Foundations of Fundamental Physics: Key Theories and Insights
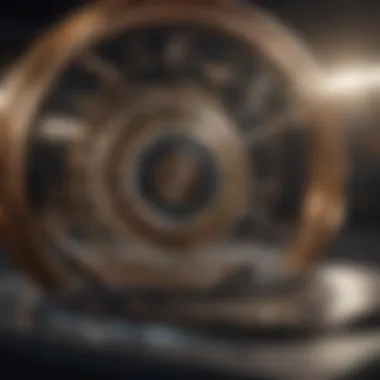
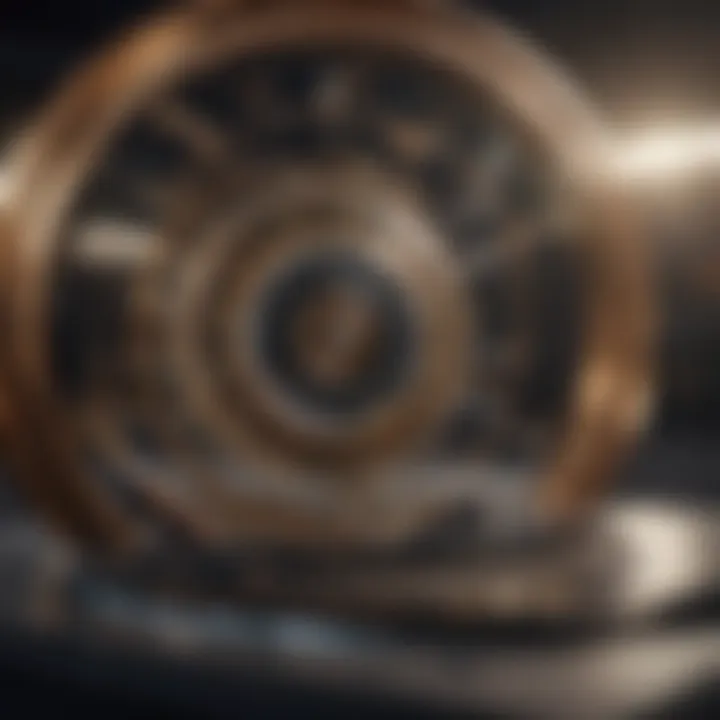
Intro
Physics is the backbone of all scientific explorations. It questions the very fabric of reality, leading us to not just comprehend the universe, but to question our place within it. As humanity progresses, the understanding of foundational principles of physics becomes not just crucial, but imperative. This exploration does not merely skim the surface; it dives deep into the essence of key theories, historical milestones, and their pervasive impacts on our daily existence.
Throughout this article, we will navigate the evolution of fundamental physics, threading historical narratives with modern implications. We will brush against the legends of classical mechanics, appreciate the intricacies of electromagnetism, and consider the subtly complex nature of thermodynamics. Each theory etched a path for progress, influencing everything from the behavior of a falling apple to the flicker of a star in the distant cosmos.
By bridging past discoveries with present understandings, we aim to deepen appreciation for the vast landscape of theoretical frameworks through ongoing research and advanced experimental techniques. Not just theories in textbooks, these ideas breathe life into how we perceive and interact with the world everyday.
"Physics is not a mere subject, itโs a lens through which we unwind the threads of existence."
As we embark on this intellectual journey, it is essential to highlight some groundbreaking research that has reshaped our perspectives in recent years. As we untangle the threads of these findings, we will encounter the simplicity in complexity that defines fundamental physics. Letโs first examine the most pivotal discoveries that have nudged the boundaries of our understanding.
Preface to Fundamental Physics
Understanding fundamental physics is akin to peeling back the layers of an onion, revealing deeper truths about the universe as each layer is removed. This field stands at the very heart of scientific inquiry, offering insights not merely into the laws that govern motion, energy, and matter, but into the very fabric of reality itself.
When we discuss fundamental physics, we refer to the principles that form the backbone of physical science, encompassing interactions on both a macroscopic and microscopic level. These principles shape our understanding of everything from the mundaneโlike how a ball rolls down a hillโto the cosmicโsuch as how galaxies interact within the cosmos. The importance of fundamental physics extends beyond academia; it influences technology, medical devices, and even philosophy, bridging gaps between various fields of study.
Definition and Scope
Fundamental physics investigates the basic interactions that define physical reality. It dissects phenomena into their core components guided by overarching laws. Without getting into the weeds, we can simply define it as:
- Branches: Including but not limited to classical mechanics, electromagnetism, thermodynamics, quantum mechanics, and relativity.
- Goals: Understanding and predicting how physical systems behave under a range of conditions, which can be applied in numerous fields such as engineering, technology, and environmental science.
The scope of fundamental physics is vast. It is foundational in shaping theories and principles that align with both observations and experiments, making it essential for any aspiring scientist or inquisitive mind.
Historical Context
Peering into the history of fundamental physics is like navigating a time-honored journey, dotted with pivotal moments that have reshaped our understanding of the universe. From ancient Greek philosophers who proposed early notions of matter, to Isaac Newtonโs formulation of the laws of motion in the 17th century, this field has evolved dramatically.
The development of electromagnetism in the 19th century showcased the interplay between electricity and magnetism, heralding a new era spearheaded by figures such as James Clerk Maxwell. Fast forward to the 20th century, where Albert Einsteinโs theory of relativity shook the foundations of classical physics, shifting how we think about time and space. Each of these milestones marked a stepping stone that led to the modern understanding we have today.
"The greatest discoveries are those that can be explained simply, yet illuminate the complexities of the universe.โ
Today, as we sift through historical contexts, itโs vital to recognize how each discovery builds on the last, forming a complex mosaic. Fundamental physics continues to evolve, tackling unanswered questions and fostering innovations that push the boundaries of what we deem possible.
Classical Mechanics
Classical mechanics serves as the bedrock of physics, allowing us to comprehend motion and the forces influencing it. This field, rooted in the works of pioneers like Isaac Newton, lays down the fundamental principles that govern everything from the paths of planets to the trajectory of a thrown ball. Its significance transcends mere academic interest; classical mechanics is essential for engineers, scientists, and anyone aiming to understand how the physical world operates.
Newton's Laws of Motion
Newton's three laws of motion capture the essence of classical mechanics, explaining how objects behave under various forces. The first law, often dubbed the law of inertia, states that an object at rest stays at rest, and an object in motion stays in motion unless acted upon by an external force. This simple yet profound observation sets the stage for exploring dynamics and establishing foundational concepts.
The second law, which introduces the famous formula F = ma (Force equals mass times acceleration), provides a quantitative framework for predicting how an object will respond to applied forces. The third law, asserting that for every action, there is an equal and opposite reaction, emphasizes the interconnectedness of forces.
These laws are not just theoretical; they are practically visible in everyday life, such as when a car accelerates or stops, illustrating how essential Newton's principles are for modern physics.
Conservation Laws
In addition to Newton's laws, conservation laws play a vital role in classical mechanics. These laws state that certain physical quantities remain constant in an isolated system. For instance, the law of conservation of energy asserts that energy can neither be created nor destroyed; it only changes form. This principle is fundamental in analyzing systems ranging from roller coasters to planetary motion.
Another key conservation law is the conservation of momentum, which maintains that the total momentum of a closed system remains constant in the absence of external forces. This becomes particularly relevant in collisions and interactions between particles.
Understanding these conservation laws provides powerful tools for problem-solving, often simplifying complex scenarios into manageable calculations. They reveal the underlying order in what might initially seem like chaotic systems.
Applications to Real-World Problems
The applications of classical mechanics are numerous, penetrating various spheres. Engineers leverage these principles to design vehicles, ensuring they can withstand forces during acceleration and deceleration. Architects apply knowledge of forces to create stable structures that can endure environmental stresses.
Furthermore, classical mechanics plays a crucial role in fields like sports science, where understanding the mechanics of movement can enhance performance. From calculating the optimal angle for a long jump to modeling the forces acting on a soccer ball in flight, the principles derived from classical mechanics inform practical applications across disciplines.
Some notable real-world scenarios where classical mechanics shines include:
- Aviation: Engineers use Newton's laws to calculate takeoff speeds and flight paths.
- Astrophysics: Understanding the orbits of celestial bodies relies heavily on classical principles.
- Automotive Engineering: Vehicle safety tests assess how cars behave under collision, based on momentum conservation.
The insights provided by classical mechanics empower not just scientists but everyone to make sense of the physical phenomena that govern daily experiences, from simple motions to complex interactions.
In summary, classical mechanics is a key element of fundamental physics. It encapsulates crucial laws that explain motion and forces, provides a rigorous framework through conservation laws, and is instrumental in tackling real-world challenges. Understanding these principles is vital for anyone stepping into the fields of science or engineering.
Electromagnetism
Electromagnetism stands as a pillar in the edifice of fundamental physics, intricately woven into the very fabric of our modern understanding of the universe. It plays a crucial role in myriad phenomena, shaping not just the realms of physics but also impacting technology, communication, and even our daily lives. When we discuss electromagnetism, weโre essentially talking about the interrelationship between electricity and magnetismโtwo forces that are distinct yet inseparably linked.
This topic is essential for a number of reasons:
- Foundational Role: Understanding electromagnetism is essential for comprehending other fields in physics, including optics, electronics, and even thermodynamics. The principles laid down by this branch of physics serve as a springboard for further exploration into complex concepts.
- Technological Impact: The influence of electromagnetism extends beyond theoretical implications. From the simplest battery-operated devices to the most intricate communication systems, it is the foundation of modern technology. Devices such as smartphones, MRI machines, and electric motors owe their functionality to the principles of electromagnetism.
- Everyday Relevance: The effects of electric and magnetic forces are observable in everyday life, influencing everything from how we interact with electronic devices to how natural phenomena such as lightning occur.
Maxwell's Equations
At the heart of electromagnetism lie Maxwell's equations. These four fundamental equations, devised by James Clerk Maxwell in the 19th century, form the cornerstone for understanding electric and magnetic fields.
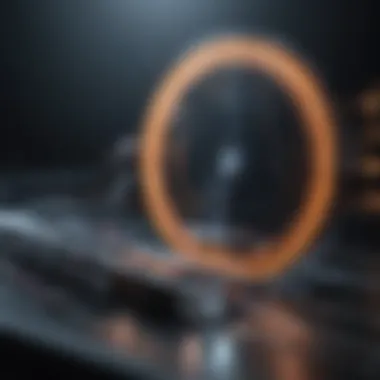
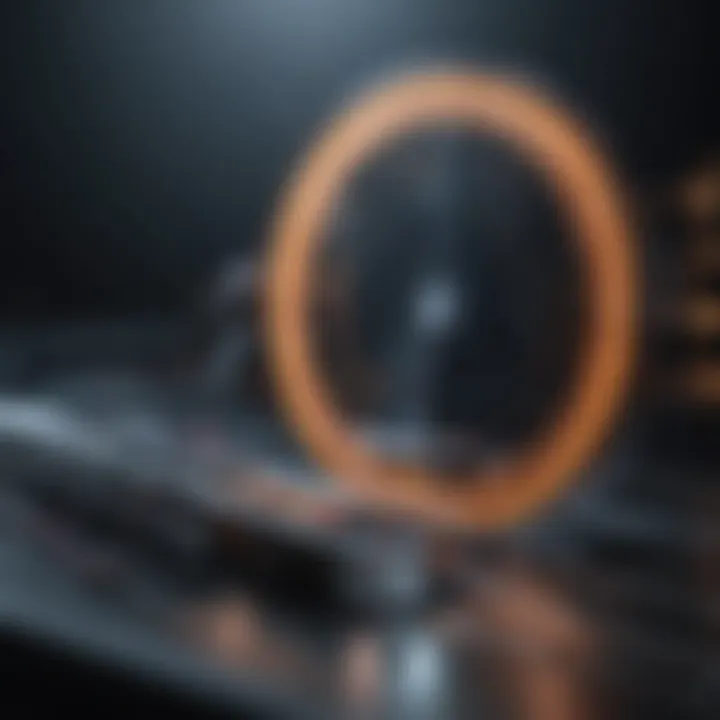
- Gauss's Law: This describes how electric charges produce electric fields.
- Gauss's Law for Magnetism: It highlights that magnetic monopoles do not exist and establishes that magnetic field lines are continuous loops.
- Faraday's Law of Induction: This law explains how a changing magnetic field creates an electric field. This principle is behind the operation of generators and transformers.
- Ampรจre's Law with Maxwell's Addition: This integrates the displacement current in electromagnetic theory, bridging the gap between electric currents and magnetic fields.
Together, these equations not only unify electricity and magnetism but also laid the groundwork for modern physics and technology. They hold vast implications, affecting everything from the behavior of electromagnetic waves to the operation of everyday electronic devices.
Electric and Magnetic Fields
Electric and magnetic fields are the manifestations of the fundamental forces at play. Electric fields are generated around charged particles, exerting forces on other charges within their vicinity. Magnetic fields, on the other hand, are produced by moving electric charges and can affect other charges and magnetic materials.
- Electric Fields:
- Magnetic Fields:
- Created by charged particles (positive or negative).
- Extend outward from positive charges and inward toward negative charges.
- Produced by the movement of electric charges.
- Can be visualized as loops or lines of force emanating from magnetic materials.
"The whole world of modern electronics is built upon manipulating these electric and magnetic fields."
Understanding these fields is essential for grasping how electricity flows, how magnets work, and how both interact to create a myriad of technological applications. Furthermore, the concepts are crucial in more advanced topics such as electromagnetism's role in quantum mechanics.
Optics and Photons
Optics, the study of light, interrelates beautifully with electromagnetism. Light itself is an electromagnetic wave, demonstrating characteristics of both electric and magnetic fields, oscillating perpendicularly to each other. This duality is crucial for understanding phenomena such as refraction, reflection, and diffraction.
Photons, the elementary particles of light, illustrate the interplay between electromagnetism and quantum mechanics. Key points include:
- Nature of Light: Light exhibits both wave-like and particle-like properties, leading to the wave-particle duality that underpins modern physics.
- Applications of Photonics: From fiber optics to lasers, understanding light's behavior underlies much of our advanced technology today.
- Color and Wavelength: The variation of light in terms of color relates directly to the frequency of electromagnetic waves, connecting visual perception to the physical principles of electromagnetism.
In summary, electromagnetism is crucial not only in theoretical physics but also in practical applications that affect our everyday lives. Recognizing the significance of Maxwell's equations, the properties of electric and magnetic fields, and the nature of light helps illuminate the underpinnings of technology and the universe.
Thermodynamics
Thermodynamics is a pillar of fundamental physics, weaving through various aspects of science and engineering as it explains how energy, heat, and matter interact. It holds a unique place not just in theoretical contexts but in real-world applications, making it essential for understanding both the natural world and the technological innovations that emerge from it. This branch delves into the principles that govern energy transitions, providing a lens through which to investigate everything from simple machines to complex biological systems.
Laws of Thermodynamics
At the heart of thermodynamics lie its four primary laws, each presenting a fundamental truth that governs physical processes. These laws establish a framework that describes how energy is conserved and transformed.
- Zeroth Law: This law introduces the concept of temperature, stating that if two systems are each in thermal equilibrium with a third, they are in equilibrium with each other as well. Itโs often overlooked but it underpins the very idea of temperature.
- First Law: This law embodies the conservation of energy principle, stating that energy cannot be created or destroyed, only transformed. For instance, when a car engine burns gasoline, chemical energy is converted into mechanical energy, illustrating this law in action.
- Second Law: Often associated with the directionality of processes, this law indicates that entropy, or disorder, always increases in a closed system over time. It's why a hot cup of coffee eventually cools down in an uninsulated environment.
- Third Law: This law states that as the temperature of a system approaches absolute zero, the entropy approaches a constant minimum. While reaching absolute zero is impossible, this concept hints at the behavior of systems at extremely low temperatures.
Understanding these laws is crucial for anyone exploring energy systems and efficiencies in various applications.
Heat and Work Interaction
Heat and work are the two pathways through which energy is transferred, each playing a unique role in thermodynamic systems. Heat transfer occurs due to a temperature difference, while work is associated with the force applied to displace an object.
- Heat Transfer: It can happen through conduction, convection, or radiation. Each method has its own implications and efficiencies. For instance, conduction occurs in solids where particle vibrations transfer energy, while convection is crucial in fluids where movements cause energy transfer.
- Work: This involves physical quantities moving against a force. A classic example is a gas expanding in a piston, pushing against external atmosphere, converting internal energy to work.
These interactions are not isolated; they are intertwined, often complementing each other in systems that convert thermal energy into mechanical energy, like steam engines or refrigeration units. The study of how heat and work coexist provides invaluable insights into optimizing energy systems.
Entropy and Its Implications
Entropy is a key concept within thermodynamics, often interpreted as a measure of disorder or randomness in a system. However, beyond this definition lies a deep well of implications that touch everything from the fate of the universe to everyday phenomena.
- Understanding Entropy: As stated by the second law of thermodynamics, in an isolated system, entropy can only increase. This means that systems tend naturally to evolve towards thermodynamic equilibrium, where no net energy transfers occur.
- Real-World Applications: In practical contexts, the principle of increasing entropy impacts many areas. For coffee enthusiasts, it explains why your hot brew eventually cools. In engineering, it forces the development of energy-efficient systems, where minimizing entropy production is fundamental to enhancing performance.
- Implications for Cosmology: On a grand scale, entropy raises questions about the eventual fate of the universe. If entropy continues to rise, the universe might trend toward a state of maximum entropy, often referred to as โheat deathโ. Understanding these implications bridges thermodynamics with cosmological theories, making it a truly interdisciplinary field.
"In the grand tapestry of physics, thermodynamics threads through the fabric of reality, illustrating how energy and complexity coalesce and govern the observable universe."
Thermodynamics, with its laws, interactions, and implications, serves as a foundational concept not just within physics but also in the broader scientific landscape. Ignoring this vital discipline would mean forgoing a comprehensive grasp of how the universe operates.
Modern Physics
Modern Physics represents a significant leap from classical approaches, fundamentally reshaping our understanding of the universe. As we explore this territory, it becomes evident that theories like relativity and quantum mechanics are not just esoteric ideas but cornerstone frameworks that inform everything from technology to our grasp of the cosmos. In this section, we will focus on the pivotal elements within Modern Physics that merit closer examination, such as its implications on how we perceive time and space, as well as the underlying principles guiding atomic interactions.
Theory of Relativity
Albert Einstein's Theory of Relativity, comprised of both Special and General Relativity, has left an indelible mark on physics. At its core, Special Relativity revolutionizes our understanding of space and time by positing that they are interwoven and dependent on the observer's velocity. This means that time can dilate; a phenomenon witnessed in the twin paradox where one twin ages slower than the other if they travel close to the speed of light.
General Relativity takes this a step further, suggesting that massive bodies like planets and stars warp the space-time fabric around them. This has profound implications for the movement of celestial objects and the very structure of the universe. For instance, the bending of light around massive objects leads to the phenomenon known as gravitational lensing. This concept is not merely theoretical; it has practical implications, influencing GPS technologies and our understanding of cosmic events.
"Relativity does not define the time or space; it describes how observers experience the universe differently based on their relative motion and gravitational presence."
Quantum Mechanics
Quantum Mechanics introduces a layer of complexity that takes physics into the microscopic realm, where particles exist in states of probability rather than certainty. Concepts like wave-particle duality and uncertainty principles challenge our intuitive perceptions of reality. For instance, the famous double-slit experiment exhibits how particles behave as both waves and individual particles, depending on observation, showcasing the peculiar nature of quantum states.
One of the key benefits of understanding quantum mechanics lies in its applications. Technologies such as semiconductors, lasers, and even the emerging field of quantum computing all hinge on principles derived from quantum mechanics. Moreover, this branch of physics opens our minds to unconventional ideas, including quantum entanglement, where particles remain interconnected regardless of the distance separating them, calling into question our traditional notions of locality and information transmission.
Particle Physics and the Standard Model
The realm of particle physics dives deeper into the components of matter, structured through the Standard Model. This model elucidates the fundamental particles that make up the universe, such as quarks and leptons, and describes the forces governing their interactions through bosons. The most famous instance is the Higgs boson, often referred to as the "God particle," discovered at CERNโs Large Hadron Collider in 2012, which provided answers to how particles acquire mass.
However, while the Standard Model has been remarkably successful, it does not encompass everything. It fails to integrate gravity and does not account for dark matter and dark energy, which make up most of the universe's mass-energy content. The ongoing quest in particle physics seeks to explore these gaps, indicating that our knowledge may still be in its infancy.
In summation, Modern Physics stands as a critical field that has transformed our worldview. Its theories encourage a blend of imagination and empirical rigor, driving forward the boundaries of human inquiry.
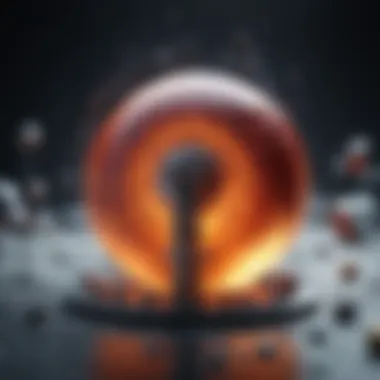
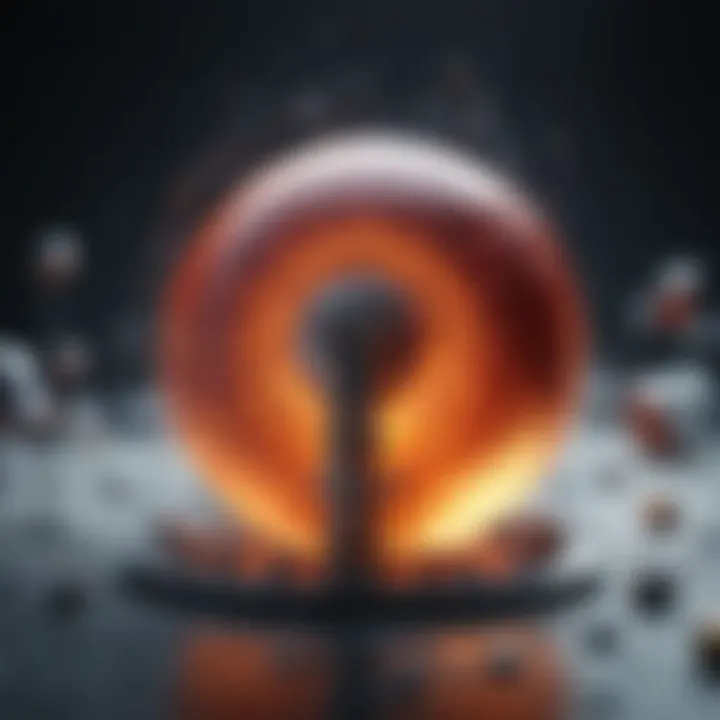
Experimental Methods in Physics
Experimental methods in physics serve as the bridge between theoretical postulations and empirical realities. They allow scientists to test hypotheses, validate predictions, and garner new insights into the workings of the universe. Thatโs crucial, as theory needs to be substantiated by data, or it risks being elegant speculation rather than a robust scaffolding of knowledge. With the rapid advancement of technology, the methods by which we explore these fundamental principles evolve, leading to richer data and a deeper understanding of nature.
Historical Experiments
The history of physics is laced with landmark experiments that fundamentally reshaped our understanding of the physical world. Take Galileo's rolling balls experiment, for instance. He rolled balls down inclined planes, challenging the prevailing notions of gravity. His meticulous measurements not only established the principle of inertia but also paved the way for Newton's laws.
Another notable experiment is the Michelson-Morley experiment in 1887, which sought to detect the luminiferous aether, a substance once thought to permeate space. Despite failing to detect it, the results played a crucial role in the development of Einstein's theory of relativity, illustrating how experiment can sometimes lead to revolutions in thought rather than confirmations.
"Some of the most significant advances in science come from the unexpected outcomes of experiments."
Furthermore, the double-slit experiment greatly highlighted the wave-particle duality of light and matter. By directing electrons or photons through two slits, an interference pattern emerges, hinting at the duality of their nature. These historical experiments lay the groundwork for subsequent explorations and underscore the importance of an empirical approach in the pursuit of knowledge.
Advancements in Technology
In modern physics, advancements in technology have magnified our capacity to conduct precise and varied experiments. Innovations such as particle accelerators and advanced imaging techniques have opened up new vistas in the exploration of matter. The Large Hadron Collider, located near Geneva, is an epitome of technological progress, enabling physicists to probe the fundamental constituents of matter by smashing protons at unprecedented speeds.
Moreover, developments in space-based observatories, like the Hubble Space Telescope, have revolutionized our understanding of astrophysics. These tools allow for the observation of distant celestial bodies, facilitating the study of phenomena like black holes and dark energy. Moreover, advancements in computational power now allow simulations to complement empirical methods, enabling researchers to experiment virtually in ways that were unfathomable a few decades ago. With machine learning and artificial intelligence integrating into data analysis, the pace of discovery accelerates further.
Current Experimental Techniques
Currently, experimental techniques in physics vary drastically depending on the area of study. For example, in condensed matter physics, techniques like neutron scattering and X-ray diffraction allow scientists to understand material properties at the atomic scale. Such methods provide invaluable insights into the behavior of materials, guiding the design of new technologies, from superconductors to nanomaterials.
In particle physics, techniques such as quantum chromodynamics (QCD) allow researchers to delve into the interactions between quarks and gluons. Advanced detectors collect data from high-energy collisions, revealing details about fundamental particles and forces.
Furthermore, cosmological experiments like the Cosmic Microwave Background radiation measurements enable insights into the early universe. Using satellite-based detectors, scientists analyze the afterglow of the Big Bang, unraveling intricacies about the universeโs formation and structure.
These techniques continually adapt, reflecting the changing landscape of physics. They allow us to endure through the complexities of nature, laying the groundwork for profound discoveries that redefine our grasp of the universe.
Theoretical Frameworks
The realm of fundamental physics is a tapestry woven with intricate theoretical frameworks that serve to explain the physical phenomena around us. These frameworks provide a structured approach to understanding how the universe operates, enabling physicists to make predictions and investigate the underlying principles that govern matter and energy. By grasping these foundational ideas, readers can appreciate the robust nature of scientific inquiry.
Modeling Physical Reality
At the heart of theoretical frameworks lies the ability to model physical reality. This involves creating mathematical and conceptual representations of physical systems, which allows researchers to simulate outcomes under various scenarios. For instance, when considering celestial mechanics, mathematicians and physicists employ models to predict the orbits of planets and the dynamics of galaxies. The Kepler laws, which describe the motion of planets around the sun, serve as a classic example of how modeling can yield insights into celestial dynamics.
Modeling also extends to fields like fluid dynamics or quantum physics, where the behavior of fluids or particles is encapsulated in equations. Just as a painter uses a palette to represent reality, physics utilizes models to provide clarity about complex systems. The benefit here is profound; accurate models lead not only to reliable predictions but also to advancements in technology and understanding.
"Mathematics is the language in which God has written the universe." โ Galileo Galilei
This quote captures the essence of modeling in physics โ it reveals how mathematical frameworks can uncover truths about the natural world.
Unification of Forces
The unification of forces represents a pinnacle in theoretical physics, symbolizing the quest to consolidate our understanding of the fundamental interactions that govern the universe. Historically, four fundamental forces have been recognized: gravitational, electromagnetic, strong nuclear, and weak nuclear forces. Each of these forces operates under its own set of principles; however, researchers strive to find a harmonious framework that binds them together.
Consider the groundbreaking work of physicists like James Clerk Maxwell, who unified electricity and magnetism into a singular theory of electromagnetism. This work laid a cornerstone for future endeavors aimed at unifying the remaining forces. More recently, candidates such as string theory and loop quantum gravity have emerged in the pursuit to reconcile general relativity with quantum mechanics.
The implications of achieving a unified theory are monumental. Not only might such a theory enhance our comprehension of existing physical laws, but it could also pave the way for new technologies and methodologies. Moreover, a unified framework would afford physicists the tools necessary to explore phenomena currently shrouded in mystery, such as dark matter and dark energy.
As science continues to advance, the unification of forces remains a central challenge, representing both the beauty and the complexity of fundamental physics.
In summary, theoretical frameworks are the backbone of our exploration of fundamental physics. They facilitate the modeling of our physical reality, allowing for precise predictions and the deepening of our understanding of universal laws. Equally essential is the pursuit of unifying these frameworks to unveil the connections between the fundamental forces, illustrating the intertwined nature of all physical phenomena.
Applications of Fundamental Physics
Fundamental physics lays the groundwork for many aspects of modern life. Its principles are not just confined to academic circles; they permeate technology, inform our understanding of the cosmos, and influence societal progress. Recognizing the importance of these applications helps us appreciate how deeply interconnected physics is with our day-to-day experiences and progress as a society.
Technology Development
The realm of technology is a testament to the applications of fundamental physics. Take, for instance, the realm of communication. Electromagnetic theory, rooted in Maxwell's equations, underpins the functioning of wireless communication devices. This isn't just an abstract concept; itโs the backbone of everyday tools like smartphones and Wi-Fi routers.
Consider also the advancements in medical imaging technology. The principles of quantum mechanics have led to innovations such as MRI and CT scans, allowing for non-invasive exploration of the human body. These advancements can be life-saving, providing healthcare professionals with tools that enhance diagnosis and treatment options.
- Key Areas Impacted by Physics in Technology:
- Communication technology
- Medical imaging
- Energy production and distribution
In energy sectors, the application of thermodynamics and fluid dynamics enables us to design better engines and renewable energy systems. Companies are increasingly turning to solar and wind technologies, guided by the principles of fundamental physics, to create efficient systems that harness natural phenomena.
Insights into Cosmology
When venturing into the cosmos, the relevance of fundamental physics becomes vastly pronounced. Cosmology, the study of the universe's origin and evolution, relies heavily on physics principles to make sense of our surroundings. The notion of spacetime introduced by Einstein is pivotal in understanding phenomena such as black holes and the expansion of the universe.
- Impirical Findings in Cosmology:
- Cosmic Microwave Background Radiation
- Hubbleโs Law and the expanding universe
- Dark energy and the fate of the universe
Through observational data, physicists are piecing together a broader picture of the universe. The data suggests that a large part of the universe is composed of dark matter and energy, which are still not fully understood. Ongoing research could potentially lead to groundbreaking discoveries, guiding us deeper into the mysteries of existence.
Societal Impacts
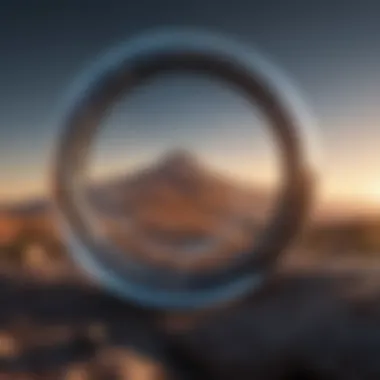
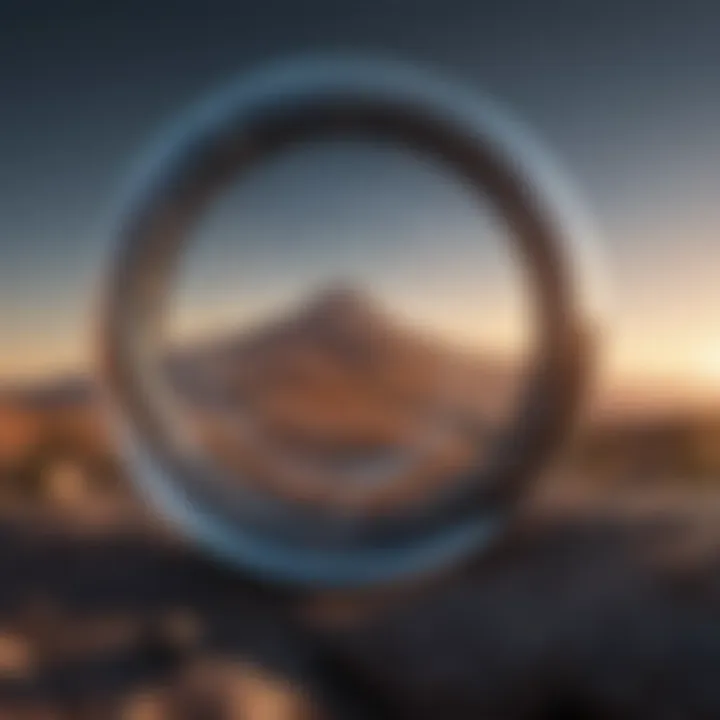
The effects of fundamental physics stretch far beyond laboratories and classrooms. The technologies developed through these principles can improve living standards, raise awareness about climate change, and enhance global communication.
The methodologies derived from fundamental physics also play a crucial role in education and public policy. Understanding how physical principles govern phenomena can inform decision-making, especially regarding environmental policies and technology regulations.
- Societal Benefits of Physics Applications:
- Improved public health through technology
- Informed policy decisions based on scientific evidence
- Greater awareness of environmental issues
To quote a well-known physicist: > "Physics isnโt just about equations; itโs about understanding our universe and the role of humanity within it."
This synthesis of knowledge and technology ultimately enhances the quality of life. It connects people at scales once thought impossible and cultivates a culture that values scientific inquiry and technological innovation. Such applications are vital today, reminding us that at the heart of every advancement lies an understanding of fundamental physics.
Challenges in Fundamental Physics
The landscape of fundamental physics is marked by complexities that present numerous challenges to scientists and researchers alike. As we delve into this multifaceted field, the ability to confront these challenges not only expands our theoretical understanding but forges paths toward groundbreaking discoveries. Tackling issues ranging from unresolved phenomena to the elusive nature of dark matter and energy demands an evolving arsenal of experimental techniques and theoretical frameworks. This section aims to outline the significance of these challenges, the intricate considerations they entail, and the potential benefits that may arise from successful navigation through this intricate web.
Unresolved Phenomena
When we discuss unresolved phenomena in fundamental physics, we're looking at mysteries that have baffled researchers for decades. Take, for instance, gravity. While we can describe its effects with precision, the fundamental nature of gravity remains perplexing. Why does it act in such a different manner than other forces? This query continues to spark debates in the scientific community, with various theories arising โ from string theoryโs extra dimensions to loop quantum gravity.
The implications of these unresolved phenomena are profound. On one hand, they illustrate the limits of our current understanding. On the other, they inspire theories and hypotheses that push the boundaries of what we know. As researchers grapple with these enigmatic aspects, they also encourage interdisciplinary collaboration, bringing together experts from various scientific fields. Such collaborations often lead to innovative experimental designs that can yield new insights.
"In every challenge lies a opportunity for knowledge and understanding."
The Role of Dark Matter and Energy
Dark matter and dark energy are perhaps the most intriguing challenges in modern physics, representing nearly 95% of the universe yet remaining largely invisible and enigmatic. These components are crucial for our understanding of cosmic evolution and structure formation, yet their properties are not well-defined. Numerous experiments and observations suggest their existence, such as the behavior of galaxies and the cosmic microwave background radiation, but the nature of dark matter and dark energy is still a puzzle wrapped in mystery.
Researchers are probing the depths of these dark entities through a variety of experimental avenues. Projects like the Large Hadron Collider are designed to search for signs of dark matter through particle collisions, while observational studies involving telescopes seek to understand dark energy's role in the universe's accelerated expansion. Despite these efforts, many questions linger:
- What exactly is dark matter made of?
- Why is dark energy causing the universe to expand at an accelerating rate?
The endeavor to address dark matter and energy not only challenges our comprehension of physics but also poses philosophical questions about our existence and the universe itself. Understanding these phenomena could be the key to unlocking answers to fundamental questions about the nature of reality.
The Future of Fundamental Physics
The trajectory of fundamental physics is akin to an arrow being launched towards yet unseen realms of understanding, where the potential to unlock nature's deepest secrets shines brightly on the horizon. The future of fundamental physics matters because it directly influences not only our grasp of the universe but also how we apply that knowledge โ from technologies that shape our daily lives to the philosophical implications of existence itself.
As we stand on the brink of new discoveries, there are several compelling elements to consider:
- Advancements in Theoretical Models: New theories, such as string theory and loop quantum gravity, promise to bridge the gap between general relativity and quantum mechanics, tackling issues that have perplexed physicists for decades.
- Unraveling Mysteries of Dark Energy and Dark Matter: Current models suggest that these components comprise around 95% of the universe's total mass-energy content yet remain elusive. Further inquiry may redefine our understanding of fundamental forces and structure of the universe.
- Technological Innovations: Advancements in experimental physics, such as the increased sensitivity of gravitational wave detectors and upgrades to particle accelerators like the Large Hadron Collider, play a crucial role in testing theoretical predictions and possibly leading to ground-breaking findings.
As we plunge deeper into this future landscape, the implications stretch beyond just scientific curiosity. They hold the potential to impact global technologies, energy solutions, and even our navigations through ethical dilemmas posed by artificial intelligence and advanced materials.
Emerging Theories
Emerging theories in fundamental physics often challenge established norms and push the envelope of human understanding. The beauty of theoretical physics resides in its ability to innovate conceptually โ leading to frameworks that might eventually reveal unobserved aspects of the universe.
Take for instance,
- String Theory: This proposes that point-like particles are actually one-dimensional strings vibrating at specific frequencies. It seems abstract, yet many believe it may offer a comprehensive framework to describe all known forces and particles.
- Quantum Loop Gravity: An alternative, this theory attempts to quantize spacetime itself, suggesting that space may consist of discrete units. The implications of this could shift our perception of reality as fundamentally different from how we perceive it now.
The exploration of these new theories is often fraught with challenges, mostly because they require rigorous mathematical models and predictions that can be empirically tested. Yet, these challenges only add to the richness of pursuing theoretical advancements that could transform how we understand physical phenomena.
Interdisciplinary Collaborations
Looking towards the horizon, collaborative efforts across disciplines signal a promising future for physics. The interplay between physics and fields like mathematics, computer science, and biology has been gaining traction.
- Mathematical Tools: Cutting-edge mathematical theories help physicists model complex systems ranging from cosmic scales with the help of topology to quantum behaviors through applied statistics.
- Computational Physics: The realm of big data and machine learning enhances our ability to simulate physical models and analyze experimental results, yielding insights that would be impractical through traditional means alone.
- Biophysics: Understanding biological systems through physical principles could lead to innovations in medical technology, health monitoring, and synthetic biology.
Moreover, such collaborations often lead to unexpected results and breakthroughs that might not have been achievable within the insulated confines of a single discipline.
"To explore the unknown, we must dare to venture beyond the borders of traditional scientific disciplines, pooling our knowledge and creativity together."
In summary, as we look towards the future of fundamental physics, it becomes evident that the confluence of emerging theories along with interdisciplinary collaboration holds the promise for discoveries that could redefine our understanding of both the universe and our place within it.
End
The essence of this concluding section lies in its ability to synthesize the extensive terrain covered in the preceding chapters and to underline why the insights gleamed from fundamental physics are not just academic exercises but rather critical components of our understanding of the universe.
Summary of Key Insights
Throughout the article, significant elements that form the backbone of fundamental physics have been meticulously dissected. Key insights include the following:
- Historical Context: The evolution of physics from classical to modern theories showcases how foundational principles have informed contemporary understanding.
- Interconnection of Theories: The relationship between classical mechanics, electromagnetism, and thermodynamics illustrates a layered architecture of knowledge, where each discipline informs others, creating a comprehensive framework for problem-solving.
- Impact of Experimental Methods: Technological advancements in experimental methods underscore the empirical nature of physics, reminding us that theories must constantly stand the test of real-world scrutiny.
- Challenges and Future Directions: Unresolved phenomena, like dark matter and dark energy, highlight the ongoing challenges physicists face. They compel continuous exploration, indicating that our journey into understanding is far from over.
These insights position fundamental physics as a dynamic field, continually adapting and expanding as new discoveries emerge.
The Ongoing Quest for Knowledge
The pursuit to comprehend the fundamental laws governing our universe is a never-ending journey. Scientists are often faced with questions that stretch the limits of our understanding. To illustrate:
- Emerging Theories: Researchers actively investigate various emerging theories, such as string theory and loop quantum gravity, which propose potential frameworks for unifying the forces of nature. These quests challenge traditional perspectives and open avenues for new realms of thought.
- Collaborative Endeavors: Interdisciplinary collaborations are becoming increasingly vital. Physicists join forces with chemists, biologists, and even computer scientists to glean insights from differing viewpoints. This cross-pollination of ideas not only enriches the foundational aspects of physics but also fosters innovative applications across fields.
As we look to the future, it is evident that fundamental physics remains a pivotal cornerstone of scientific inquiry. It invites both seasoned experts and curious minds alike to join in the exploration, acknowledging that our understanding may be mere stepping stones to even greater revelations.
"The most beautiful thing we can experience is the mysterious. It is the source of all true art and science."
โ Albert Einstein
This call to embrace the unknown encourages an ongoing quest for knowledge that resonates deeply within the heart of physics, proving that in every question lies the potential for discovery.