Understanding the Foundations of Quantum Physics
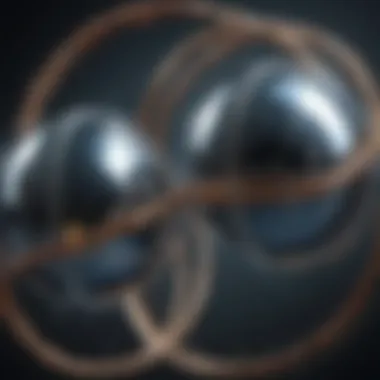
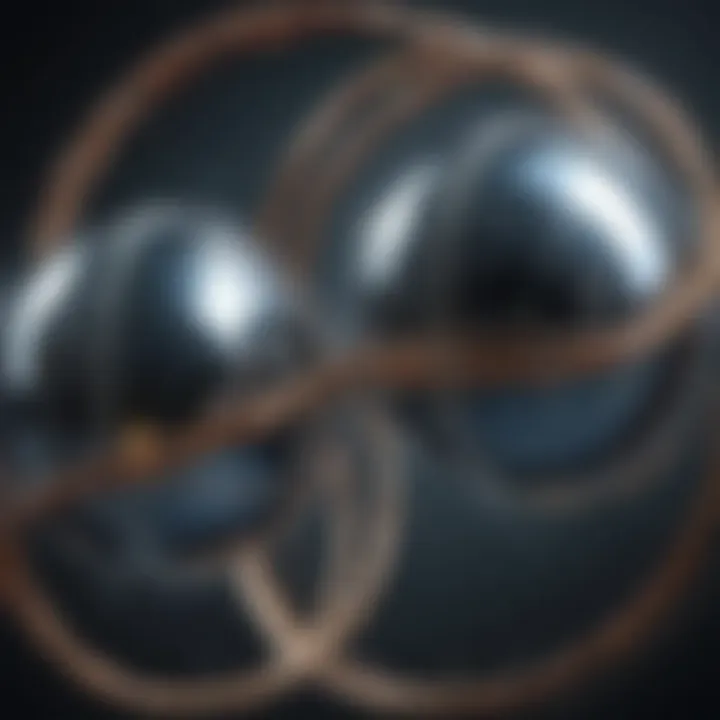
Intro
Quantum physics might sound like the kind of thing that’s reserved for sci-fi novels or high-end research labs, but its principles are deeply woven into the fabric of modern reality. From the moment you wake up and turn on your coffee maker, to the phone you check for messages, quantum mechanics plays a pivotal role. Yet, for many, its complexities remain an enigma, shrouded in jargons like entanglement or superposition.
This exploration aims to demystify those terms as we peel back the layers of quantum physics, laying out a roadmap to understanding. With recent advances reshaping not only theoretical science but practical applications in technology, we stand at the frontier of a quantum revolution. Whether you are a student, a researcher, or simply a curious mind, this guide will walk you through the key findings, complex concepts, and their far-reaching implications.
When delving into quantum physics, it’s essential to grasp the various components that come together to create its intricate tapestry. The upcoming sections will unravel everything from the foundational principles that serve as the bedrock of quantum mechanics, to the mind-bending scenarios that can make one question the very nature of reality.
In the following sections, we will examine recent discoveries in the field and highlight their significance. We will simplify advanced theories, allowing everyone, regardless of background, to grasp these fascinating ideas. So buckle up, as we navigate through the peculiarities of the quantum realm.
Preface to Quantum Physics
Quantum physics, in its essence, delves into the behavior of matter and energy at the smallest scales. This fascinating field stands pivotal in modern science, paving the way for technological innovations and reshaping our understanding of the physical world.
The importance of introducing quantum physics lies in its capacity to challenge our classical perceptions of reality. Understanding how particles interact, travel, and exist simultaneously in multiple states uncovers a realm where traditional rules no longer apply. This journey into the quantum world not only enhances scientific knowledge but also unlocks the door for practical applications that influence everyday life.
Historical Context
Early Discoveries
Early discoveries in quantum theory carried tremendous weight. Pioneers like Max Planck and Niels Bohr set the stage for what would become a revolutionary shift in physics. Planck's introduction to quantization of energy in 1900 was a catalyst, prompting further exploration and challenge to classical physics. These initial forays into quantum behavior illuminated the path for future research and propelled physics into a new era.
The key characteristic of these discoveries is their ability to introduce concepts that diverge sharply from established norms. These ideas were not merely incremental improvements but rather substantial paradigm shifts. The acknowledgment that matter could exhibit particle-like or wave-like behavior, based on observation, exemplifies the fundamental way early quantum theory reshaped perceptions of reality. The benefit here lies in the development of a framework that resonates well in this article, as it cultivates a deeper understanding of phenomena that otherwise would seem vague or enigmatic.
However, while early discoveries formed the backbone of quantum mechanics, they also had their drawbacks. The abstract nature of these proposals often perplexed contemporaries, leading to a plethora of interpretations that would define the discipline.
Key Figures in Quantum Mechanics
The evolution of quantum mechanics would be incomplete without acknowledging the influential figures who shaped the discourse. Icons such as Albert Einstein and Werner Heisenberg contributed insights that fundamentally altered the landscape of physics. Einstein’s famous equation, E=mc², while often associated with relativity, also holds implications in quantum fields, demonstrating the interconnectedness of energy and matter.
Key figures in quantum mechanics represent the advocacy for questions that challenge the very essence of existence. Their unique approaches aimed at grasping the implications of quantum phenomena, marking significant strides in theoretical physics. Their contributions have sparked debates, intrigued scholars, and driven inquiry into the nature of reality itself.
This inclusion provides a multi-dimensional perspective on quantum physics, transitioning from initial discoveries to crucial theorists, thus enriching the narrative of its historical development. The slight disadvantage, however, might come from the fact that interpretations may diverge, leading readers into complex debates that can sometimes obscure practical takeaways.
Fundamental Concepts
Waves and Particles
Delving into the fundamental concepts, we encounter the duality of waves and particles — a cornerstone of quantum physics. This complex relationship reflects how particles like electrons can exhibit properties of both discrete entities and continuous waves depending on the experimental conditions. The implications of this duality extend beyond theoretical physics; they have practical applications, influencing technologies like semiconductors and quantum sensors.
A defining characteristic of this concept lies in its capacity to capture the essence of quantum behavior, allowing for a nuanced grasp of atomic interactions. Its significance is profound, as it opens avenues to explore phenomena that evade classical explanation. However, such intricacies also introduce ambiguities that can lead to misunderstandings, especially among those new to this subject.
The Dual Nature of Light
The concept of light’s dual nature exemplifies the unexpected behaviors that quantum physics often reveals. Light cannot be confined strictly to being a wave or a particle, as it acts in both capacities based on the circumstances. This aspect has led to groundbreaking research in areas like quantum optics and has paved the way for technologies such as lasers that rely on manipulating light at the quantum level.
Light's duality emphasizes the necessity for a flexible mindset when contemplating quantum phenomena, presenting a unique feature as it challenges traditional views of its behavior. The advantages are clear; grasping this concept is crucial for understanding advanced quantum theories and applications. Yet, at the same time, the complexity of light's behavior can pose learnig hurdles for many, engendering confusion that could impede comprehension.
Principles of Quantum Mechanics
The realm of quantum physics is both mesmerizing and at times confusing. Among its fundamental pillars are the principles of quantum mechanics, which serve as a framework for understanding how particles behave at microscopic scales. The importance of these principles lies in their ability to explain phenomena that are utterly alien to our everyday experiences. From the seemingly bizarre behavior of particles to the implications for future technology, these principles are key to unlocking a deeper grasp of the universe.
Quantum Superposition
Quantum superposition is a cornerstone concept in the study of quantum mechanics. It dictates that a particle can exist in multiple states simultaneously until it is measured. This notion is not just intriguing; it fundamentally alters how we perceive the universe.
Defining Superposition
At its core, defining superposition involves acknowledging that particles can be in multiple states at once. For instance, think of a spinning coin. While it spins, it’s both heads and tails at the same time, only settling on one when it is caught and observed. This characteristic is seen as beneficial in quantum contexts, as it allows for complex computations and possibilities that classical systems could never achieve.
The unique feature of superposition is its role in quantum computing. Quantum bits, or qubits, leverage this to perform calculations at astonishing speed, presenting significant advantages over classical bits, which can only exist in a state of 0 or 1. Yet, one must not overlook its complexities. Measurement collapses the superposition into one definite state, leading to questions surrounding the observer's role in determining quantum outcomes.
Implications for Quantum States
Considering the implications for quantum states, superposition influences how we understand the nature of reality itself. It pushes the boundaries of determinism, suggesting that outcomes are not just probabilistic but dependent on the act of measurement.
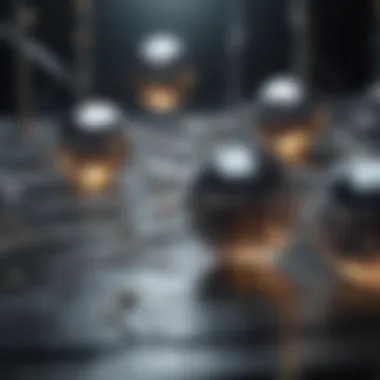
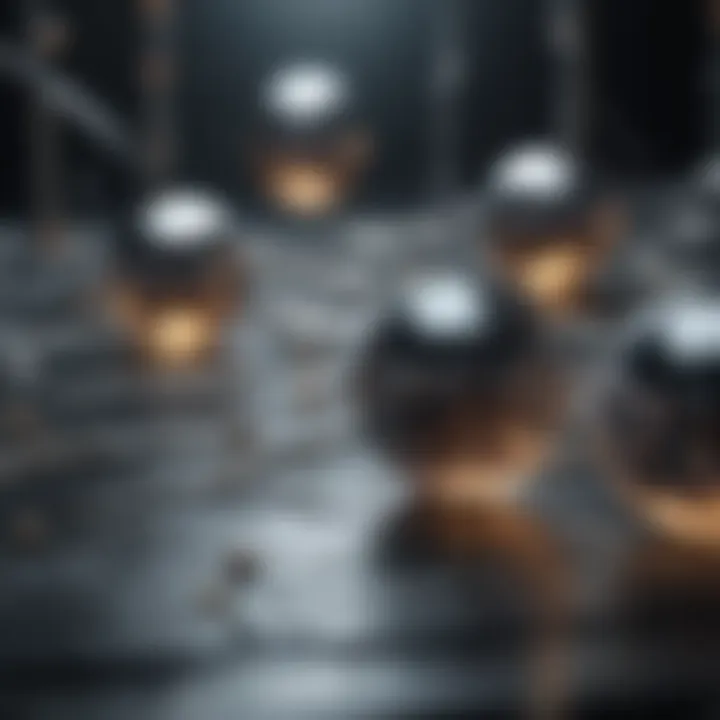
The pivotal characteristic of this principle lies in its unpredictability, creating a landscape where anything could happen. This unpredictability is appealing as it leads to advanced algorithms in quantum computing, but it also raises concerns about the reliability of quantum systems. The interplay between possibility and certainty makes superposition a focal point of ongoing research.
Quantum Entanglement
Entanglement is another key principle of quantum mechanics. It occurs when two particles become connected in such a way that the state of one immediately influences the state of another, regardless of the distance between them. This foreign notion challenges our classical ideas about local realism and causation.
Understanding Entanglement
When discussing understanding entanglement, it is noteworthy how it fosters instantaneous correlations between entangled particles. If one were to measure one particle and observe its state, the second particle would instantly reflect a corresponding state, no matter how far apart they are. This characteristic has made entanglement a popular subject in theoretical and experimental physics.
Its unique aspect lies in its potential to revolutionize communication through quantum teleportation and secure data transfer, which offers immense benefits over classical methods. However, the very nature of entanglement also invites skepticism, leading to debates about its interpretations and the underlying reality of our universe.
The Einstein-Podolsky-Rosen Paradox
The Einstein-Podolsky-Rosen paradox challenges the meaning of entanglement by positing that quantum mechanics is incomplete. Einstein and his contemporaries claimed that if quantum mechanics were true, it would imply “spooky action at a distance,” which stood at odds with prevailing notions of locality.
This paradox's significance is amplified as it sparks discourse on the limits of our understanding. A key characteristic of this paradox is its ability to illustrate the complexities and implications entangled particles present, making it crucial for this article.
When delving into the paradox, one discovers its unique aspect in how it informs philosophical debates on the nature of reality and measurement in quantum mechanics. While it prominently critiques quantum mechanics, it has also led to renewed investigations into the interplay between quantum mechanics and classical physics. This makes it both a challenge and a source of advancement in our understanding of the quantum world.
"Entanglement shows that the properties of particles are not intrinsic, as we think, but depend on their relationship with each other."
In summary, the principles of quantum mechanics not only reveal the intricacies of the quantum world but also have far-reaching implications for our understanding of the universe and the development of new technologies.
Quantum Theories and Models
Quantum theories and models are essential pillars of quantum physics, providing frameworks that help dissect the fundamental behaviors of particles at the quantum level. They offer not only insight into the peculiarities of matter and energy, but they also serve as a bridge between abstract concepts and practical applications. As we delve deeper, it becomes apparent that these theories underpin many revolutionary technologies we encounter today.
The Copenhagen Interpretation
Overview of Copenhagen
The Copenhagen interpretation stands as a cornerstone in the study of quantum physics. Proposed mainly by Niels Bohr and Werner Heisenberg during the early 20th century, it provides a compelling yet somewhat controversial lens through which to view quantum phenomena. This interpretation posits that quantum systems ultimately do not have definite properties until they are observed. The act of measurement plays a crucial role—it defines the state of the particles, collapsing their possible outcomes into a single reality.
A notable characteristic of the Copenhagen interpretation is its emphasis on the wave function. The wave function encapsulates all potential states of a quantum system and evolves according to the Schrödinger equation until it is measured. This understanding has gained traction, if only for its practical utility in making predictions about experiments. However, the interpretation has its share of objections; critics argue that it sidesteps deeper issues of reality and knowledge. Nevertheless, it remains a popular choice for this article because its foundational ideas continue to influence both quantum mechanics and philosophical discourse.
"To be is to be perceived." – George Berkeley, reminiscent of ownership in the Copenhagen interpretation.
Critiques and Alternative Theories
Critique of the Copenhagen interpretation often hinges on its perceived neglect of a more robust ontological framework. Opponents argue that the theory does not satisfactorily address the underlying nature of reality. For instance, David Bohm proposed an alternative known as the de Broglie-Bohm theory. This theory introduces hidden variables that provide a deterministic view, effectively removing the ambiguity that comes from mere observation.
Another significant critique revolves around the idea of complementarity, another foundational element of the Copenhagen interpretation. It suggests that different measurements can reveal complementary information about a quantum system but cannot provide a full picture. While this perspective has its own merits, critics often consider it too vague, lacking a comprehensive explanation. By addressing these critiques, this article achieves a balanced viewpoint, showcasing both the strengths and limitations of the Copenhagen interpretation alongside alternative theories.
Many-Worlds Interpretation
Concept Explanation
The Many-Worlds Interpretation offers a fascinating perspective on quantum mechanics, positing that all possible outcomes of quantum measurements are realized in separate, branching universes. This concept stems from Hugh Everett III's work in 1957 and radically challenges the traditional understanding laid out by the Copenhagen interpretation. One key feature is the insistence that there is no collapse of the wave function; instead, every outcome occurs, just in different realities.
This approach is beneficial as it strives to eliminate randomness from the equation, providing a deterministic framework that aligns with classical physics. The concept's unique feature is its inherent multiverse scenario, which allows for fantastical implications about the nature of existence. The major drawback, however, lies in its philosophical and empirical testability. Critics often argue that the multitude of universes cannot be scientifically verified, leaving the Many-Worlds Interpretation on uncertain ground in terms of acceptance.
Implications for Reality
The implications of the Many-Worlds Interpretation extend far beyond mere academic debate; they force us to confront profound questions about reality itself. If every possible decision branches into a new universe, what does that mean for our understanding of choice and consequence? The key characteristic here is the way it alters our perception of causality—every conceivable action spawns a new world, creating an ever-expanding tapestry of existence.
Much like the Copenhagen interpretation, this theory spurs intellectual curiosity and discussion. Its unique proposition that all possible outcomes exist challenges the fabric of reality as understood through classical mechanics. However, such assertions have not been without contention. Many remain skeptical, questioning whether human perception, which fundamentally shapes our understanding of reality, can ever reconcile with a universe of infinite possibilities.
Overall, both the Copenhagen and Many-Worlds interpretations stand as reputable models in quantum physics, highlighting the rich tapestry of theories that attempt to explain reality's most elusive aspects. By embracing both perspectives while being open to critiques and alternatives, this article engages readers in the philosophical and scientific dance that quantum theories entail.
Quantum Physics and Technology
Quantum physics has become a linchpin of modern technology, influencing various fields, from computing to encryption. The intricate principles that govern quantum systems sit at the heart of innovations that propel industries into the future. Understanding quantum physics isn’t just for physicists; it shapes the technology we use every day.
Quantum Computing
Defining Quantum Computers
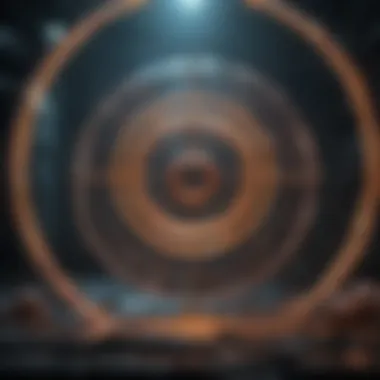
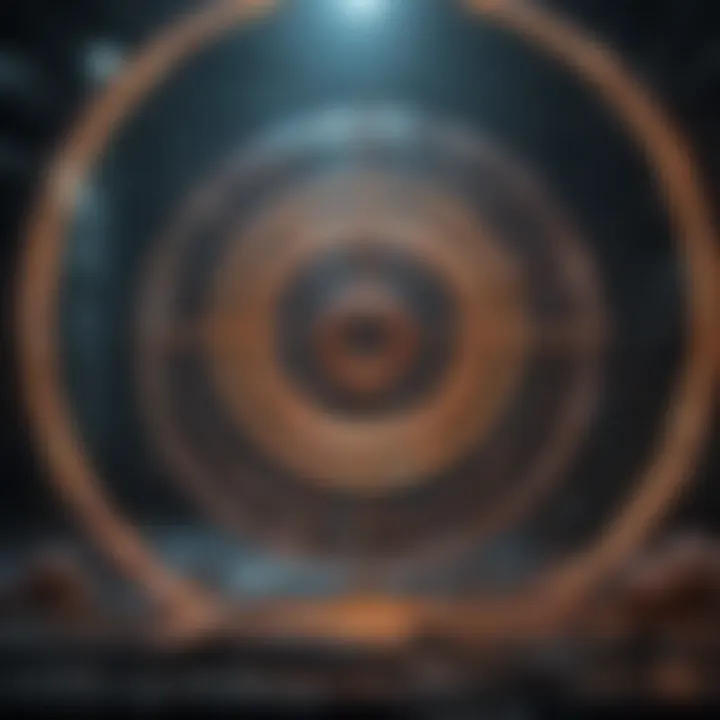
Quantum computers are defined by their core characteristic: the ability to process information using quantum bits, or qubits. Unlike classical bits, which are binary and can be either 0 or 1, qubits can exist in a state of superposition, allowing them to represent both simultaneously. This unique capability means quantum computers can tackle complex problems at incomprehensible speeds compared to their classical counterparts.
One significant contribution of quantum computers lies in their potential for solving problems deemed unsolvable by classical computers, such as factoring large numbers efficiently or simulating molecular interactions accurately. This could revolutionize fields like cryptography and drug discovery.
"In a world of increasing complexity, quantum computing offers a glimmer of hope, a new way to approach problems that have long eluded solutions."
Despite their advantages, quantum computers bring challenges. They are notably error-prone due to decoherence, where the system loses its quantum state. This can affect the reliability of computations and necessitates further research.
Superiority Over Classical Computing
When comparing the superiority of quantum computing to classical computing, it's remarkable how quantum systems can search through vast amounts of data more efficiently. This becomes crucial in fields like artificial intelligence, where large datasets prevail. The key characteristic here is quantum parallelism, which allows quantum computers to evaluate many possibilities at once.
For instance, while a classical computer might search for an element in a list sequentially, a quantum computer can accomplish this exponentially faster using algorithms like Grover’s search algorithm. This fundamentally alters the efficiency scope of computing.
Yet, it is essential to be aware of the hurdles. Quantum computers require extreme operational environments—typically near absolute zero—to function effectively. These requirements can introduce logistical complications and often make maintenance costly. Nonetheless, the potential payoffs in terms of computational power and speed may well justify these inconveniences.
Quantum Cryptography
Basics of Quantum Cryptography
Quantum cryptography revolves around utilizing quantum mechanics to secure communication. One pivotal aspect is the principle of quantum key distribution, which ensures that any attempt at eavesdropping is easily detectable. This characteristic highlights the security benefits of quantum cryptography and reinforces its growing importance in information technology.
The unique feature of quantum cryptography lies in entanglement. When two particles are entangled, a change in the state of one instantly affects the other, regardless of distance. This phenomenon underpins the reliability of quantum cryptographic systems, as intercepting the key will inevitably disturb the quantum state, alerting users to potential security breaches.
However, while the theoretical benefits are clear-cut, practical implementations are still grappling with challenges such as distance limitations and the current immaturity of technology. Nevertheless, its promise of unparalleled security makes it a hot topic in cybersecurity discussions.
Practical Applications
The practical applications of quantum cryptography are expanding rapidly; industries are starting to hedge their bets on this technology. For banks and governmental organizations, it could reshape how sensitive data is transmitted across networks, providing a level of tamper resistance unheard of with classical methods.
One significant characteristic of implementations in this sphere is that they often require terrestrial fiber-optic networks to ensure stable transmission of quantum states. The advantage stands with the possibility to create secure communication channels that can withstand future attacks by quantum computers themselves. This leads to intriguing discussions about the future of data security.
While practical applications are increasing, there still exist barriers. For example, significant costs and the technical complexities of deploying such systems may initially limit adoption. Yet, as technology advances, these obstacles may blur, allowing broader implementation in various sectors.
Real-World Applications
The realm of quantum physics is not merely a theoretical abstraction confined to dusty textbooks and academic lectures. It extends its profound influence into various facets of everyday life, demonstrating its practical significance. Understanding real-world applications of quantum physics unveils a treasure trove of technological advancements and innovations that reshape the way we experience our environment. The interplay of quantum principles with technological development is essential in areas like sensing and materials science.
Quantum Sensors
Quantum sensors have emerged as invaluable tools in various industries. Their unique properties allow them to measure physical quantities with astonishing accuracy. This heightened sensitivity comes from the principles of quantum mechanics.
Types of Quantum Sensors
There are several types of quantum sensors, each tailored to specific measurement challenges.
- Atomic Clocks: These are perhaps the most well-known, relying on the oscillation frequencies of atoms to provide unparalleled precision in timekeeping. By utilizing quantum states, they achieve accuracies that far surpass traditional timepieces.
- Quantum Interferometers: Used for gravitational wave detection, these sensors exploit the interference of quantum states to measure minute changes in space-time.
The key characteristic of quantum sensors is their ability to operate under conditions that leave classical sensors in the dust. For example, the precision of atomic clocks not only supports satellite navigation systems but also enhances standards for international timekeeping.
Advantages Over Traditional Sensors
The advantages of quantum sensors compared to their traditional counterparts are manifold.
- Increased Sensitivity: Quantum sensors can detect very weak signals that conventional sensors would miss completely.
- Enhanced Accuracy: They are capable of providing measurements with uncertainties lower than those achievable by classical methods.
- Miniaturization Potential: Many quantum sensors can be miniaturized, making them suitable for portable applications in various fields.
Unique features such as entanglement and superposition imbue these sensors with a transformative potential. However, their complexity can pose challenges in practical implementation, necessitating specialized knowledge for optimum performance.
Impact on Material Science
Material science benefits immensely from quantum physics. Here, quantum effects play a crucial role in understanding and manipulating material properties at an atomic level.
Quantum Effects in Materials
Quantum effects in materials refer to phenomena that occur due to the discrete nature of energy levels within atoms. This aspect is fundamental to the development of new materials with tailored characteristics.
For instance, phenomena like superconductivity and magnetoresistance can be traced back to quantum behavior. The ability to manipulate these effects leads to the creation of materials with remarkable conductivity, potentially revolutionizing electronics and energy storage.
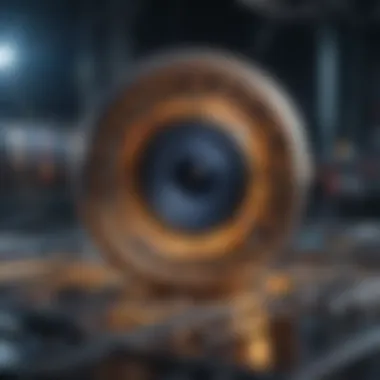
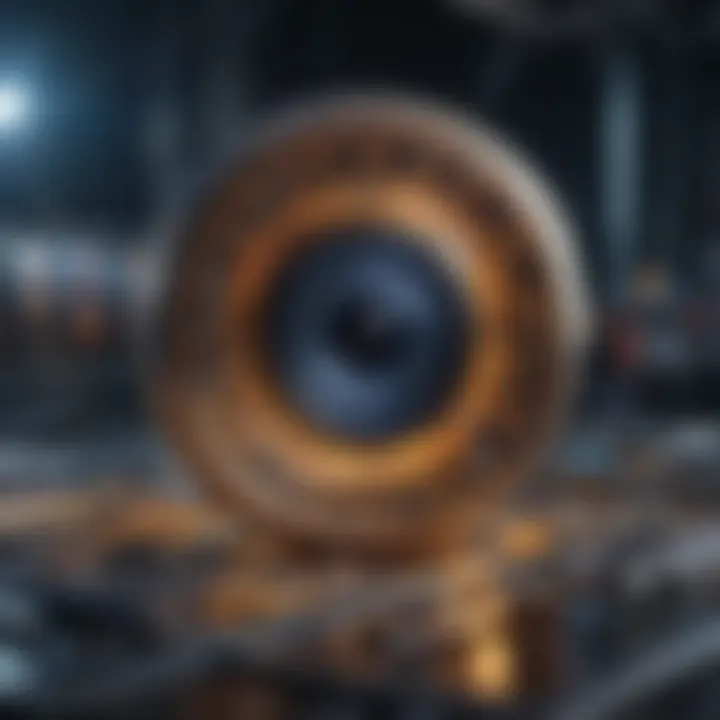
This understanding opens doors for applications in energy-efficient devices, paving the way to substantial energy conservation practices.
Applications in Nanotechnology
The applications in nanotechnology are abundant, driven by the unique properties of materials at the nanoscale. By leveraging quantum principles, researchers can design materials with specific functions.
- Quantum Dots: These are semiconductor particles that exhibit quantum mechanical properties, crucial for applications in photovoltaics and biological imaging due to their tunable optical properties.
- Nanocomposites: By incorporating quantum effects, materials can be designed to enhance strength while remaining lightweight.
Through refined integration of quantum principles, nanotechnology fosters the development of better batteries, supercapacitors, and other electronic devices.
Great potential lies within a better understanding of quantum concepts, even if practical implementation requires ongoing research to overcome technical challenges. The future of quantum applications in material science holds remarkable promise, bridging the gap between theoretical exploration and tangible human benefits.
Philosophical Implications of Quantum Physics
Delving into quantum physics inevitably leads to profound philosophical discussions. The intricate ideas surrounding quantum mechanics not only challenge our understanding of the physical world but also compel us to reconsider what we perceive as reality. Discussions involving the nature of consciousness, the role of the observer, and the fundamental building blocks of existence arise. This section aims to unravel how quantum physics influences modern philosophical thought, casting light on those age-old questions about reality and existence while simultaneously revealing the limitations of our scientific inquiries.
Reality and Observation
The Observer Effect
The observer effect, a cornerstone of quantum mechanics, suggests that the act of observation can fundamentally alter a system's behavior. When measuring a quantum state, such as the position or momentum of a particle, one may inadvertently influence its properties. This phenomenon illustrates the delicate relationship between the observer and the observed, highlighting a critical tension between subjective awareness and objective reality.
A key characteristic of the observer effect is its reliance on the principle of superposition; quantum systems exist in multiple states until they are observed. This aspect presents a significant hurdle for scientists and philosophers alike, as it calls into question the validity of our observations.
The unique features of the observer effect lie in its challenges to traditional notions of causality and determinism. In essence, it brings forth a refreshing, though bewildering, perspective into our understanding of the universe. The discussion around this topic is rich and varied, presenting both advantages and disadvantages in conceptualizing the nature of reality.
Debates on Reality vs. Perception
The debates on reality versus perception revolve around the fundamental question: what is real? Quantum physics, through its unconventional principles, has stirred up a whirlwind of philosophical conversations. The argument centers on whether reality exists independently of observation or if it is intrinsically linked to our perceptions.
One prominent characteristic in this discourse is the acknowledgment that our senses may mislead us, influenced by the limitations of our understanding of quantum phenomena. Consequently, this raises vital questions about the nature of knowledge and understanding. Such discussions are crucial for educators and researchers, as they're confronted with the task of conveying complex ideas about reality shaped by quantum mechanics.
Its unique feature involves raising awareness about the subjective nature of our experiences, inviting skepticism towards the validity of what we observe. The advantages of exploring these debates lie in questioning the assumptions on which we build our scientific knowledge. Yet, there are disadvantages too—navigating this philosophical terrain could lead to an overwhelming sense of uncertainty, challenging established beliefs and theories.
Determinism vs. Indeterminism
Philosophical Perspectives
The tension between determinism and indeterminism remains a hot topic of philosophical inquiry in quantum discussions. Determinism posits that all events, including human actions, are determined by preceding events. Indeterminism challenges this view, suggesting that not all occurrences can be predicted based on prior states due to inherent randomness in quantum mechanics.
A key aspect of philosophical perspectives here is the implication that our understanding of free will may be jeopardized by quantum indeterminacy. By confronting the constraints of human agency, this section compels readers to reflect on the nature of causation and event-horizon.
Moreover, such inquiries provide depth to the philosophy of science. By grappling with these notions, readers can engage in meaningful dialogues about the implications of quantum physics on ethical considerations and the essence of choice itself.
Implications for Scientific Inquiry
Finally, the implications of quantum mechanics on scientific inquiry cannot be overstated. As researchers navigate the blurry lines between known and unknown realms of quantum physics, they must grapple with the evolving understanding of scientific truth.
One key characteristic of this intersection is the impact on methodologies. Traditional empirical methods face challenges as scientists explore phenomena that cannot be observed directly or are influenced by their observation. This realization urges a reconsideration of how we gather data and what constitutes evidence in scientific studies.
The unique feature here is the evolving perspective on what it means to conduct science in a quantum framework. On one hand, it opens up new avenues for inquiry, prompting innovative research designs. On the other hand, it may create frustrations as researchers attempt to reconcile classical and quantum approaches.
"Quantum physics doesn't just tweak the playbook; it rewrites the entire narrative of what we comprehend about nature."
Exploring the philosophical implications of quantum physics enriches our appreciation of reality. It's a complex terrain, filled with questions that may not have clear answers but certainly invite deep reflection and inquiry.
The End
The culmination of this discourse on quantum physics emphasizes its indispensable role in enhancing our comprehension of the universe. The expansive nature of this field stretches beyond mere scientific intrigue; it weaves through the very fabric of modern technology and philosophical thought. A key takeaway is that the principles discussed—quantum superposition, entanglement, and the debates surrounding determinism—are not confined to the theoretical realm but ripple through practical applications, steering advancements in technology and shaping our understanding of reality itself.
Summary of Key Points
In summary, the article elucidated the following pivotal points:
- Quantum Mechanics Basics: Explored fundamental concepts, such as the duality of light and the quantum nature of particles.
- Core Principles: Delved into quantum superposition and entanglement, explaining how these principles challenge classical notions.
- Technological Impact: Discussed advancements in quantum computing and cryptography, highlighting their significance in contemporary applications.
- Real-World Applications: Covered quantum sensors and their advantages, demonstrating the practical benefits derived from quantum physics.
- Philosophical Discussions: Examined the implications of quantum theory on our understanding of reality and determinism.
This succinct summary serves to remind readers of the essential elements that intertwine throughout the fabric of quantum physics, reiterating its complexity yet importance.
Future Directions in Quantum Research
As we peer into the horizon of quantum research, certain trajectories appear promising:
- Quantum Supremacy: The ongoing quest to achieve quantum supremacy will further push the boundaries of processing power, allowing for the solution of problems previously deemed intractable.
- Quantum Networks: The establishment of quantum networks promises secure communication channels that could revolutionize the landscape of data security, making quantum cryptography more widespread.
- Interdisciplinary Research: Synergies between quantum mechanics and fields like biology are gaining traction, with studies focusing on quantum effects in biological processes, which might unveil fascinating insights in the future.