Exploring the Large Hadron Collider: Key Insights
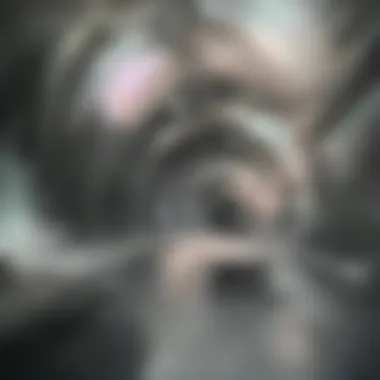
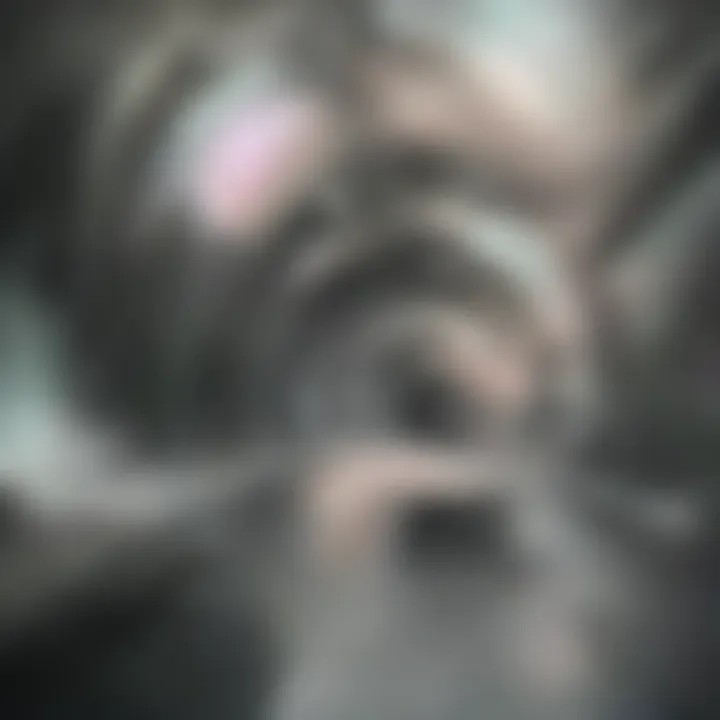
Intro
The Large Hadron Collider (LHC) stands as a monumental achievement in the field of physics and engineering. Situated at CERN, the European Organization for Nuclear Research, it is the most powerful particle accelerator in existence. The LHC was designed primarily to test fundamental theories in particle physics, particularly the Standard Model. This model describes the electromagnetic, weak, and strong nuclear interactions that govern particle physics.
Scientists use the LHC to explore both well-established theories and uncharted territories in physics. One of its key objectives is to search for the Higgs boson, a particle crucial to our understanding of mass. Furthermore, it seeks to unravel the enigmatic nature of dark matter, believed to account for much of the universe's mass, despite being undetectable by conventional methods.
With the LHCโs ability to collide protons at unprecedented energy levels, it has continually surprised the scientific world with its findings, providing fresh insights into the structure of matter and the universe.
Key Research Findings
Overview of Recent Discoveries
Since its inception, the LHC has been instrumental in several groundbreaking discoveries. One of the most notable was the discovery of the Higgs boson in 2012. This particle was predicted by the Standard Model and its detection confirmed theories that have been in place for decades. It affirmed the existence of the Higgs field, a mechanism that gives mass to elementary particles.
In subsequent years, the LHC has contributed to investigations into various phenomena, such as the properties of quark-gluon plasma, which is thought to be the state of matter just after the Big Bang. Additionally, it has intensified the search for supersymmetric particles, which would help explain dark matter.
Significance of Findings in the Field
The discoveries made at the LHC extend far beyond the confines of CERN. They have profound implications for astrophysics, cosmology, and our understanding of the universeโs evolution. The confirmation of the Higgs boson opened new avenues for research into high-energy particle physics and validated theories that were previously speculative. This not only enhanced our understanding but has also reinforced the importance of theoretical predictions in guiding empirical research.
The LHC's work on dark matter and other particles suggests that we may only be scratching the surface of understanding the universe's mysteries. As research continues, it is likely that new theories will emerge, challenging our current perspectives and expanding our knowledge of the cosmos.
Breakdown of Complex Concepts
Simplification of Advanced Theories
To make the research conducted at the LHC accessible to a wider audience, it is essential to break down complex theories into understandable terms. The concept of the Higgs field, for instance, can be summarized simply: it is a field that exists throughout the universe. Particles acquire mass by interacting with this field, analogous to moving through a thick syrup.
By explaining advanced theories in straightforward language, students and the general public can grasp the significance of the LHCโs findings.
Visual Aids and Infographics
Utilizing visuals can greatly enhance understanding. Infographics that depict particle interactions, the structure of the LHC, or the process of detecting particles help to effectively convey intricate information. Such aids can bridge the gap between complex scientific concepts and general comprehension, fostering a deeper interest in physics.
"The findings at the LHC not only expand our knowledge but challenge our understanding of the very fabric of the universe."
As we proceed into deeper discussions regarding the LHC, one must acknowledge its quintessential role in pushing the boundaries of science, making it an indispensable asset in the quest for knowledge.
Prolusion to the Large Hadron Collider
The Large Hadron Collider is a prominent facility in the field of particle physics. Its significance cannot be overstated. This section serves as an essential foundation for understanding the collider's role and impact in modern physics. By investigating the LHC, we delve into its design, functions, and the ambitious goals set forth by researchers.
Overview of CERN
CERN, the European Organization for Nuclear Research, is based in Geneva, Switzerland. Its mission is to advance scientific knowledge through the exploration of matter and the universe's origins. Founded in 1954, CERN has been at the forefront of revolutionary discoveries in physics. The institution consists of member states, mostly from Europe. Researchers collaborate here to conduct experiments on fundamental particles.
CERN houses several major facilities, with the Large Hadron Collider being the most notable. It is the world's most extensive particle accelerator, spanning approximately 17 miles. Scientists at CERN investigate the interactions between particles at high energy levels, shedding light on the building blocks of matter. The collaborative spirit at CERN allows scientists from diverse backgrounds to converge and share insights, pushing the boundaries of knowledge in a way that is both enriching and impactful.
Purpose of the LHC
The main purpose of the LHC is to investigate fundamental questions about the composition of matter and the forces that govern the universe. At its core, the LHC seeks to understand the Higgs boson, a particle that is crucial to the Standard Model of particle physics. Finding the Higgs boson helped to confirm existing theoretical frameworks and provided answers to pressing questions about mass and stability of the universe.
Another critical aspect of the LHC's purpose is to explore phenomena such as dark matter. Scientist hypothesize that dark matter makes up a substantial portion of the universe. However, its nature remains elusive. The LHC aims to discover potential candidates for dark matter particles, enhancing our understanding of these mysteries.
In summary, the LHC is a vital instrument in uncovering the secrets of the universe. Its contributions to particle physics illuminate pathways for future research and reveal the interconnectedness of various elements within the cosmos.
The LHC not only tests the tenets of existing physics but also seeks to unveil the realities beyond our current models, which could revolutionize our comprehension of nature itself.
Design and Structure of the LHC
The design and structure of the Large Hadron Collider are central to its function and effectiveness as the world's most powerful particle accelerator. Understanding how it is built and arranged helps grasp its role in contemporary physics research. The LHC is a marvel of engineering, demonstrating the intersection of sophisticated scientific principles and cutting-edge technology. Its configuration allows for the collision of protons at unprecedented energy levels, aiming to unlock the mysteries of the universe.
Technical Specifications
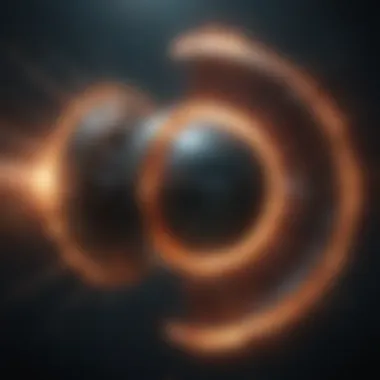
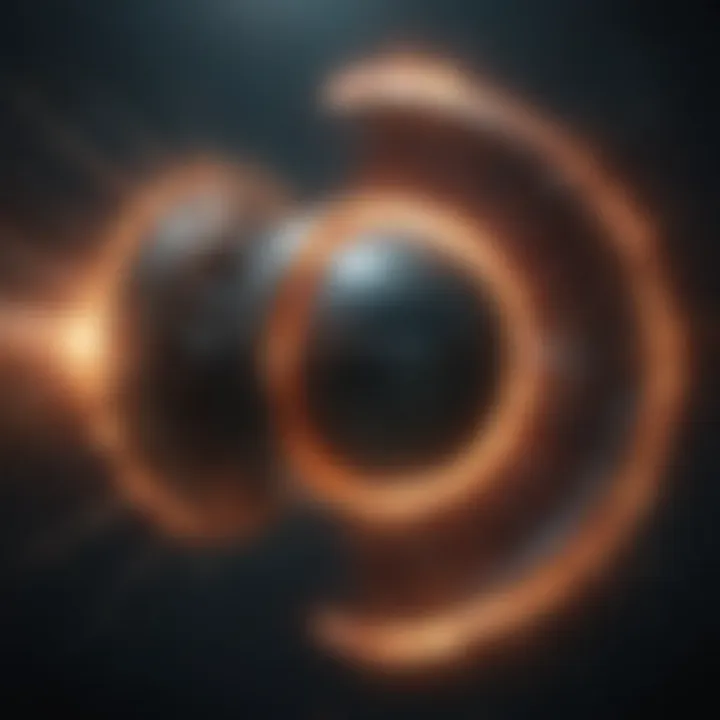
The LHC spans a circumference of about 27 kilometers (17 miles) and is located underground near Geneva, Switzerland. It consists of a complex system of superconducting magnets and detectors, which work together to accelerate particles to speeds comparable to that of light. The design incorporates advanced technology to ensure operational efficiency and safety. The collider operates at a magnet strength of 8.33 Tesla, which is sufficient to bend the paths of charged particles in their circular course.
Key specifications include:
- Energy Levels: Operating at 13 TeV (teraelectronvolts) during its latest run.
- Proton Beams: The LHC can collide multiple proton beams simultaneously, enhancing research scope.
- Cooling Systems: Utilizes liquid helium for magnet cooling to achieve superconductivity.
Components of the Collider
The components of the LHC are meticulously crafted for optimal performance. They encompass various parts that contribute significantly to its scientific ambitions.
Magnets
The LHC's magnets are essential for directing and focusing the particle beams. A notable unit, known as the dipole magnet, is pivotal in bending the particle paths around the collider. The key characteristic of these magnets is their superconductivity, which allows them to operate at high intensities without energy loss.
Benefits include:
- Efficiency: Superconducting magnets minimize energy consumption while maximizing performance.
- High Precision: They achieve precise control over particle trajectories, crucial for collision accuracy.
One possible disadvantage is the complexity of the cooling system required to maintain their superconductive state.
Detectors
Detectors in the LHC serve to observe and analyze the particles created in collision events. They capture data about particle interactions, allowing scientists to study phenomena like the Higgs boson. The key characteristic of these detectors, including the ATLAS and CMS, is their diverse array of technologies designed for different measurements.
Advantages include:
- Versatility: Capable of detecting various particle types and energies.
- Data Quality: High resolution and fast readout rates enhance data integrity.
However, a disadvantage can be the sheer volume of data that requires rigorous analysis processes.
Beam Pipes
The beam pipes are the vacuum tubes through which the particle beams travel. Maintained at a near-perfect vacuum, they minimize interference during particle acceleration. A key characteristic of the beam pipes is their ultra-smooth inner surfaces, which help reduce scattering of particles.
Advantages include:
- Controlled Environment: Ensures consistent conditions for particle travel.
- Reduced Loss: Limits particle loss due to interactions with residual gas.
On the downside, installation and maintenance can pose significant challenges.
"The construction and advanced design of the LHC highlight humanity's quest for understanding the fundamental nature of the universe."
Understanding the design and structure of the LHC helps appreciate its importance in modern physics. The comprehensive integration of various components ensures it remains at the forefront of particle physics research.
How the LHC Operates
Understanding how the Large Hadron Collider operates is vital for grasping its contributions to modern physics. This advanced accelerator system is instrumental in recreating conditions similar to those present just after the Big Bang. It allows scientists to observe fundamental particles and uncover their interactions, which can lead to groundbreaking discoveries regarding the fabric of the universe itself.
Particle Acceleration Process
The particle acceleration process is the backbone of the LHC's functionality. It involves a series of complex steps designed to accelerate protons and ions to nearly the speed of light. Initially, hydrogen atoms are stripped of their electrons to create protons. These protons are then injected into the linac, a linear accelerator, where they gain energy. Once accelerated, the protons enter the Proton Synchrotron and then the Super Proton Synchrotron to achieve even higher energies.
At this point, protons are situated in two beams which travel in opposite directions within a circular tunnel measuring 27 kilometers in circumference. When in position, magnetic fields direct these beams towards collision points within the collider's detectors. This process exemplifies the complex nature of the LHC and emphasizes the precision required to achieve the desired collision energies.
Collision Events
Collision events are critical as they provide a window into fundamental interactions. When the beams collide, the energies reach unprecedented levels. These moments create a variety of particles, including short-lived entities that can help scientists probe theories regarding particle physics. For example, studying the collision of protons has confirmed the existence of the Higgs boson.
Additionally, the detectors are equipped to capture the resulting data. Each event is analyzed to understand particle behavior and characteristics. This meticulous examination of collision events can yield insights about the fundamental forces that govern the universe. By using advanced algorithms and simulation models, researchers can decode the overwhelming data produced by each collision.
The sheer amount of data generated during collision events necessitates robust data processing capabilities, helping scientists sift through thousands of events to isolate significant findings.
Major Discoveries at the LHC
The Large Hadron Collider has played a pivotal role in enhancing our understanding of fundamental physics. The discoveries made at the LHC are not only groundbreaking but also reshape the way we understand matter and energy in the universe. Each significant finding contributes to the larger framework of particle physics, confirming or contradicting existing theories and pushing the boundaries of scientific knowledge.
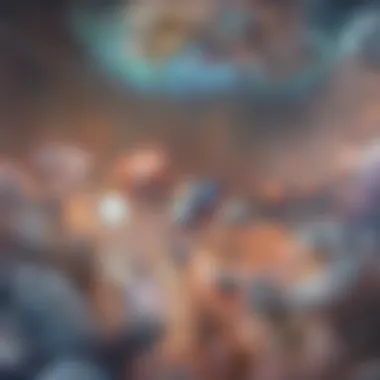
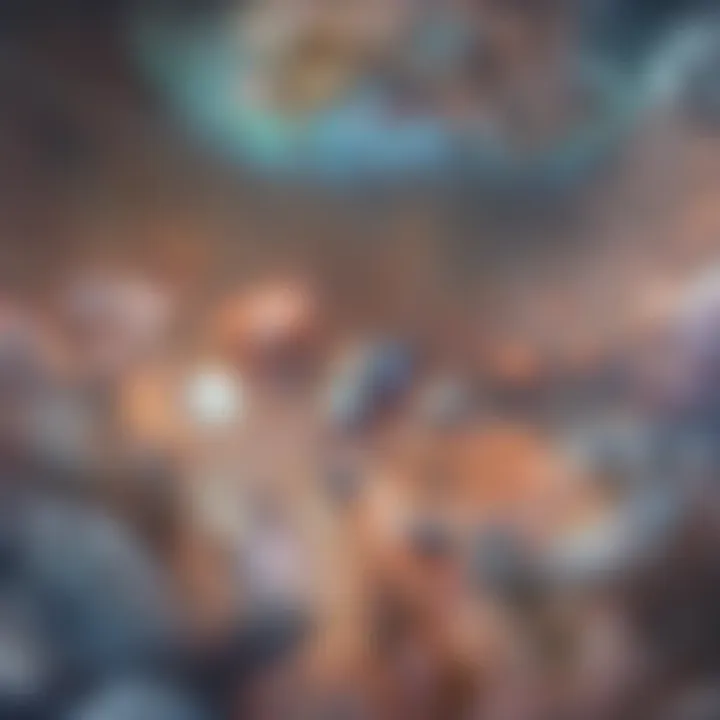
Higgs Boson Discovery
The discovery of the Higgs boson in 2012 marked a monumental moment in particle physics. This particle is crucial because it confirms the existence of the Higgs field, which is responsible for giving mass to other particles. Theoretically proposed in the 1960s, the Higgs boson's search mobilized a vast scientific effort and resources. Its discovery at the LHC is a testament to the collider's capabilities.
Researchers identified the Higgs boson by analyzing the decay products of high-energy proton collisions. The LHC experiments ATLAS and CMS detected signals consistent with the properties of the Higgs boson. This confirmed its existence and solidified the validity of the Standard Model of particle physics.
The Higgs boson discovery not only verified a fundamental aspect of the Standard Model but also opened new avenues for research into unknown realms of particle physics.
Search for Supersymmetry
Another significant area of research at the LHC is the search for supersymmetry. Supersymmetry is a theoretical framework that posits each particle has a superpartner, doubling the number of fundamental particles. This theory could address several unresolved questions in physics, such as the nature of dark matter.
Despite extensive searches at the LHC, no direct evidence of supersymmetric particles has been found yet. However, researchers continue to analyze collision data in hopes of uncovering hints of these particles. The ongoing efforts signify the collider's ability to probe and challenge existing theoretical frameworks, even while specific results remain elusive.
Studies on Dark Matter
Studies on dark matter represent another frontier of exploration at the LHC. Dark matter is believed to compose approximately 27% of the universe's mass-energy content yet remains undetectable by conventional means. The LHC's high-energy collisions are thought to potentially create dark matter particles.
Researchers look for missing energy signatures in collision events, which could indicate the production of dark matter particles. The findings related to dark matter at the LHC help refine models and theories surrounding its properties. Additionally, collaborations with astrophysics continue to explore connections between cosmic phenomena and collider experiments.
By advancing our understanding of these fundamental aspects of the universe, the LHC continues to be at the forefront of modern physics research.
Theoretical Implications of LHC Findings
The findings from the Large Hadron Collider (LHC) resonate deeply within the fabric of theoretical physics. They challenge existing paradigms and propose new avenues for understanding the universe. The implications of these discoveries extend far beyond the experimental data. They touch on fundamental questions surrounding the basic building blocks of matter and the forces that govern them.
At the core of particle physics resides the Standard Model. This model describes how particles interact through fundamental forces. The LHC's discoveries have either reinforced or questioned elements of this model, prompting theorists to reassess the entire landscape of particle interactions. Any deviation from predicted behavior could signal new physics, laying groundwork for theories that integrate previous understandings with newfound data.
Standard Model of Particle Physics
The Standard Model has been a cornerstone of modern physics since the mid-20th century. It categorizes all known elementary particles and describes three of the four fundamental forces, excluding gravity. The LHC's capability to collide protons at unprecedented energies has enabled physicists to confirm many aspects of the model, especially the existence of the Higgs boson.
- Higgs Boson: Its discovery in 2012 was a significant milestone, confirming the mechanism that gives mass to particles. This finding not only validated the Standard Model but also bolstered confidence in theoretical predictions surrounding particle behavior.
- Other Particles: The LHC continues to search for various predicted particles, such as those related to dark matter and supersymmetry, which could suggest extensions to the Standard Model.
A crucial element of the Standard Model is that it is incomplete. For instance, it cannot explain dark matter or gravity's role. The LHC's experiments contribute data that may illustrate shortcomings, urging physicists to expand their theoretical frameworks.
Beyond the Standard Model
Considering the limitations of the Standard Model leads to the exploration of theories beyond it. Physicists are investigating several concepts, including supersymmetry, string theory, and other potential interactions.
- Supersymmetry: This theory posits that every particle has a superpartner, which could help unify the forces and offer solutions to dark matter. The LHC has not yet found these particles, but ongoing experiments may change this.
- Extra Dimensions: The existence of additional spatial dimensions beyond our conventional three could explain various phenomena, including the weakness of gravitational force relative to other fundamental forces. The LHC experiments seek to uncover evidence supporting or refuting such theories.
The search for new physics is ambitious. The LHC operates at the frontier of scientific inquiry, aiming to push boundaries that govern our understanding of reality. Findings from experiments not only fill gaps but also ignite deeper questions about the universe.
"The discoveries at the LHC may lead us into uncharted territories of theoretical physics, expanding our understanding and potentially rewriting the laws that govern our universe."
As research continues, the implications of the LHCโs findings remain profound. Every piece of data collected leads theorists closer to unraveling mysteries associated with the fundamental nature of matter, energy, and the cosmos.
International Collaboration in Research
International collaboration plays a vital role in the research conducted at the Large Hadron Collider (LHC). The very design and operation of the LHC embodies a spirit of global partnership. Thousands of scientists, engineers, and support staff from over 100 countries converge at CERN to push the frontiers of knowledge. This collaborative approach enhances the innovation, diversity of ideas, and resource sharing that are fundamental in conducting high-level scientific research.
One significant aspect of international collaboration is the pooling of expertise from different countries. Each participating nation brings its unique strengths, whether in theoretical physics, engineering, or computational science. For instance, researchers from Japan excel in detector technologies, while contributions from the United States and European countries may focus on advanced computing capabilities. Such synergy fosters a dynamic research environment.
Moreover, the sharing of resources is another key benefit. Constructing and operating a facility of this magnitude demands substantial financial and technical investment that is beyond the capacity of a single entity. By collaborating, countries share these burdens, making it feasible to maintain and upgrade the LHC. This model has transformed particle physics into a truly global endeavor.
As an example of successful international collaboration, the discovery of the Higgs boson in 2012 demonstrated how collective efforts yield monumental achievements. Scientists across various institutions analyzed data and worked together to verify the existence of this elusive particle.
"The LHC is not just a machine; it is a symbol of what we can achieve when we unite our efforts across borders."
Global Participation
The LHC is a veritable melting pot of scientific talent, attracting researchers from diverse academic backgrounds and cultures worldwide. The global participation aspect is reflected in the diverse array of scientific disciplines involved, including theoretical physics, engineering, and computational analytics.
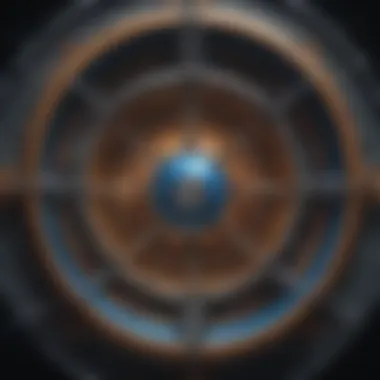
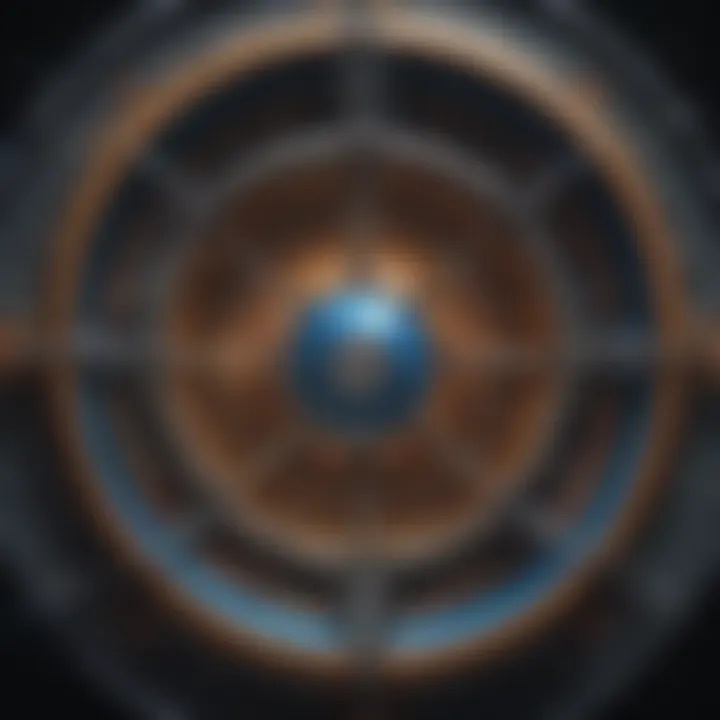
Some notable points about global participation include:
- Countries like France, Germany, เคญเคพเคฐเคค (India), and the United States have made immense contributions in terms of personnel and technology.
- Major institutions like MIT, Stanford University, and the University of Tokyo are key players in the collaborative research efforts at LHC.
- Global collaboration platforms facilitate the training and development of young scientists, allowing them hands-on experience in cutting-edge research.
These factors create a rich environment for innovation and learning, fostering international ties among future generations of scientists.
Impact on Scientific Community
The impact on the scientific community resulting from the LHC's international collaboration is profound. By working collaboratively, scientists are not only able to enhance their individual research but also contribute to a collective knowledge base that benefits everyone involved.
- Shared Knowledge: Researchers develop new techniques and share findings across borders, which catalyzes advancements in fields beyond particle physics, like materials science and medical imaging.
- Career Development: Young researchers gain exposure to international standards of research, creating a generation of scientists adept in global collaboration.
- Public Outreach: Joint efforts in communicating scientific findings to the public elevate the visibility of science, fostering greater engagement and enthusiasm towards scientific inquiry.
In summary, the LHC serves as a pivotal model for international research collaboration. The comprehensive impact on the scientific community is reflected in the ways knowledge, career growth, and public interest are cultivated globally.
Challenges and Controversies
The exploration of the universe using the Large Hadron Collider (LHC) is not without its hurdles. Understanding the challenges and controversies surrounding this monumental project is crucial for comprehending its impact in the realm of physics. These issues affect public perception, funding, and the scientific community's confidence in such large-scale experiments. This section will delve into the technical difficulties faced by the LHC, as well as the public misconceptions that often cloud discussions about its importance.
Technical Difficulties
Operating the LHC involves a complex network of sophisticated technologies. One of the primary challenges has been ensuring the collider's functionality and reliability during experiments. The LHC is designed to achieve unprecedented collision energies, which can sometimes lead to malfunctions and unexpected issues.
For example, in 2008, shortly after the LHC's first startup, a fault occurred in the superconducting magnets, resulting in a two-year delay for repairs. Such incidents not only set back timelines but also increase costs significantly. Continuous upgrades and maintenance are essential for keeping the LHC operational. This ongoing work requires substantial expertise and resources, raising questions about the sustainability of similar future projects.
Moreover, the sheer scale of data generated during particle collisions is another challenge. Each collision creates vast amounts of data that need to be processed and analyzed. The current data handling systems must be constantly refined to manage the influx of information efficiently. Failures in data management can lead to wasted experiments and missed discoveries.
Public Misconceptions
Despite significant advancements, the Large Hadron Collider has faced a myriad of misconceptions from the public. One of the most notable is the fear that the LHC could create black holes that might consume the Earth. This notion, while dramatic, is grounded in misunderstanding. Theoretical physicists assert that if micro black holes were to form, they would evaporate almost instantly due to Hawking radiation.
Another common misconception relates to the nature of the experiments conducted at the LHC. Many people assume that experiments are only geared toward understanding the Higgs boson. While the Higgs boson is a centerpiece of research, the LHC investigates a plethora of other topics, including dark matter and supersymmetry. These topics, while less sensational, are fundamentally crucial for developing a comprehensive understanding of the universe's architecture.
To alleviate these misconceptions, communication between scientists and the public is essential. Clarity in explaining complex theories can build trust and demystify the work being done at facilities like CERN. Initiatives to improve public understanding must focus on educating and addressing concerns, thereby fostering a more informed dialogue about the scientific work at hand.
Future of the Large Hadron Collider
The future of the Large Hadron Collider (LHC) is a topic of significant relevance, as it continues to shape the understanding of fundamental physics. With upcoming developments and experiments, the LHC stands as a central tool for the scientific community. Its role in investigating the mysteries of particle physics has profound implications not only for science but also for technological advancements in various fields.
Upcoming Experiments
The LHC's future is marked by exciting upcoming experiments that aim to probe deeper into the fabric of the universe. On the horizon are several experiments designed to investigate areas such as:
- High-Luminosity LHC (HL-LHC): This upgrade will increase the collision rate, allowing physicists to gather more data and improve the precision of measurements. It aims to enhance the discovery potential for new particles and phenomena.
- Search for New Physics: The LHC will continue its hunt for signs of new physics beyond the Standard Model. This includes searching for supersymmetric particles, dark matter candidates, and exploring the nature of forces that govern matter.
- Quantum Chromodynamics (QCD) Studies: Further investigations into QCD will help physicists understand the strong force better, contributing to our knowledge of how particles interact under extreme conditions.
These experiments are expected to yield insights that could revolutionize current physics theories and potentially lead to groundbreaking discoveries.
Long-term Goals of CERN
CERN has defined several long-term goals that outline its research priorities and objectives. These goals reflect a commitment to advancing knowledge and technology. Key aims include:
- Enhancing Scientific Knowledge: CERN seeks to unravel the fundamental laws of nature, aiming to provide answers to pressing questions about the universe, such as the existence of extra dimensions or the nature of dark energy.
- Technological Development: Advances from the LHC also drive innovation in other sectors. Technologies developed for particle detection and acceleration are being adapted for medical imaging, radiation therapy, and data processing.
- Educational Outreach: CERN aims to maintain its role as a hub for global scientific collaboration. It focuses on educating the next generation of scientists and disseminating knowledge to a broader audience.
In summary, the future of the Large Hadron Collider is bright, with many thrilling experiments on the way. The aspirations of CERN, along with the ambitions of the global scientific community, ensure that it will remain at the forefront of particle physics for years to come.
Finale
The conclusion of this article highlights the pivotal role of the Large Hadron Collider in modern physics. The LHC is not merely a complex assembly of machinery; it is a beacon of exploration into the unknown realms of the universe. As scientists continue to decipher the mysteries surrounding elementary particles, the LHC's significance becomes ever clearer.
Significance in Modern Physics
The discoveries made at the LHC have profound implications. First, the confirmation of the Higgs bosonโs existence has provided much-needed support for the Standard Model of particle physics. This model has been the cornerstone of physics for decades, allowing for the prediction of particle behaviors. The findings at the LHC also propel future research, fostering a deeper understanding of the forces that govern the universe.
Moreover, the LHC is essential for examining potential new physics beyond the Standard Model. This pursuit includes exploring phenomena such as supersymmetry and dark matter, areas that could reshape our understanding of the cosmos. Thus, the LHC is not just a device for verification but a launchpad for future theories and discoveries.
Legacy of the LHC
The legacy of the LHC transcends its specific findings. It exemplifies the spirit of international collaboration, as diverse teams of scientists from around the world converge to explore fundamental questions. The technological advancements achieved through the LHC project, including innovations in data analysis and detector technology, have applications that extend beyond particle physics.
Additionally, the LHC serves as a powerful educational tool, inspiring new generations of physicists. The excitement and curiosity it generates can lead to greater public interest in science and technology. Its impact will likely continue to resonate, influencing both scientific inquiry and educational pursuits.
In summary, the Large Hadron Collider stands as a pivotal instrument for understanding the universe. Its discoveries not only affirm existing theories but also open pathways to new knowledge. As science progresses, the legacy of the LHC will undoubtedly play a critical role in shaping our comprehension of the fundamental components of reality.