Navigating the Future of Qubit Computing
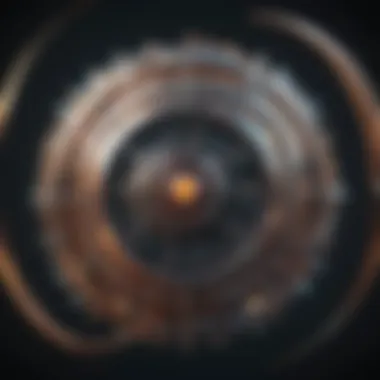
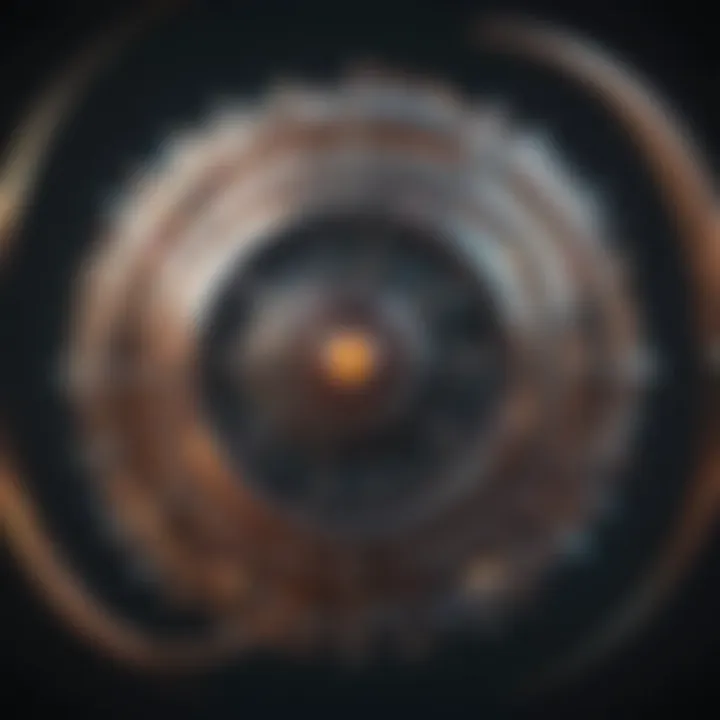
Key Research Findings
Overview of Recent Discoveries
In recent years, the field of qubit computing has seen an explosion of interest and experimentation. Researchers have published numerous studies that reveal the potential and challenges of quantum bits, which serve as the cornerstone for quantum computing. One key finding includes the development of superconducting qubits, which have shown great promise due to their scalability and coherence time, allowing for persistent quantum states that are crucial in computations.
Another significant discovery backs the validation of error-correction codes tailored for qubit systems. Traditional error correction methods fall short in quantum environments, where the principles of superposition and entanglement complicate matters. New approaches such as surface codes have emerged, offering a way to manage and mitigate errors efficiently. This is significant since error rates can be the Achilles heel of any quantum computing system.
Moreover, engineers and physicists have revealed how qubits can exhibit behavior that defies classical intuition. For instance, through a process called entanglement swapping, qubits can share information without a direct connection. These findings not only foreshadow new computational capabilities but also illustrate the dramatic divergence between classical and quantum systems.
Significance of Findings in the Field
The implications of these discoveries are far-reaching. Qubit computing is primed to revolutionize various industries, from cryptography to materials science. The ability to perform computations that were previously deemed impossible, such as factoring large integers in a fraction of the time, raises questions about current encryption methods. Additionally, advancements in quantum machine learning are paving the way for algorithms that can learn from vast datasets much quicker than their classical counterparts.
This transformation could redefine how businesses manage data, interact with artificial intelligence, and develop new products. As researchers continue to explore and refine qibt technologies, the importance of investing and prioritizing quantum research becomes unmistakable. Findings are not merely academic; they are stepping stones toward practical applications that may soon alter the technological landscape.
Breakdown of Complex Concepts
Simplification of Advanced Theories
Understanding the intricacies of qubit computing can be bewildering. However, by breaking down advanced theories, one can recognize the foundations that support this burgeoning field. At its core, a qubit can represent more than just a zero or a one. Unlike classical bits, qubits can exist in multiple states at once due to a principle known as superposition. This allows them to perform many calculations simultaneously, a feat classical computers cannot match.
Additionally, concepts such as quantum entanglement and quantum tunneling contribute to the potency of qubit computing. Entanglement, often described as a magical connection between particles, allows qubits separated by vast distances to instantaneously affect one another. As a result, qubit systems can exchange information rapidly, transcending conventional geographic and temporal limits.
Visual Aids and Infographics
Visual aids and infographics serve as excellent tools for demystifying qubit computing. For example, consider a diagram that illustrates the difference between classical bits and qubits, highlighting how qubits can occupy multiple states. Such illustrations can clarify these abstract notions and anchor them in a more digestible visual format. In the context of error correction, infographics that visualize surface codes or the qubit error correction process could also enhance understanding, showcasing how these methods protect quantum states and maintain computation integrity.
"In the quantum realm, certainty is a mirage, and probabilities are the gold standard. This nuanced behavior yields a wealth of opportunities, albeit with significant challenges."
By engaging with these more accessible representations, both newcomers and seasoned experts can foster a deeper comprehension of the landscape of qubit computing, setting the stage for further exploration and innovation in quantum technologies.
Preamble to Qubit Computing
In todayβs fast-evolving technological landscape, the emergence of qubit computing represents not just a shift but a leap into a new paradigm of processing information. As we grapple with the limits of classical computers, the significance of understanding qubits becomes crystal clear. Qubits, or quantum bits, serve as the building blocks of quantum computing, and they hold the promise to tackle problems that are infeasible for traditional systems. This section aims to outline the fundamental importance of qubit computing, its key elements, and the benefits it brings.
Defining Qubits
At its core, a qubit can be understood as the quantum analogue of a classical bit, which is the simplest unit of information in classical computing. However, unlike classical bits that hold values of either 0 or 1, qubits can exist simultaneously in both states, a phenomenon known as superposition. This ability to be in multiple states at once is what equips quantum computers with their astonishing potential to perform complex calculations vastly more quickly than their classical counterparts.
For instance, consider a qubit system used for search algorithms. While a classical computer might sequentially search through data, a quantum computer can analyze multiple possibilities at once due to the unique properties of qubits. This is not just a theoretical concept: practical implications abound in fields such as cryptography, material science, and artificial intelligence, where the ability to process vast amounts of information simultaneously can lead to groundbreaking advancements.
Historical Context of Quantum Computing
To appreciate the current state of qubit computing, it is essential to delve into its historical context. Quantum computing has roots that trace back to the early 1980s when physicist Richard Feynman proposed that quantum systems could simulate other quantum systems far more efficiently than classical computers. This sparked a wave of interest that ignited the quest to harness quantum mechanics for computation.
Over the decades, the theoretical groundwork laid by pioneers such as Lov Grover and Peter Shor laid the foundation for algorithms that would demonstrate the potential of quantum speed-ups. Shor's algorithm, capable of factoring large integers exponentially faster than any classical algorithm, serves as a critical milestone, showcasing the promise of quantum computing to revolutionize areas such as cryptography.
"Quantum mechanics is one of the most successful theories in physics, and its principles have begun to fuel the future of computing."
While significant strides have been made since those early days, including advancements in qubit design and error correction, challenges remain. The journey toward practical quantum computing continues to be paved with both excitement and caution, as researchers and engineers work tirelessly to unlock the full potential of qubits. Understanding the historical developments that have shaped this field enhances our appreciation for its current and future significance.
In summary, this introduction encapsulates the essence of qubit computing, emphasizing the transformative potential of qubits and their foundational role in the evolution of computational capabilities. Their unique properties not only highlight the departure from classical computing but also chart a course toward unprecedented possibilities in technology and science.
The Fundamentals of Quantum Mechanics
Understanding quantum mechanics is key to grasping the broader implications of qubit computing. This section breaks down the essential principles that stand in stark contrast to the conventional computing paradigm, laying the groundwork for future discussions about qubits and their applications. At its core, quantum mechanics deals with the behavior of matter and energy at microscopic scalesβan arena where classical physics simply falls short. Without delving into these fundamentals, one might find the nuances of qubit operation bewildering. The importance of this section lies in establishing a solid foundation, as it paves the way for a deeper exploration of quantum information and qubit technology.
Classical vs. Quantum Information
The juxtaposition of classical information against quantum information sets the stage for a fascinating discussion. Classical information, which comprises bits, operates strictly in a binary formatβwhere each bit is either a 0 or a 1. This simplicity is effective for many applications but inherently limited when it comes to processing complex tasks. Think of classical bits as a single light switch that can be either on or off.
In stark contrast, quantum information utilizes qubits, significantly transforming how data can be represented and manipulated. A qubit can represent numerous states simultaneously due to superposition. To illustrate:
- A qubit can exist in the state of 0,
- It can be in the state of 1,
- Or it can be in a blend of both.
This capability allows quantum computers to perform many calculations at once, making them potentially exponentially faster than classical computers when tackling specific problems. For instance, while a classical computer might solve problems sequentially, a quantum computer can explore multiple pathways simultaneously, unlocking a new realm of computational power.
Furthermore, classical data is susceptible to straightforward copying, while quantum data entwines itself into the very fabric of quantum states through entanglement. This leads to an entirely new perspective on data security and confidentiality, as monitoring a quantum system can potentially alter its state, thus providing intrinsic protection against eavesdropping.
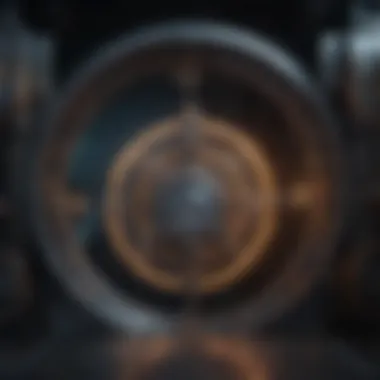
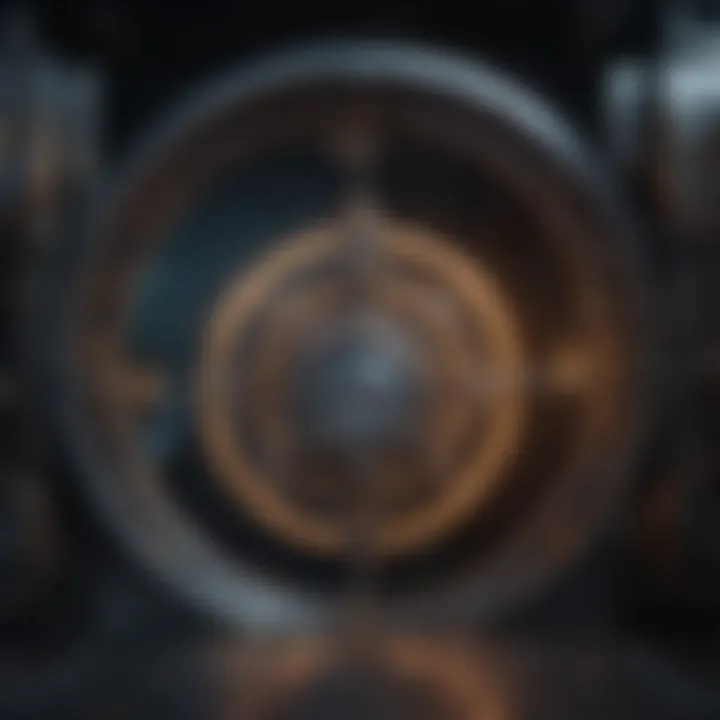
The nuances of how these two forms of information operate reveal not just differences in processing but fundamental shifts in capabilities, influencing sectors such as cryptography, optimization, and simulation. Understanding this dichotomy enhances our appreciation for the profound advancements qubit computing promises to deliver.
Principles of Superposition and Entanglement
Two foundational concepts in quantum mechanics are superposition and entanglement, both pivotal to the functionality of qubits.
Superposition refers to the ability of a quantum system to be in multiple states at once. Imagine casting a stone into a pond, sending forth ripples in all directions. Similarly, when a qubit is in a state of superposition, it can simultaneously represent 0 and 1. This ability enables quantum computers to perform extensive computations in parallel, drastically reducing the time required to solve complex problems compared to classical systems.
"Superposition is not just a theoretical concept; it's a game-changer in how we think about information processing."
On the other hand, entanglement is an even more astonishing phenomenon. When qubits become entangled, the state of one qubit becomes directly related to the state of another, regardless of the distance separating them. Picture two coins flipped far apart yet still revealing the same face at the same moment. This interdependence enables powerful correlations that can enhance computational efficiency and facilitate secure communications.
Entangled qubits react to each other's changes instantaneously, raising questions about locality and information transfer across distances. This peculiarity is at the heart of quantum teleportation and quantum cryptography, showcasing how quantum mechanics can transform secure communication methods.
The principles of superposition and entanglement not only define how qubits operate but also lay the ground for innovative financial modeling, complex systems simulation, and artificial intelligence advancements.
In summary, comprehending the fundamentals of quantum mechanics provides the necessary insights to appreciate qubit technologies. Recognizing how quantum information differs from classical approaches is crucial, particularly when discussing practical applications and future advancements in qubit computing.
Qubit Technologies
Qubit technologies form the bedrock of quantum computing, providing the framework needed for harnessing the power of quantum bits. The significance of this section in the broader context of the article cannot be overstated. Understanding how qubits function paves the way for appreciating the complexities involved in their implementation and usage. Qubits, while simplistic in definition, do exhibit profound intricacies that make their technologies vital for any ambitious quantum computing endeavor.
Physical Implementations of Qubits
The physical realization of qubits is done through various technologies, each presenting unique advantages and challenges. By exploring the different methods of implementing physical qubits, we gain insight into their operational nuances, which can influence the direction of quantum computing research and applications.
Superconducting Qubits
Superconducting qubits are perhaps one of the most prominent implementations in the field of quantum computing. These qubits are based on superconducting circuits, typically made from materials like niobium or aluminum, and are cooled to extremely low temperatures to enable superconductivity. A key characteristic of superconducting qubits is their relatively fast processing speed.
- They can achieve coherence times on the order of tens to hundreds of microseconds, which is significant for operations.
The unique feature that stands out is their ability to utilize microwave photons for quantum state manipulation, offering rapid gate operations. This speed is what makes superconducting qubits a popular choice among leading companies and research institutions.
However, they do come with their drawbacks. The cooling requirements can be demanding and expensive, and the coherence times can still be shorter compared to other qubit types. It can also be tricky to scale up the number of qubits without running into issues of increased noise and complexity.
Trapped Ion Qubits
Trapped ion qubits are another leading technology, focusing on ions confined using electromagnetic fields. This method operates at room temperature, which inherently simplifies some of the hardware requirements seen in other types of qubits. A strong point of trapped ion qubits is their exceptionally long coherence times, often reaching minutes, allowing for more complex calculations within the same quantum state.
- The precision of entangling operations via laser beams is one of the marked advantages, as it opens pathways for performing high-fidelity gate operations.
The unique aspect of this technology lies in its scalability. As researchers develop better techniques for optimizing control over the laser systems, the potential for increasing the number of qubits continues to rise. Yet, it is necessary to keep in mind that ion trap systems are also relatively slow when it comes to performing operations compared to superconducting qubits, making the trade-off between speed and fidelity a crucial consideration.
Topological Qubits
Topological qubits represent a theoretical breakthrough in the quest for robust quantum computing. They utilize the properties of particle-like excitations in two-dimensional materials, which are designed to protect quantum information from decoherence. This inherent resistance to errors presents a key characteristic of topological qubits, making them particularly intriguing for future applications.
- A notable benefit of topological qubits is their potential for fault tolerance. Because they are less sensitive to local disturbances, they can theoretically maintain coherence over longer periods, which is significant for large-scale quantum computation.
The concept of braiding is a unique feature, allowing operations to be executed by manipulating the spatial positions of these qubits instead of directly altering their states. While this technology holds promise, it remains largely experimental, with many engineering challenges still to be addressed before practical application.
Decoherence and Its Challenges
Decoherence poses immense challenges in maintaining the integrity of quantum data. It arises from interactions between qubits and their environments, leading to the loss of information. Managing decoherence through various error correction techniques is vital for the success of any qubit technology. Understanding the nuances of decoherence and its implications is a critical consideration within the landscape of quantum computing.
Quantum Algorithms
Importance of Quantum Algorithms
Quantum algorithms represent the beating heart of quantum computing, illustrating how quantum systems can solve problems in ways that classical digital computers cannot even begin to touch. The significance of these algorithms extends far beyond mere speed; they offer new methodologies for tackling complex challenges in various fields, such as cryptography, optimization, and artificial intelligence. By leveraging qubits' unique properties, specifically superposition and entanglement, quantum algorithms can process vast amounts of data simultaneously, positioning them as transformative tools in both academia and industry.
The benefits of quantum algorithms are hard to overlook. They can potentially reduce the time it takes to solve problems that currently take traditional computers years or even decades. Consider an example from the world of cryptography: Shor's algorithm can factor large integers exponentially faster than the best-known algorithms for classical computers. This poses a significant challenge for current encryption techniques reliant on the difficulty of factorization, hinting at a future where our understanding of security might need a substantial refresh.
Beyond just performance, the implications are enormous. Quantum algorithms can redefine what it means to process information, opening doors to innovative solutions that can address pressing global challenges. As researchers continue to explore these algorithms, the ongoing development of quantum systems holds the promise of a new era of computational power and efficiency.
Shorβs Algorithm
Shorβs algorithm is often heralded as a milestone in quantum computing. Developed by mathematician Peter Shor in 1994, this algorithm efficiently factors large integers. To grasp its importance, it's imperative to appreciate the implications it has on modern cryptography. The RSA encryption system, widely used for secure online communications, relies on the principle that factoring very large numbers is computationally intensive. However, Shorβs algorithm effectively shatters that assumption by introducing a polynomial-time solution to the problem.
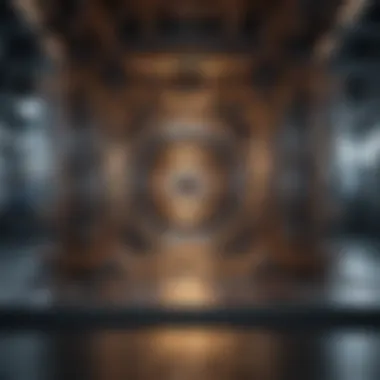
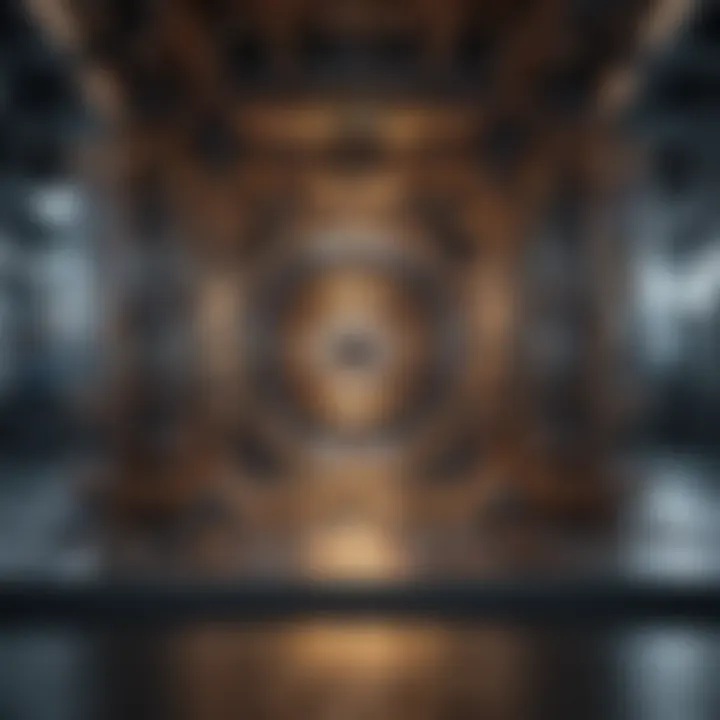
The mechanics behind Shorβs algorithm capitalize on quantum parallelism, where multiple computations occur at once thanks to superposition. This is akin to having a multitude of workers tackling facets of the problem in harmony, rather than one worker plodding through the tasks sequentially. Ultimately, the revelation that quantum computers could feasibly break certain encryption standards has led to an ongoing discussion about the future landscape of cybersecurity, pushing the development of quantum-resistant cryptographic systems to the forefront.
Groverβs Algorithm
Groverβs algorithm, introduced by Lov Grover in 1996, takes on a different but equally significant role in the quantum computing ecosystem. Unlike Shorβs algorithm, which targets factorization, Groverβs algorithm provides a way to search through unsorted databases more efficiently than any classical algorithm can. Specifically, it allows for searching a database of N items in only about (O(\sqrtN)) operations, compared to the (O(N)) operations required with classical methods.
This efficiency emerges from a prime property of quantum mechanics, using quantum superposition and interference to amplify the probability of finding the desired outcome while simultaneously reducing that of the unwanted ones. Groverβs algorithm can be applied in a range of scenarios, from optimization problems to cryptographic search functions. Its key advantage lies in its versatility, making it valuable not just for theoretical pursuits but also practical applications across various industries, including finance and logistics.
"Quantum algorithms like Shor's and Grover's don't just promise faster calculations; they signify a paradigm shift in solving problems that have remained intractable for classical methods."
The ongoing research in quantum algorithms underlines the dynamic nature of this field, as researchers constantly seek to develop new algorithms and refine existing ones, pushing the boundaries of what quantum computing can achieve.
Understanding these algorithms is not an academic exercise; it holds real-world consequences. Enterprises and governments alike are gearing up for a future where quantum computing offers solutions that are not just faster, but also smarter, more intricate, and infinitely more capable than anything we have today.
Applications of Qubit Computing
Exploring the applications of qubit computing unearths the unique capabilities that quantum bits possess compared to their classical counterparts. Quantum computing holds potential that can revolutionize various sectors, driving advancements across industries. From cracking complex encryption systems to enabling drug discovery processes at unprecedented speeds, the implications are vast. This segment will delve into specific applications, showcasing their significance and the transformative benefits they bring.
Cryptography
In the realm of cryptography, the power of qubit computing extends far beyond traditional methods. Classical systems rely on algorithms that take considerable time to crack cryptographic codes. In contrast, quantum algorithms, particularly Shor's algorithm, enable the pinpointing of prime factors exponentially faster than any classical approach.
Imagine a world where the most secure digital communication could potentially become vulnerable due to advances in quantum computing. This reality necessitates the development of quantum-resistant encryption protocols to safeguard sensitive data. By integrating qubits into cryptographic systems, we not only bolster security but also prepare for a future where quantum threats loom large.
"The rise of qubit computing introduces uncertainties but also greater security paradigms in the world of digital protection."
Drug Discovery
Drug discovery represents another promising application of qubit computing. The traditional methods of testing chemical compounds are often time-intensive and costly. Quantum computing can simulate molecular structures and interactions efficiently, allowing researchers to predict how new drugs will behave in the body before moving to lab tests.
This approach accelerates the discovery process significantly. Consider the development of a life-saving medication. A quantum system can run simulations to identify viable compounds much faster than classical methods, thus reducing the overall timeline and cost of bringing effective treatments to patients. This not only enhances the efficiency of the process but also opens opportunities for tackling diseases that have resisted conventional medicinal advancements.
Material Science
In material science, qubit computing offers a fresh perspective on material design and analysis. Researchers can leverage quantum algorithms to model complex systems at atomic levels, examining properties and behaviors of materials that were previously too challenging to analyze with classical computing power.
The enhanced precision and speed could lead to breakthroughs in creating stronger, lighter materials or even superconductors operating at room temperatures. For instance, imagine developing a super-efficient battery or a revolutionary new material for electronics that drastically reduces energy consumption. As we step into the quantum era, the possibilities for advancing material science become increasingly exciting and essential in addressing modern-day challenges.
In summary, the applications of qubit computing extend far and wide, with cryptography, drug discovery, and material science being just the tip of the iceberg. By harnessing the unique properties of quantum bits, industries can pursue innovations that were once beyond reach, ultimately driving progress in critical fields.
Challenges in Qubit Computing
The endeavor of qubit computing holds immense promise, yet it also presents a myriad of formidable challenges. Understanding these challenges is crucial for not just enthusiasts but also for the broader audience interested in the future of technology. The section will dissect key elements like error correction and scalability, offering insights that illuminate why overcoming these hurdles is pivotal for the evolution of quantum systems.
Error Correction
In the quantum realm, the issue of error correction is a significant concern. Unlike classical bits, which are less prone to errors during computations, qubits are much more susceptible to various types of noise and interference. This vulnerability arises mainly from the delicate state of qubits, which can easily be disrupted by their environment. The effects of decoherence and quantum noise can wreak havoc on computations, making error correction a critical aspect of qubit technology.
Successful error correction in quantum computing necessitates sophisticated algorithms and complex architectures. One popular approach is the implementation of quantum error-correcting codes, such as the Shor code or the surface code. These codes function by encoding logical qubits into multiple physical qubits, effectively allowing the system to detect and correct errors without measuring the qubits directly, which could otherwise collapse their quantum state.
"Quantum error correction requires a meticulous balance of redundancy and efficiency, ensuring that errors are managed without bogging down the computational process."
Despite advancements, the process of error correction remains resource-intensive. It necessitates a substantial overhead in terms of the number of qubits requiredβoften requiring many more physical qubits to create a single fault-tolerant logical qubit. This leads us to another pressing challenge: scalability.
Scalability Issues
Scalability in qubit computing pertains to the ability to increase the number of qubits while maintaining performance and reliability. Currently, the construction and management of large-scale quantum systems can be compared to herding catsβboth complex and prone to chaos. The physical limitations of existing qubit technologies pose significant obstacles. For example, superconducting qubits require intricate cooling systems to achieve operational temperatures near absolute zero. Similarly, trapped ion qubits rely on precise laser controls, which can be tricky to scale effectively.
Moreover, when trying to forge larger quantum circuits, maintaining the coherence time of qubits becomes an arduous task. As the number of qubits increases, managing entanglement across all qubits can lead to diminishing performance due to increased susceptibility to errors. Strategies like modular quantum computing, where smaller quantum systems are linked together, are gaining traction. However, the technology for interconnecting qubit modules efficiently remains a challenge that must be addressed.
Comparative Analysis: Quantum vs. Classical Computing
In an era where technology grows at an exponential rate, it's crucial to understand how quantum computing, particularly through the lens of qubit systems, stands apart from traditional classical computing. This comparison not only sheds light on the fundamental distinctions in how these computing paradigms operate but also highlights the vast potential that quantum computing holds in reshaping various industries. Understanding these differences is essential for students, researchers, educators, and professionals alike as it informs current and future technological trends.
Performance Metrics
When comparing quantum and classical computing, performance metrics are pivotal. These metrics often include speed, efficiency, and computational capability. Classical computers rely on bitsβzeros and onesβto process information, whereas quantum systems utilize qubits, which can represent and compute multiple states simultaneously thanks to principles like superposition.
- Speed: Quantum computers excel in specific tasks. For instance, problems that involve large datasets or complex variable interactions can be solved much faster with quantum algorithms, like Shor's or Grover's, than any classical counterpart. A primary example is factoring large integers, which is notoriously time-consuming for classical systems.
- Efficiency: Quantum computing's ability to perform parallel computations means they often require fewer computational steps for certain algorithms. This leads to better resource management, enabling tasks that would take classical systems years to execute to be completed in mere minutes.
- Computational Capability: Classical computing has limitations when dealing with phenomena that require capturing a multitude of states and their interactions. Quantum computers can model systems such as molecules for drug discovery more comprehensively due to their capacity to handle the complexities of quantum mechanics.
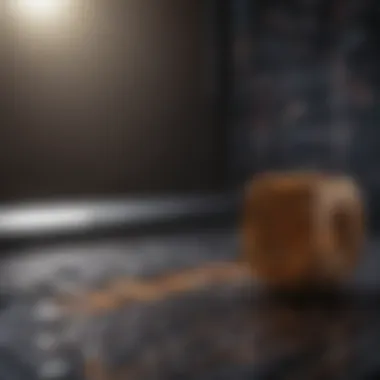
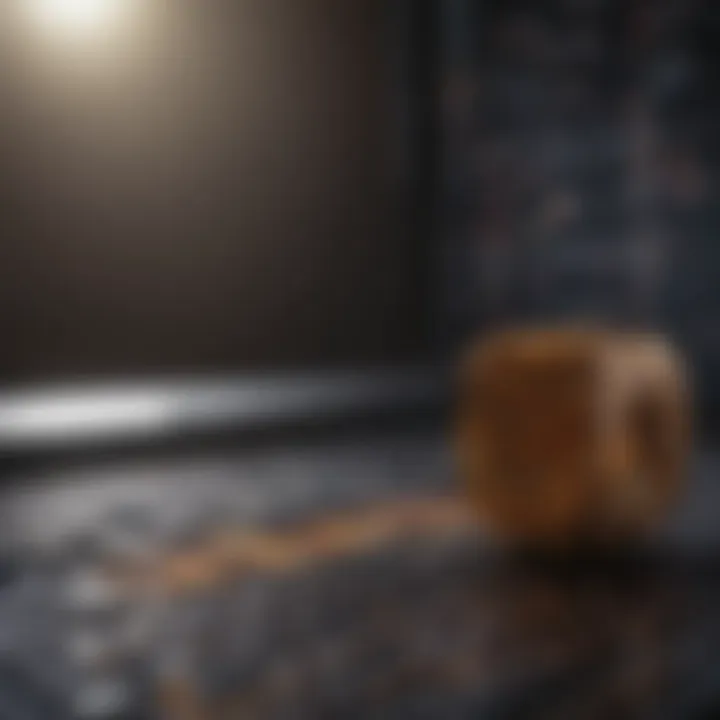
"The operational framework of quantum computing is not just a mere enhancement over classical computing; it represents an entirely different approach to problem-solving."
Use Cases and Limitations
The exploration of use cases highlights the transitions between classical and quantum applications. Despite the considerable promise qubit computing holds, itβs vital to recognize its limitations.
Use Cases
Quantum computing finds application in diverse fields, such as:
- Cryptography: The potential for quantum key distribution offers fundamentally secure communications, enhancing privacy.
- Drug Discovery: Quantum simulations allow scientists to predict molecular behaviors, streamlining the discovery process of new drugs.
- Optimization Problems: Fields like logistics and supply chain management stand to benefit from enhanced optimization algorithms that quantum computing can provide.
Limitations
However, itβs equally important to note the current hurdles:
- Error Rates: Quantum systems are susceptible to errors due to decoherence and noise, leading to questions about reliability in critical applications.
- Scalability: Building large-scale quantum systems is challenging. Many current experiments demonstrate potential, yet scaling these systems for practical use is still an open question.
- Resource Requirements: Quantum computing requires specialized equipment and environments, which may hinder broader implementation.
The Future of Qubit Computing
The realm of qubit computing holds significant promise for driving forward technological advancements and reshaping our understanding of computation. As industries and academic institutions lean into this frontier, it's crucial to dissect its future, particularly focusing on how emerging technologies and interdisciplinary collaborations will experience the maturation of quantum computing capabilities.
Emerging Technologies
Qubit computing is set to usher in an array of groundbreaking technologies that will fundamentally alter computing paradigms. Among these technologies are quantum processors, which are advancing at an impressive pace. These processors leverage various qubit implementations, like superconducting or trapped ion qubits, to achieve unprecedented computational speeds.
- Quantum Supremacy: This term refers to the moment when a quantum computer can solve problems deemed unsolvable by classical computers in a practical timeframe. Although Google claimed to have achieved this milestone in 2019, further advancements are anticipated as more refined algorithms and improved qubit stability come into play.
- Hybrid Quantum-Classical Models: The future may see an integration of quantum capabilities within classical computers, creating hybrid systems. This approach can optimize processes across various sectors, such as optimization problems in logistics or finance.
- Quantum Networking: A robust future in qubit computing will also include enhancements in quantum networking. This technology lays the groundwork for secure communications through quantum encryption methods, derived from the principles of quantum mechanics.
These emerging technologies don't just hold academic interest; their implications are vast. From revolutionizing data analysis to reshaping artificial intelligence, the ripple effects are likely to touch every facet of modern life.
Interdisciplinary Collaborations
The growth of qubit computing isn't a solo journey; it's increasingly reliant on collaborations across disciplines. The challenges of developing effective quantum algorithms or efficient qubit architectures can't be placed at the feet of computer scientists alone.
- Synergy with Physics: Quantum computing fundamentally emerges from principles of quantum mechanics. Physicists are key players in advancing our understanding of qubit behaviors and the underlying phenomena that enable their operation and error reduction.
- Partnerships with Other Sciences: Fields like chemistry and material sciences are exploring applications of qubit technologies for complex simulations. For instance, drug discovery can benefit from quantum simulations of molecular interactions that classical computers can't efficiently model.
- Focus on Ethics and Governance: As the landscape shifts, considerations about ethical implications and governance must arise. Collaborations with social scientists and ethicists can ensure that advancements in quantum computation serve the broader public interest, promoting a responsible deployments of these technologies.
"Interdisciplinary collaborations are the bedrock upon which the future of qubit computing stands: complexity requires diversity of thought."
In summary, the future of qubit computing is not just about making faster computers; it's about evolving an ecosystem where diverse disciplines converge to birth innovations that could drastically change how we understand and approach technology. As we stand at this juncture, the potential for growth and transformation is boundless.
Ethical Considerations in Quantum Computing
The development of quantum computing technology presents numerous ethical considerations that must not be overlooked. As qubit computing emerges as a powerful tool, its potential applications and implications can affect individual privacy rights, security protocols, and societal structures as a whole. Understanding these considerations is crucial, not just for technologists but also for policymakers, educators, and the general public.
Impacts on Privacy and Security
Quantum Computing holds the cards to unlock vast amounts of data, raising concerns about privacy erosion in multiple contexts. For instance, algorithms designed for qubit systems could potentially crack encryption methods that currently protect sensitive information. This means managing personal data could get complicated. Regular cybersecurity measures may not cut it when quantum computers become widely accessible.
- Shorβs Algorithm, which can factor integers exponentially faster than classical algorithms, poses a direct threat to conventional encryption. As a result, personal identification information, financial details, and confidential communications risk exposure.
- The banking industry, for example, might need to rethink how it secures transactions. Quantum systems could read encrypted transactions if they aren't prepared or resilient enough.
- The advent of post-quantum cryptography is one possible solution, but it's still an evolving field.
"The arrival of quantum technologies necessitates a paradigm shift in our approach to digital security. We cannot afford to sit on our hands while the potential threats loom over us."
As organizations and governments strive to implement protective measures, the balance between technological advancement and ethical responsibility will be tested.
Societal Implications
The ramifications of quantum computing extend beyond the realm of data security. Ethical considerations touch upon how society interfaces with this powerful technology. Several issues arise when assessing these societal implications:
- Economic Disparities: Not everyone will have equal access to quantum technologies. Those with resources may leverage these advancements to create substantial competitive advantages. This could widen the gap between tech-savvy enterprises and those struggling to keep pace.
- Job Displacement: Automation thrives on advancements in computing. Quantum computing could redefine industries by making certain job roles obsolete. Workforce readiness should be anticipated, fostering an environment where individuals can reskill and adapt.
- Political Dynamics: Control over quantum technology can potentially empower certain governments while undermining others, breeding geopolitical tension. Questions about surveillance, data ownership, and ethical governance in a quantum world should lead to critical discussions.
In closing, the ethical landscape surrounding quantum computing is both complex and compelling. As significant decisions are made regarding this technology's development and deployment, we must navigate potential pitfalls with an informed approach, encouraging dialogue among stakeholders in various fields. This mindfulness can help foster a future where innovation and ethical responsibility go hand in hand.
Closure
In the landscape of modern computation, the realm of qubit computing stands out as a beacon of innovation and transformation. The conclusion of this article sheds light on the pivotal significance of understanding qubits and their implications for various sectors of society. It synthesizes the myriad elements discussed throughout the sections, reinforcing key takeaways and exploring benefits that quantum computing promises.
Summarizing Key Insights
To distill the essence of our exploration, several key insights emerge. First, the distinction between classical and quantum computing hinges on how information is processed and stored. Classical bits, the bedrock of traditional computing, represent either a 0 or a 1, while qubits can exist in various states simultaneously, thanks to the principles of superposition. This characteristic enables quantum computers to tackle complex problems at speeds unattainable by their classical counterparts.
Moreover, the applications of quantum computing span diverse fields such as cryptography, drug discovery, and materials science. The inherent capability of qubits to perform parallel computations opens up new frontiers in optimizing solutions to real-world problems. Understanding these insights not only enlightens students and educators but also empowers researchers and professionals aiming to push the envelope in technological advancement.
"Quantum computing is a game changer. Its potential to redefine how we approach complex computations cannot be overstated."
Final Thoughts on the Evolution of Computation
As we stand on the precipice of a new computational era, it is crucial to grasp that the evolution of technology is more than a mere advance in speed. It embodies a fundamental shift in how we interpret and interact with information itself. Quantum computing symbolizes this shift, where possibilities stretch beyond traditional paradigms.
However, it's important to acknowledge the challenges ahead. Issues such as error correction, decoherence, and scaling up quantum systems remain at the forefront of research. Yet, the interdisciplinary efforts pooling insights from various domains offer promising avenues for overcoming these hurdles. As we forge ahead, the future of qubit computing shines brightly, promising an exciting journey into uncharted territories of knowledge and technology that will reshape societal landscapes.
Ultimately, as students, researchers, and professionals delve deeper into the world of qubit computing, they not only grasp its potential but also contribute to a broader understanding of its transformative capabilities. The journey of qubit computing is just beginning, and the implications of its applications are set to redefine not only computation but the very framework of innovation.