, grappling with these issues forces us to reconsider the role of the observer in the quantum realm. The act of measurement, which seems straightforward, plays a critical role in determining the state of quantum systems. Are observers merely passive recorders of reality, or does their interaction fundamentally shape that reality? This questioning leads to profound philosophical considerations about knowledge and existence itself.
Additionally, the implications extend to our understanding of free will and determinism. The adaptability of quantum mechanics invites thoughts on whether our choices are predestined by physical laws or if a form of randomness plays a role. This intersection challenges not only scientists but also ethicists and philosophers to engage in discussions about moral responsibility in a potentially probabilistic universe.
"The more we learn about the quantum realm, the more we realize that reality is a complex tapestry woven with threads of probability, perception, and paradox."
In this light, analyzing quantum mechanics from a philosophical perspective is not merely an academic exercise; it reflects a kind of inquiry that can resonate with every individual. As we navigate through the intricacies of existence, understanding these philosophical implications offers enriching insights and opens avenues for transformative thinking.
The Nature of Reality
The nature of reality, through the lens of quantum physics, is profoundly intricate. At its core, quantum mechanics posits that reality is not merely what is observed, but also what is implied by the probabilities of various states. Consider the famous thought experiment of Schrödinger's cat, where a cat in a box can be both alive and dead until someone opens the box to observe it. This illustration speaks to how unobserved states exist in superposition, creating a narrative where reality is dynamically framed by observation itself.
This begs the question: If reality can be shaped by what we choose to observe, how do we define it? The uncertainties inherent in quantum probabilities suggest that there may not be a single objective reality, but rather many layers or dimensions of existence that interact according to the rules of quantum mechanics. While this notion is challenging, it invites a richer interpretation of our experiences and the world around us.
Free Will and Determinism
Delving into free will and determinism offers yet another layer of complexity. The random nature of quantum events can cast a shadow on deterministic views that have dominated classical physics. If at the fundamental level, events can happen without a deterministic cause, how does that influence our understanding of free will?
A few pertinent questions arise: Are our decisions truly our own, or are they swayed by probabilistic events outside our control? Furthermore, how do we reconcile moral accountability if some aspects of our decision-making can be attributed to randomness? In this light, quantum mechanics doesn't just challenge traditional views—it redefines the canvas of human agency and moral philosophy, pressuring us to consider a more nuanced spectrum of freedom and responsibility.
Modern Challenges and Research Frontiers
In the realm of quantum physics, there are hurdles that scientists face which not only shape current research but also define the trajectory of future discoveries. Understanding Modern Challenges and Research Frontiers helps to contextualize ongoing debates and innovations within the field. The concept of quantum gravity, for instance, represents a fascinating intersection of quantum theory and general relativity that has yet to be reconciled. By tackling these challenges, researchers aim to deepen our comprehension of the universe while paving the way for technological advancements.
Quantum Gravity
At the heart of the discussions surrounding quantum gravity lies the quest for a unified theory that merges the principles governing quantum mechanics with those that describe gravity. Traditional physics has treated these forces as separate entities, but the inconsistencies that arise when applying both theories to extreme conditions—like black holes or the Big Bang—signal the need for a new framework.
The challenge of quantum gravity isn't just academic. It bears profound implications for our understanding of space and time. Consider this: If one were to probe the very fabric of spacetime at a quantum level, one might discover various exotic phenomena—think about wormholes or even the multiverse theories that keep philosophers and physicists up at night.
Researchers are exploring theories such as loop quantum gravity and string theory as potential candidates to unite these realms. Yet, practical experiments that could validate or refute these theories are challenging to design. The scales involved, often at the Planck length, are far removed from what current technologies can measure.
Quantum Biology
An emerging field that often slides under the radar is quantum biology, which investigates how quantum phenomena affect biological processes. The idea that quantum mechanics could influence the workings of life might sound strange at first, but consider how plants perform photosynthesis. Some studies suggest that quantum coherence allows plants to capture sunlight more efficiently than any artificial system we have designed.
In addition to photosynthesis, quantum effects are thought to play a role in navigation for certain species, such as birds which use Earth’s magnetic fields. This area of research is less understood but ripe with potential discoveries that could revolutionize our knowledge of both biology and physics.
The potential challenges are exhilarating as well. How does quantum entanglement behave in the noisy environments of living cells? And what does it imply for the evolution of complex organisms? The inquiry into quantum biology has broader impacts, leading to developments in catalysis, medical imaging techniques, and even in the understanding of diseases at a quantum level.
"The quest to comprehend how quantum mechanics threads through the fabric of biology could redefine everything we thought we knew about life itself."
Moving forward, it is crucial for both experimental and theoretical physicists to engage with these challenges. With fresh ideas and interdisciplinary collaboration, we stand on the brink of groundbreaking discoveries that may finally illuminate the intertwined destinies of quantum mechanics and the phenomena of life.
Culmination
The conclusion of this extensive exploration into quantum physics serves as a crucial point for synthesizing the multitude of layers discussed throughout the article. By revisiting essential topics, we lay the groundwork for a more profound understanding of this intricate field and its implications on both a scientific and philosophical level.
Recapitulation of Key Themes
In this journey through quantum physics, several key themes emerged, shaping the reader's comprehension:
- Fundamental Concepts: We delved into the wave-particle duality, superposition, and entanglement, fundamental constructs that challenge classical views of nature.
- Mathematical Foundations: Understanding linear algebra's role, probability theories, and operators helped clarify how quantum mechanics operates on a mathematical plane.
- Pivotal Experiments: The exploration of experiments like the double-slit phenomenon and quantum teleportation showcased how empirical evidence substantiate theoretical underpinnings.
- Philosophical Debates: We also examined the philosophical implications of quantum mechanics, including questions about reality's nature and concepts surrounding free will.
- Modern Applications: Practical uses in sectors such as computing, cryptography, and medicine point to quantum physics' growing relevance in technology.
These themes provide a rich tapestry that interweaves historical context with modern implications, highlighting the dynamism inherent in quantum mechanics.
The Future of Quantum Physics
Looking forward, the future of quantum physics holds abundant promise and challenge. As new technologies emerge, the interplay between theory and experimentation becomes increasingly vital.
- Quantum Computing: Innovations in this domain could revolutionize how we process information. Companies like Google and IBM are actively pursuing breakthroughs, suggesting that quantum computers may outstrip traditional systems in complexity and power.
- Interdisciplinary Research: There is excitement around fields like quantum biology, where the principles of quantum physics are potentially influencing biological processes. Understanding these connections may usher in a new era of scientific integration.
- The Quest for Quantum Gravity: Addressing the incompatibility between quantum mechanics and general relativity is a grand pursuit. Solutions could unlock deeper insights into the universe’s workings.
As we venture into this emerging landscape, engaging with quantum physics remains imperative. The implications of continued research promise to alter our perception of reality and fidelity in technological advancements.
"The most beautiful experience we can have is the mysterious. It is the fundamental emotion which stands at the cradle of true art and true science." – Albert Einstein
In summary, the journey through quantum physics is not merely an academic exercise; it represents a pathway toward understanding the universe at its most foundational level. Embracing its complexities and remaining curious about its future can lead to remarkable discoveries in both science and philosophy.