In-Depth Analysis of Particles and Their Interactions
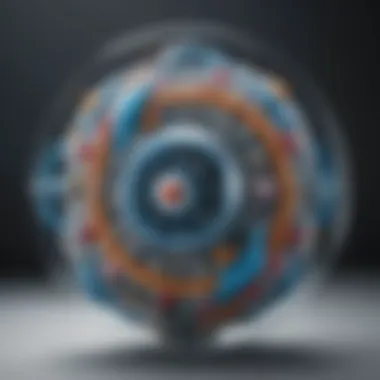
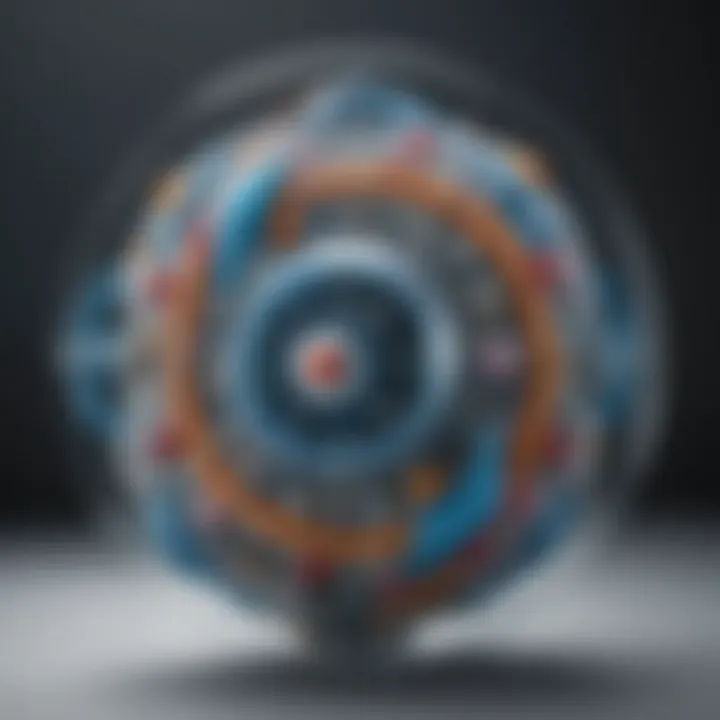
Intro
Particles are the building blocks of our physical world, characterizing everything from the smallest atom to expansive cosmic structures. The scientific inquiry into particles goes beyond mere definitions; it unravels the underlying principles that govern matter and energy. This exploration not only focuses on foundational elements like quarks and leptons but also delves into composite entities such as baryons and mesons. Each particle type has distinct properties and interactions, offering a comprehensive understanding of their roles across various disciplines within physics and beyond.
Our journey into the realm of particles is fueled by a rich history of discoveries and advancements. As we unravel the layers of subatomic particles, we find that their significance stretches into realms like technology and medicine, where they play a pivotal role in innovations such as medical imaging and particle acceleration. By shedding light on these concepts, the aim here is to provide clarity and depth, ensuring readers can navigate the complex landscape of particle physics with confidence.
Key Research Findings
Overview of Recent Discoveries
Recent advancements in particle physics have unveiled numerous insights, providing a deeper understanding of the fundamental components that make up our universe. Notably, the discovery of the Higgs boson at CERN raised profound questions about mass and its formation in the universe. This particle's existence was a missing piece of the Standard Model, a theoretical framework that describes electromagnetic, weak, and strong nuclear interactions. In 2020, researchers confirmed evidence of a new exotic meson which challenges current concepts of particle classification, prompting a reevaluation of established theories.
Significance of Findings in the Field
These groundbreaking findings have profound implications:
- Fragile Balance: The interactions between different particle types underscore the delicate balance of forces that govern matter.
- Technological Innovation: Advances in identifying particles contribute to the enhancement of technologies like quantum computing and hadron therapy for cancer patients.
Above all, every new finding feeds into our understanding of the universe and helps us shape new technologies that benefit society.
Breakdown of Complex Concepts
Simplification of Advanced Theories
Understanding particle physics often feels like reading a novel in an unfamiliar language. Complex theories such as Quantum Chromodynamics or Electroweak Unification can be intimidating. By breaking them down into digestible parts, we can gain clarity.
- Quantum Chromodynamics (QCD): At its core, QCD explains how quarks and gluons interact, held together by the strong force. Think of quarks as ingredients and gluons as the chef that binds them.
- Electroweak Theory: This theory unifies electromagnetic and weak forces. Imagine combining two ingredients that seem different but when mixed, create a more powerful dish.
Visual Aids and Infographics
To grasp particle interactions visually,
"A picture is worth a thousand words." Utilizing diagrams and infographics serves as an effective tool, especially for complex concepts. Infographics can illustrate fundamental particle types, their charges, and interactions, presenting a clearer picture that complements written explanations. Incorporating well-designed graphs allows students and professionals to visualize relationships and properties, ushering in deeper comprehension.
For further exploration and global perspectives on particles, consider visiting resources such as Wikipedia and Britannica.
Taking the time to engage with these concepts enriches our understanding of the intricate patterns and forces shaping our universe.
Preface to Particles
Understanding particles isn't just a walk in the park; it's an essential foundation for grasping the very essence of matter that shapes our universe. In this article, we're diving headfirst into the realm of particles, where we'll dissect their types, behaviors, and the intricate web of interactions that define them. From the smallest subatomic constituents to larger combinations, particles provide a lens through which we can observe the universe's inner workings.
Defining Particles
At its core, a particle can be seen as the basic building block of all matter. Defined primarily in the context of physics and chemistry, particles can range from fundamental entities like electrons and quarks to more complex forms like atoms and molecules. To break it down:
- Fundamental Particles: These are the indivisible components that include quarks and leptons, serving as the foundational bricks of atoms.
- Composite Particles: These are formed from combinations of fundamental particles, such as protons and neutrons, which themselves make up atoms.
This breadth of definition leads us to appreciate particle physics not just as an academic endeavor but as the foundation upon which all physical sciences build.
Historical Context of Particle Physics
When one steps back to view the trajectory of particle physics, it's staggering to see how far we've come. The saga began long ago, tracing back to thinkers like Democritus, who first theorized that everything is composed of tiny, indivisible units he termed "atomos." Fast-forward to the early 20th century, and revolutionary experiments radically redefined our understanding of atomic structure. Ernest Rutherford’s gold foil experiment unveiled the nucleus, highlighting a complex atomic architecture.
In recent decades, the discoveries of the electron, neutrinos, and the lavishly theorized Higgs boson have cemented particle physics as a cornerstone of modern science. The journey is not just a timeline of discoveries but also one of collaborative effort, from large-scale projects like CERN to the contributions of individual scientists worldwide.
Importance of Studying Particles
Why should we even care about particles? For starters, studying particles provides profound insights into the fundamental forces of nature. This includes everything from gravity to electromagnetism, influencing how we understand the physical world around us. Additionally, progress in particle physics has paved the way for breakthroughs in numerous fields, including medical imaging technologies and advancements in materials science.
- Practical Applications:
- Particle accelerators have led to the development of treatments for cancer, specifically through radiation therapy.
- Innovations in technology, like semiconductors, stemmed from understanding particle behavior.
"The study of particles isn’t merely theoretical; it offers tangible benefits that ripple through various domains of human activity."
In a nutshell, the exploration of particles not only sheds light on the underpinnings of matter but also propels us toward future innovations, making our quest for knowledge all the more critical.
Fundamental Particles
Fundamental particles serve as the building blocks of matter in the universe. They are the essential constituents that interact and combine in various ways to form everything around us. Understanding these particles is key in unraveling the complexities of the physical world. In this section, we will take a closer look at three main categories of fundamental particles: quarks, leptons, and gauge bosons.
Quarks
Quarks are elementary particles that combine to form protons and neutrons, which in turn make up the atomic nucleus. They come in six types, often called flavors: up, down, charm, strange, top, and bottom. Each quark flavor has its own unique properties, making them a diverse and intriguing topic of study.
Types of Quarks
The significance of quarks lies in their different types. Each type has a distinct charge and contributes uniquely to the properties of matter.
- Up Quark: Positive charge of +2/3, a key player in forming protons.
- Down Quark: Negative charge of -1/3, essential for neutrons.
- Charm, Strange, Top, Bottom: These heavier quarks add complexity to the particle spectrum but are less common in ordinary matter.
The presence of multiple types reflects the diversity in subatomic interactions. When exploring particle physics, distinguishing these types becomes crucial, as they govern the stability and behavior of matter itself.
Quark Combinations
Quark combinations are fundamental in constructing baryons (such as protons and neutrons) and mesons. The way quarks arrange themselves influences the characteristics of these particles. A baryon consists of three quarks while a meson is made of a quark and an antiquark.
- Baryon Examples: Proton (uud), Neutron (udd).
- Meson Examples: Pion (uard), Kaon (saru).
The combinations determine the mass, charge, and stability of the particles formed. Thus, comprehending these combinations enables deeper insights into the forces that hold matter together in the universe.
Role in Particle Formation
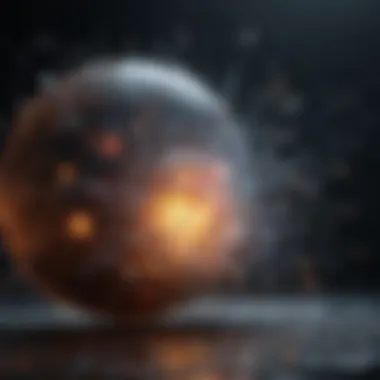
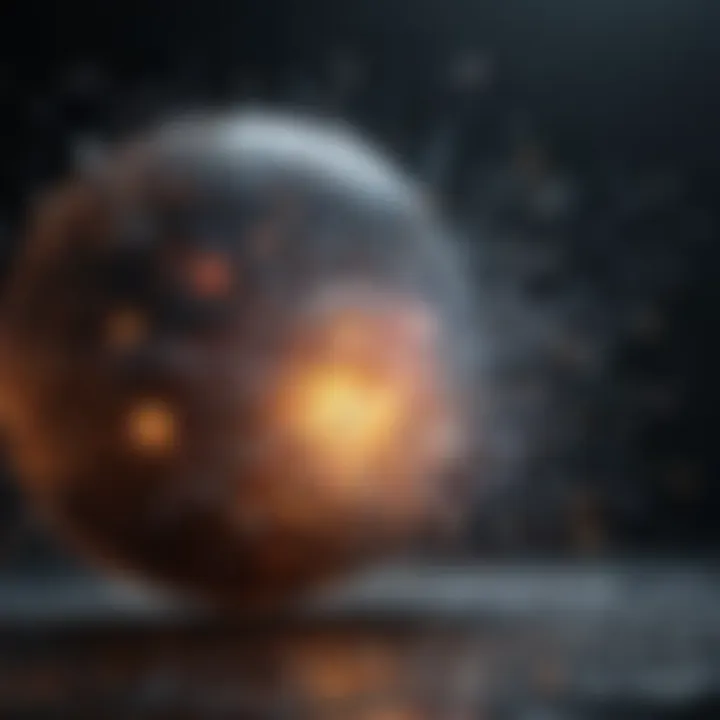
Quarks are indispensable in the process of particle formation. They interact via the strong force, mediated by gluons, which bind them together. This interaction is not only pivotal in creating baryons and mesons, but also contributes to the overall structure of matter.
- Gluons: Gauge bosons that act as the force carriers for the strong interaction between quarks.
Understanding quarks' various roles aids in deciphering the origins of matter and the unique properties that arise from these tiny building blocks.
Leptons
Leptons are another category of fundamental particles that do not participate in strong interactions, unlike quarks. The most well-known lepton is the electron, but there are others too. These particles carry a charge of either +1 for electrons or -1 for positrons, with neutrinos being neutral.
Types of Leptons
The six types of leptons include the electron, muon, tau, and their respective neutrinos. Each lepton contributes diversely to phenomena like weak interactions and lepton flavor.
- Electron: Integral for electricity and chemistry.
- Muon: Heavier cousin of the electron, mostly observed in high-energy events.
- Tau: Even heavier, but short-lived.
- Neutrinos: Nearly massless and interact very weakly with matter.
The types highlight the diverse roles leptons play in particle physics. Their varying masses and interactions open up discussions about the fundamental workings of the universe.
Characteristics of Leptons
Leptons exhibit unique characteristics that set them apart from other particles. Their ability to exist in three generations (electron, muon, tau) allows for different physical behaviors.
- Charge: As mentioned, leptons can be charged or neutral, which affects their interactions.
- Mass: The mass difference among types can influence decay rates and particle behavior.
These characteristics make them essential in understanding decay processes and interactions within the universe.
Significance in Physics
Leptons play a crucial role in the Standard Model of particle physics, showcasing weak nuclear forces and decay processes like beta decay. They are vital for understanding the universe's fundamental structure and behaviour. Their studies lead to observable phenomena in astrophysics and cosmology.
Gauge Bosons
Gauge bosons are the force carriers mediating the electromagnetic, weak, and strong forces. They are crucial for the interactions among fundamental particles. Understanding these bosons is essential in particle physics since they govern the fundamental forces in nature.
Photons
Photons are the gauge bosons of the electromagnetic force. They are massless and travel at the speed of light. Understanding photons helps explain electromagnetic phenomena, like light and radio waves.
- Key Characteristic: They exhibit wave-particle duality, behaving as both particles and waves.
Their massless nature allows them to carry energy across vast distances, making them a significant topic of discussion in quantum mechanics and thermodynamics.
W and Z Bosons
The W and Z bosons are responsible for mediating weak nuclear forces, involved in processes such as radioactive decay.
- W Boson: Has a charge; responsible for the transformation of different types of particles.
- Z Boson: Neutral and mediates neutral currents.
Both play an integral role in the weak force interactions that dominate at subatomic levels.
Gravitons Hypothetical Nature
Gravitons are hypothetical gauge bosons that are theorized to mediate the gravitational force. While they have not been detected, their existence is postulated in quantum gravity theories.
- Unique Feature: They are expected to be massless like photons, allowing gravitational forces to propagate through space.
Understanding gravitons offers potential pathways into unifying general relativity with quantum mechanics, a challenge faced by physicists today.
"The exploration of fundamental particles provides the foundational knowledge of how the universe operates at its core. Understanding these elements ultimately informs our grasp of reality."
Composite Particles
Composite particles play a significant role in our understanding of the universe. Unlike fundamental particles, which are the building blocks of matter, composite particles are formed from combinations of two or more fundamental particles. This structure not only enriches our grasp of particle physics but also illustrates the complexity of interactions at the subatomic level. By examining composite particles, we gain insights into how matter behaves under various conditions, how forces interact, and how the cosmos is fundamentally constructed.
Baryons
Definition and Structure
Baryons are a class of composite particles made up of three quarks. Their structure is unique in that they primarily exist due to the strong force, which binds their constituent quarks together. This multiquark arrangement provides baryons with significant properties, such as having a half-integer spin, which classifies them as fermions.
The stability and mass of baryons come from the interplay between the quarks and gluons that hold them tightly together. This structure is not just a fascinating detail; it forms the backbone of most visible matter in the universe, including protons and neutrons, the very components of atomic nuclei.
Types of Baryons
There are different types of baryons, which can be categorized mainly into baryon resonances and stable baryons. Stable baryons include protons and neutrons, which are vital for the structure of atoms as we know them. Baryon resonances, on the other hand, are particles that exist only for a fleeting moment before decaying into lighter particles.
The distinction between these types illustrates the varied aspects and roles of baryons in both experiments and our theoretical understanding of particle physics. Stable baryons are crucial for building atoms, thereby being essential in chemistry and biology, while resonances reveal details about strong force dynamics and particle interactions during high-energy collisions.
Importance in Astrophysics
In astrophysics, baryons are critical as they account for a significant portion of the normal matter in the universe. Understanding baryonic matter helps us explore profound questions about the structure of galaxies and the formation of stars. Their properties, interactions, and abundance offer insights into the evolution of the universe.
Baryons' gravitational effects also play a role in explaining cosmic phenomena, such as dark matter's influence on galactic structures. When delving into observations of cosmic microwave background radiation or the formation of cosmic structures, baryons are central to our interpretations. This makes them a topic of high relevance, as they help bridge the gap between fundamental physics and cosmological models.
Mesons
Definition and Formation
Mesons are another type of composite particle but are distinct in that they are formed from a quark and an antiquark pair. This structure gives mesons a unique identity as they are bosons, possessing integer spin. The formation process is primarily mediated by the strong force from gluons, which leads to their relatively short-lived existence compared to baryons.
The simplicity of mesons, relative to baryons, illustrates essential elements of quantum chromodynamics, which is the theory describing strong interactions. This makes mesons a focal point for understanding how particles interact and how forces govern their behavior in the subatomic realm.
Different Meson Types
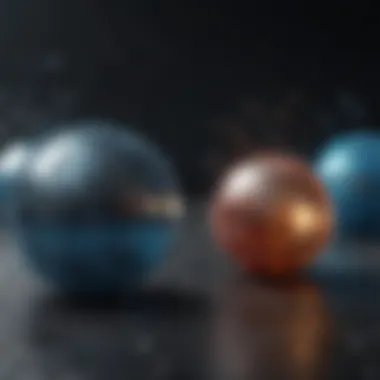
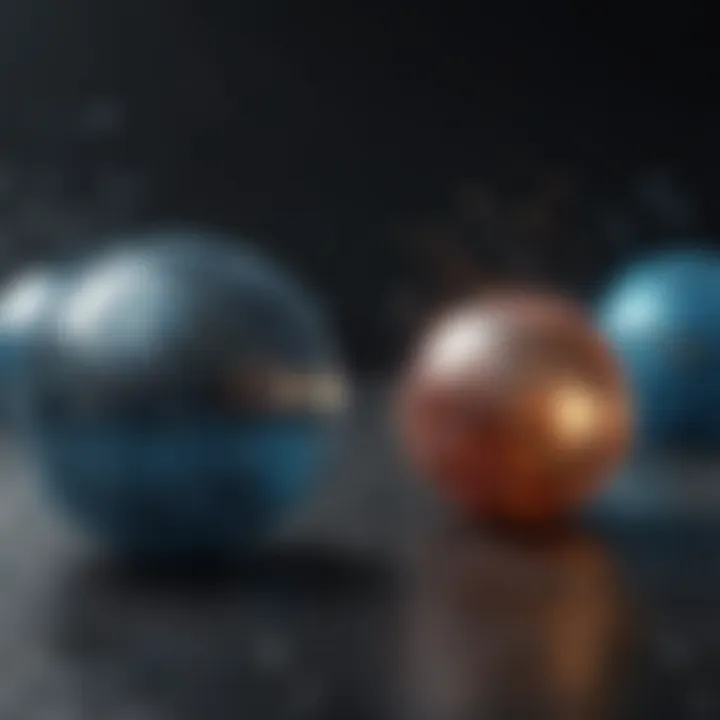
There is a variety of mesons categorized based on their quark content and quantum properties. For instance, the pion is vital in mediating the strong force between baryons, while kaons and other heavier mesons provide insights into flavor physics. Each meson type has different contributions to interactions in particle colliders and nuclear reactions, demonstrating their importance in experiments designed to unearth the mysteries of the universe.
Generating a deeper understanding of these meson types allows physicists to assess the validity of quantum theories and enhances overall knowledge of particle physics applications.
Role in Strong Force
Mesons have a pivotal role in mediating the strong force between baryons. This force is responsible for binding protons and neutrons within an atomic nucleus, making mesons essential players in nuclear stability. Their presence is vital in various processes, including nuclear decay and particle production in high-energy physics laboratories.
The study of how mesons operate within the strong force helps physicists refine models of atomic models and nuclear behavior. Interactions involving mesons can also lead to new paradigms in understanding how matter behaves under extreme conditions, such as those found in neutron stars or during cosmic events.
Mesons not just connect the dots between quarks and baryons, but play a crucial role in the fundamental interactions that govern the universe.
Interactions and Forces
Understanding the concept of interactions and forces is essential when discussing particles. They govern how particles behave, interact with one another, and ultimately shape the universe's structure. In this section, we will unpack the fundamental forces that are pivotal in particle physics. Each force holds a unique role, providing a framework for understanding the intricate web of interactions that occur in nature.
Fundamental Forces
The world of fundamental forces can be likened to the strings of a complex violin, each producing a note that contributes to the symmetry and harmony of the universe. Here are the four primary forces that act among particles:
Gravitational Force
The gravitational force is perhaps the most familiar to us. It’s the pull that keeps our feet on the ground and governs the motion of celestial bodies. But in the particle realm, its implications are less about falling apples than they are about cosmic scale structures.
One significant characteristic of the gravitational force is its long-range nature, meaning it can affect objects over vast distances. The overarching influence of gravity extends to every particle in the universe, albeit its effectiveness diminishes with distance. This makes it a crucial force for comprehending the universe's larger-scale structures like galaxies, but relatively weak when considering atomic and subatomic interactions.
While not often a focus in particle physics due to its relative weakness compared to other forces, gravity retains its unique feature of simplicity—being described by a single law, Newton's Law of Universal Gravitation. In the context of this article, highlighting its grandeur helps underline the scale over which different forces operate, providing a backdrop against which electromagnetic and nuclear forces come into play.
Electromagnetic Force
Next comes the electromagnetic force, which is indeed a heavyweight in terms of influence at the atomic and molecular levels. This is the force responsible for electricity and magnetism, and it plays a crucial role in how particles interact with each other through charged exchanges. Think of it as the invisible handshake between charged particles.
A key characteristic of the electromagnetic force is that it can both attract and repel. This duality makes it intricate and fascinating. Unlike gravity, it operates far more effectively at short ranges, influencing particle interactions in atoms' shells. Its strength is manifested in binding electrons to protons, leading to the formation of atoms.
The unique feature of this force includes not just its strong influence but also its capacity to enable chemical reactions and form various states of matter. Its impact on particle physics cannot be understated, as the interactions derived from electromagnetic forces are foundational for all of chemistry.
Strong and Weak Nuclear Forces
Now, let’s delve into the strong and weak nuclear forces that play pivotal roles within the atomic nucleus. They might be less familiar but are critical in maintaining the structure of matter itself. The strong force operates as the glue that holds protons and neutrons tightly together in atomic nuclei. Without this force, the same protons would repel each other due to their positive charge, and nuclei could not exist.
The strong nuclear force is incredibly powerful but operates over an extremely short range—only effective within the confines of an atomic nucleus, hence its ability to create stability at that scale. A benefit of this force is the formation of heavier elements and the robustness of the atomic structure. Conversely, this power also has disadvantages; the energy locked in nuclei can lead to nuclear reactions, both beneficial (nuclear power) and destructive (nuclear warfare).
In contrast, the weak nuclear force plays a vital part in processes that involve subatomic particles known as leptons. It is responsible for processes like beta decay in radioactive materials. While not as strong as the strong force, it has unique strengths, such as allowing particles to change types. This feature makes the weak force fascinating because it results in various particle interactions that lead to the formation of neutrinos and, by extension, has implications for our understanding of the universe's evolution.
Quantum Field Theory
In the realm of particle interactions and forces, quantum field theory emerges as a cornerstone of modern physics. This theory treats particles as excited states of underlying fields, fundamentally reshaping how we perceive the universe. It fundamentally integrates the quantum mechanics of micro-scale actions with the classical forces discussed. In simple terms, if particles are the actors in a theater, then quantum fields are the stage on which their drama unfolds.
Particle Interactions
The interactions between particles can be thought of as a complex dance, where each force acts as a choreographer guiding how closely or loosely they move together. Understanding these interactions deepens our grasp of particle physics and the forces at play.
Scattering Processes
One core aspect of particle interactions is scattering processes. These occur when particles collide and interact, resulting in new particles or states being produced. They are vital for experimental endeavors, like those conducted in particle accelerators, where understanding how particles scatter can unveil the properties of their interactions.
Scattering processes are beneficial because they allow researchers to test theoretical predictions against experimental results. A unique feature of this aspect is that they can reveal information about the internal structure of particles. However, they can also lead to complexities in analyzing outcomes, with a wide array of potential result types based on energy levels and angles.
Virtual Particles
Virtual particles might seem a bit counterintuitive, as they exist in transient states that aren't directly observable. However, they play crucial roles in mediating forces between real particles. The concept may sound a bit peculiar, but it emphasizes the flexibility and imagination that quantum physics embodies.
Their characteristic nature involves popping in and out of existence over minuscule time frames, facilitating interactions between forces. This transient feature leads to a deeper understanding of how forces operate, as virtual particles are responsible for the exchange of momentum and energy between interacting particles. One might argue the advantage of this concept lies in its ability to describe complex interactions succinctly, though the drawback rests in the abstract nature that can make it harder to visualize.
Feynman Diagrams
Feynman diagrams offer a visual representation of particle interactions, serving as a map for understanding the processes described above. Introduced by physicist Richard Feynman, these diagrams illustrate how particles interact through mediating forces, presenting a compact yet rich insight into particle behavior. They distill complex calculations and interactions into easily digestible images.
The key characteristic of Feynman diagrams is their ability to simplify complex quantum interactions into a more manageable format. This simplification is beneficial, especially when the labyrinth of interactions can be overwhelming. On the flip side, mastering the intricate rules for interpreting these diagrams can require practice, which might deter beginners.
In summary, the realm of interactions and forces provides the structural backbone to particle physics, unearthing profound insights into the universe. Gravitational, electromagnetic, and nuclear interactions collectively inform our understanding of everything from the tiniest particles to the grandest cosmic phenomena.
Applications of Particle Physics
The field of particle physics, often tucked away in the corners of complex scientific discussions, has profound implications in everyday life. Understanding how particles interact with one another not only feeds our curiosity about the universe but also propels technological innovations across various sectors. The practical applications of particle research stretch from the medical field to advancements in technology, ultimately helping society tackle real-world challenges.
Healthcare Innovations
Radiation Therapy
Radiation therapy is a treatment option predominantly used to combat cancer. The process involves targeting cancerous cells with high-energy particles, such as protons or X-rays, to damage their DNA and inhibit their replication. What sets radiation therapy apart is its precision—modern advancements allow oncologists to deliver doses that maximize tumor shrinkage while sparing healthy tissue as much as possible. This makes it not just a functional choice but an immensely beneficial one for patients.
The unique feature of radiation therapy lies in its ability to adapt to individual patient needs, thanks to advancements in imaging technology which allow tailored treatment plans. However, it does have its drawbacks, notably the potential for side effects that can affect quality of life. Nonetheless, ongoing research aims to refine techniques, making this therapy safer and more effective.
Medical Imaging
In the medical field, imaging technologies such as positron emission tomography (PET) and magnetic resonance imaging (MRI) hinge on principles of particle physics. These modalities facilitate non-invasive examinations of the human body, allowing doctors to observe structures and functions in real time. This capability is vital for accurate diagnosis and treatment planning, essentially making it a pivotal aspect of modern healthcare.
The key characteristic of medical imaging is its non-invasive nature, which can greatly reduce patient discomfort and anxiety. However, the reliance on contrast agents and the need for costly equipment can pose challenges to accessibility in some regions. Despite these issues, the integration of advanced imaging techniques continues to illuminate the path toward more informed medical decisions.
Particle Therapy Techniques
Alongside traditional radiation therapy, particle therapy techniques, specifically proton and heavy ion therapies, are gaining traction. These methods utilize particles instead of conventional X-rays, allowing for greater precision in targeting tumors. The hallmark of particle therapy is its Bragg peak effect, where the majority of the energy is deposited directly at the tumor site, sparing surrounding healthy tissues even further.
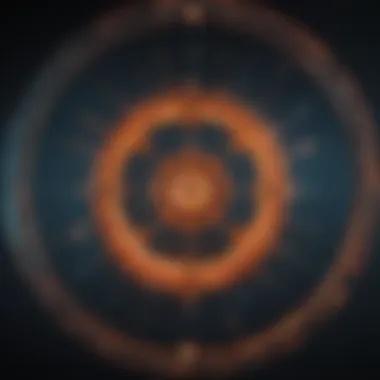
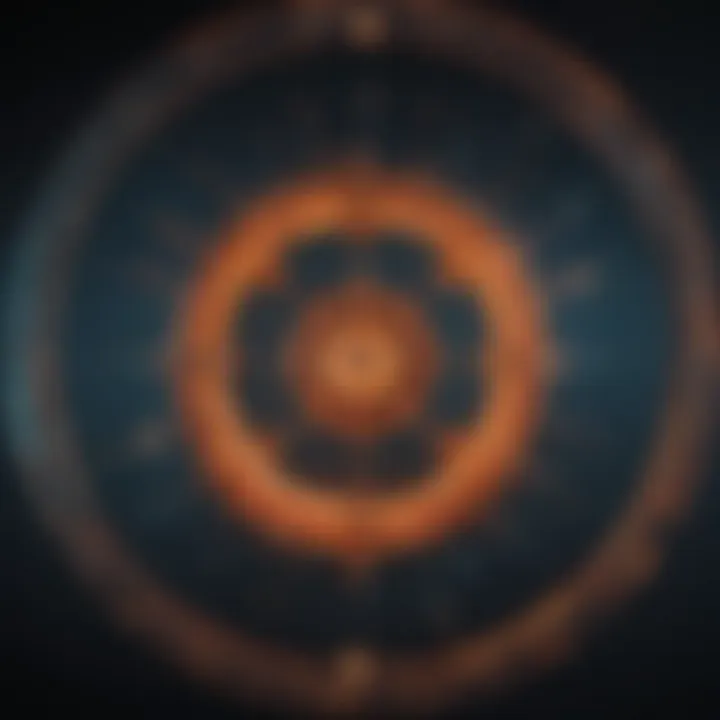
This unique approach can lead to fewer side effects and enhanced recovery for patients when compared to conventional therapies. However, the complexity and costs of establishing such facilities pose significant barriers to widespread adoption. Still, as technology advances, more healthcare systems are likely to consider this nuanced but impactful option.
Technology Advancements
Semiconductors
Semiconductors have revolutionized modern technology, acting as the backbone of electronic devices ranging from your smartphone to sophisticated computer systems. At a molecular level, semiconductors are materials that conduct electricity under certain conditions, making them ideal for integrated circuits. Their unique properties allow the manipulation of electrical signals, which is crucial for the performance of almost all digital devices.
This functionality stems from the properties of fundamental particles, such as electrons and holes, highlighting why an understanding of particle behavior is crucial in the tech industry. Advances in semiconductor technology continue to push the boundaries of miniaturization and energy efficiency, drastically improving the availability of cutting-edge technology for consumers.
Particle Accelerators
Particle accelerators are monumental devices utilized to propel charged particles to high velocities, enabling groundbreaking research in particle physics. These machines are not limited to laboratories; their prowess enhances numerous scientific disciplines, including high-energy physics, materials science, and even medicine. The Large Hadron Collider, for instance, has been instrumental in numerous discoveries, including the famous Higgs boson.
Their importance cannot be overstated; however, the costs and infrastructure required for such equipment can be daunting. Still, the knowledge gained from particle accelerator research has far-reaching implications beyond physics, shaping our understanding of the universe and even leading to technological innovations that permeate daily life.
Computational Simulations
As computational power increases, simulations are becoming indispensable in particle physics research. These programs allow scientists to model complex particle interactions that would be otherwise impossible to study directly. This aspect of particle physics eliminates the need for extensive physical trials and accelerates the pace of discovery.
Simulations are a vital tool, especially when dealing with particle data from detectors in experiments like those at CERN. However, there is a trade-off; inaccuracies in modeling can lead to misleading results. Despite these hurdles, the contribution of simulations to particle physics and other disciplines is irrefutable, driving forward our understanding of the fundamental laws governing the universe.
Cosmic Research
Particle Astrophysics
Particle astrophysics sits at the lucrative intersection of particle physics and astrophysics, studying cosmic phenomena and their implications on fundamental physics. This field examines rare particles from space, such as cosmic rays, which provide insights into the origins of the universe and fundamental forces. By detecting and analyzing these particles, scientists can gather data that would help decode the mysteries surrounding black holes, cosmic inflation, and more.
The many characteristics that particle astrophysics embraces make it a powerful domain in modern scientific inquiry. Yet the field has its challenges; understanding the conditions of the universe’s infancy remains a significant hurdle. Still, the pursuit of knowledge in this area continues to furnish humankind with crucial insights about our universe.
Understanding Dark Matter
Dark matter constitutes a significant portion of the universe, yet its nature remains one of the most perplexing mysteries of contemporary science. Researchers utilize particle physics principles to probe dark matter, searching for particles that could explain its elusive properties. Understanding dark matter could revolutionize our grasp of the cosmos and provide clarity on how these unseen entities influence galactic structures.
This investigation is crucial as dark matter impacts the entire universe's dynamics. The unique feature of studying dark matter is its ability to challenge our fundamental understanding of physics while prompting a re-evaluation of established theories. Yet, the indirect methods used pose difficulties, leading to ongoing debates within the scientific community.
Exploring the Early Universe
Delving into the conditions of the early universe gives researchers the chance to confront the core tenets of cosmology. By examining particles and their interactions during the initial moments post-Big Bang, scientists hope to unveil the processes that led to the formation of galaxies, stars, and the physical laws we observe today. This arduous endeavor blends particle physics with cosmology, offering a comprehensive view of existential questions.
One of the key characteristics of studying the early universe is its capacity to provide context for cosmic background radiation and cosmic inflation theories. However, the speculative nature of this research can sometimes lead to skepticism within scientific circles. Despite this, the drive to comprehend our origins continues unabated, motivating new generations of physicists to press onward in this fascinating quest.
Emerging Trends in Particle Research
Emerging trends in particle research are pivotal to advancements across various scientific fields. These trends not only shape the theoretical frameworks of particle physics, but also have practical implications in technology, healthcare, and even astronomy. As the scientific community delves deeper into the fundamental nature of matter, these developments could very well redefine our understanding of the universe and the particles that constitute it.
Advancements in Detection Techniques
Over recent years, the development of new detection techniques has been crucial in expanding our capabilities within particle research. The utilization of ultra-sensitive sensors has vastly improved our ability to detect elusive particles that are often hard to capture. For example, the advancements in photodetector technologies now allow researchers to measure faint signals from neutrinos. Neutrinos, being notoriously difficult to detect due to their weak interactions with matter, have given rise to innovative detection methods like liquid scintillation detectors and radio frequency arrays. These advancements energize the field, paving the way for breakthroughs in understanding the universe’s fundamental processes.
The Higgs Boson Discovery
The discovery of the Higgs boson in 2012 at CERN’s Large Hadron Collider revolutionized particle physics. However, the implications of this discovery stretch far beyond the event itself.
Implications of the Discovery
The Higgs boson plays an essential role in the Standard Model of particle physics, providing the mechanism by which particles acquire mass. The observation of this particle confirmed long-standing theories and validated decades of research. This discovery particularly shines a light on the symmetries and interactions that underlie particle physics, influencing ongoing and future research trajectories. Its significance does not merely reside in its existence; rather, it opens avenues for additional inquiries into beyond-the-Standard-Model physics, such as dark matter and supersymmetry. The implications are not just academic; they extend to practical fields, such as designing more effective particle accelerators.
Future Research Directions
Looking ahead, future research directions in particle physics are aimed at exploring phenomena that the Higgs boson implies. For starters, researchers are investigating how the Higgs field interacts with other particles, which could shed light on matter’s fundamental properties. The possibility of additional Higgs-like particles or sectors hints at richer physics beyond the Standard Model. This area of research is seen as particularly beneficial as it stimulates the development of more sophisticated collision experiments and novel theoretical frameworks, pushing the boundaries of our knowledge further.
Unanswered Questions
Despite the monumental discovery of the Higgs boson, numerous unanswered questions remain. Scientists are still grappling with issues such as the hierarchy problem, which questions why the Higgs boson mass is so light compared to the Planck mass. Also, the connection between the Higgs field and dark matter is far from clear. Such unanswered questions render the field ripe for exploration. Addressing these enigmas is not just an academic exercise; it invites a closer inspection of the very fabric of the universe, promising exhilarating discoveries in the years to come.
The Role of Artificial Intelligence
In recent times, artificial intelligence has become a game-changer in particle research, impacting how data is analyzed, simulations are enhanced, and patterns are recognized in physics.
Data Analysis
AI-driven algorithms have transformed data analysis processes within particle physics. The sheer volume of data generated by particle detectors poses challenges in extracting meaningful information. Machine learning models can sift through vast datasets to identify patterns that would otherwise go unnoticed. These advancements not only make it easier to draw insights from data but also streamline the tedious task of event classification. The potential for increased accuracy and efficiency positions AI as a valuable tool in advancing particle research.
Simulation Enhancements
Simulations in particle physics have benefitted greatly from AI techniques. Traditionally, simulating complex particle interactions is a computationally intensive endeavor. However, AI can optimize the simulation processes, predicting outcomes faster and with reduced resource requirements. Enhanced simulations make it feasible to experiment with countless scenarios and specifications, yielding a deeper understanding of particle behavior under varying conditions. The efficiencies gained from these enhancements significantly contribute to the ongoing research landscape.
Pattern Recognition in Physics
The ability of AI to recognize patterns within experimental data accelerates progress in theoretical and experimental physics. As researchers seek to find nuances in particle interactions, AI can highlight correlations and anomalies, guiding scientists toward new hypotheses and experiments. This capacity allows for a more dynamic, responsive research environment that adapts as new information surfaces. The synergy between AI and particle physics underscores a shift in research methodologies, one that embraces technological innovation to probe the depths of the subatomic realm.
Ending
As we wind down our examination of particles, it’s vital to acknowledge the significance of this topic in the realm of modern science. Particle physics doesn’t merely represent a niche discipline; it serves as the bedrock upon which much of contemporary understanding is built. The interactions and behaviors of these imperceptible fragments of matter hold keys to answers that have eluded scientists for centuries, from the origins of the universe to the fundamental forces that shape our reality.
Summary of Key Insights
Throughout this discourse, we’ve traversed a landscape rich with various particle types, each playing unique roles in the fabric of the cosmos. The fundamental particles—quarks and leptons—lay the groundwork for the more complex composite structures such as baryons and mesons. Specifically:
- Quarks, which combine to form protons and neutrons, are the building blocks of atomic nuclei. Their confinement within hadrons challenges our comprehension of individual particle behavior.
- Leptons, on the other hand, exist independently and are essential in processes like beta decay, connecting closely with the weak nuclear force.
- Gauge bosons facilitate the interactions between these particles, each carrying the fundamental forces that guide their behaviors.
- Moving beyond the basics, the study of composite particles like baryons and mesons opens numerous doors to understanding strong interactions and the dynamics of atomic nuclei.
"To unravel the mysteries of particles is to unlock the secrets of the universe itself."
Future Outlook for Particle Physics
The road ahead for particle physics is paved with both challenges and opportunities. Researchers are venturing into uncharted territories, employing cutting-edge technologies and methodologies, buoyed by the insights gained from landmark discoveries such as the Higgs boson. Future directions may include:
- Advanced Detection Techniques: Improvements in detectors and methodologies will enhance our ability to explore elusive particles like neutrinos or investigate dark matter candidates.
- Artificial Intelligence: Leveraging AI in data analysis will streamline the massive datasets produced by particle experiments, allowing for rapid identification of valuable patterns and anomalies.
- New Theoretical Models: As our experimental findings evolve, so too must our theoretical frameworks, giving rise to new models that could encompass phenomena not yet understood.
In sum, the future of particle physics holds immense potential. As the boundary of knowledge expands, each discovery has the power to reshape our understanding of the universe—one particle at a time.